the Creative Commons Attribution 4.0 License.
the Creative Commons Attribution 4.0 License.
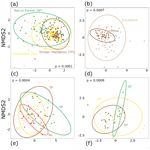
Back to the future? Conservative grassland management can preserve soil health in the changing landscapes of Uruguay
Leonardo R. Ramírez
Sarah Tietjen
Marcos Barra
Erick Zagal
The “soils of the Anthropocene” are predominately agricultural. To understand them, we analyzed agri- and silvicultural intensification of Uruguayan grasslands (GLs) in a country-wide survey on fertility proxies, pH and trace metals in topsoils originating from different land uses across the whole country. Thus, our results reflect interactions of both the natural diversity of Uruguayan soil formation and the impacts of land use change. We observed a loss of nutrients, trace metals and organic matter from GLs, croplands and timber plantations (TPs). As an example, the cation exchange capacity was 160 % higher in native forests (NFs) compared to GLs and lowest in TPs, reaching only half of the cation exchange capacity (CEC) in GLs. Acidification of topsoils continues as three-fourths of all samples are “extremely acidic” and “very strongly acidic”. Topsoils of riverine forests accumulate more trace metals compared to the other uses. We assume an accumulation in the topsoils of riverine forests, where high levels of nutrients, trace metals and organic carbon (OC) are found. The translocation of nutrients and organic matter across the landscape to the erosion base depends on local land use trajectories. Increasing soil acidification is driving a positive feedback loop, and land use intensification has lead to degradation of local black soils within a few decades. Our data raise questions about the resilience and carrying capacity of Uruguayan soils with regard to currently implemented highly productive management forms, including the use of TPs for carbon sequestration, and supports more conservative forms of extensive management on the GL biome.
- Article
(3588 KB) - Full-text XML
- Comment
-
Supplement
(1915 KB) - BibTeX
- EndNote
Human activities alter the bio- and pedosphere, leaving a footprint of such a magnitude that it can be verified stratigraphically (Waters et al., 2016). This unprecedented transformational force is intimately related to the expansion of societies and their productive frontiers, causing a loss of biodiversity, habitat and soil degradation and, consequently, ecosystem modification (Foley et al., 2005; Borrelli et al., 2017). In this context, soil sciences have transitioned from studies on natural soil formation to the science of “anthropedogenesis” (Richter, 2020), focusing on the “soils of the Anthropocene” that are predominately agricultural (48 million km2) or urban (1.5 million km2; Ritchie and Roser, 2013).
The temperate grasslands (GLs) of South America have historically been characterized by rolling plains and low hills that have been extensively exploited for cattle production and its derivatives since the arrival of European colonization. The Río de la Plata GLs are one of our planet's four major black soil regions (Durán et al., 2011; Liu et al., 2012) and contains some of the most fertile soils in the world. Playing an important role in global food production, they are characterized as thick, humus and base cation rich and with a high cation exchange capacity throughout their profile. Maintaining these properties are therefore crucial to developing sustainable and productive agriculture (Durán et al., 2011; Liu et al., 2012).
Today, the “Uruguayan savanna” is one of the top three most critically endangered biomes (Veldman et al., 2015). In recent decades, however, the GLs have decreased due to the expansion of cash crops and Eucalyptus timber plantations (TPs), both of which are promoted by national legislation, as well as land grabbing and trans-nationalization (Piñeiro, 2012). This land use intensification, with its increased input of energy, nutrients and pesticides, leads to an overall loss of soil fertility and increasing toxicity related to acidification, salinization and contamination (Liu et al., 2012; Borrelli et al., 2017). The ecological, economic and cultural functions of soils are severely degraded, and the degradation of black soils in South America is of particular concern because they have only been heavily exploited for a comparatively short period of time (Durán et al., 2011).
Since the first decades of the twentieth-century, compared to other disciplines, soil sciences have received an extraordinary amount of attention in Uruguayan academia, governance, the productive sector and from the general public, resulting in a national soil inventory program in 1965. The subsequent classification of Uruguayan soils and their productivity (Comisión Nacional de Estudio Agronómico de la Tierra, CONEAT, index) remains an important source for today's land taxation and for management plans by legal conservation regulations. This detailed classification takes into account soil type, texture, natural vegetation, altitude and geology (Lanfranco and Sapriza, 2011).
As soil degradation is extremely relevant for countries like Uruguay, which are socioeconomically dependent on their soils (Zubriggen et al., 2020), it is a topic of discussion for local farmers, academia and the public. An update of the state of soils and related processes is needed (García-Preìchac et al., 2004; Carvalho et al., 2021), particularly as there has been little study of the impacts of Uruguayan GL intensification on soil properties (Beretta-Blanco et al., 2019). At the same time, as a new paradigm for GL intensification with a wide set of means including fertilization has been proposed to increase economic and environmental sustainability (Jaurena et al., 2021), it is urgent to obtain more insights into the dynamics of nutrients in soils of Uruguay and their availability for crops (Beretta-Blanco et al., 2019).
Soil classifications are mainly based on subsoils. However, we focus on topsoil as the most relevant and a very responsive interface for ecological processes and farmers' management. Understanding the state of topsoils and their processes is crucial for developing recommendations for sustainable land management practices. Due to the diversity of perspectives on soil quality and health and related ecosystem services, operational procedures for evaluation of soil functioning are still lacking (Bonfante et al., 2021). We contribute to a better understanding of globally occurring degradation processes among often conflicting goals such as desired soil productivity, yield limits, especially in erosion sensitive soils, and necessary soil conservation.
We therefore explored soil parameters describing current chemical conditions of topsoils that are parts of different soil groups and orders and different Uruguayan soil categories, specifically, in order to explore the gains and losses of macro- and micronutrients and soil organic carbon (SOC) across landscapes, and to determine the impact of land use change on acidification and trace metal presence and related trade-offs with soil degradation and conservation. In detail, we address the following question: how do fertility proxies such as SOC and nutrient content, acidification (pH) and trace metal accumulation in topsoils vary across different land uses (i.e., comparing GLs; TPs; native forests, NFs; and croplands, AC)? Thus, we expand the knowledge across land uses from more natural to strongly modified uses and discuss the results in light of different degradation processes such as erosion, depletion of nutrients or carbon, acidification and accumulation of pollutants and in the light of current debates on intensification.
2.1 Study area and design
Uruguay covers about 176 000 km2, and has a population of 3.5 million, mainly in urban areas. The western half of Uruguay is dominated by Mollisols, developed on a wide range of sediments from the Devonian to the Cenozoic era (partially associated with Vertisols), while the eastern plains and wetlands have, in addition to Mollisols, other soil types on slopes, rocks and on flood plains (i.e., Alfisols, Ultisols, Entisols and Inceptisols; Durán et al., 2011).
Table 1General characteristics of Uruguayan topsoils: descriptive statistics for the parameters of all single samples (n) across different land uses and classification of different soil types (for details on different land uses, i.e., GL, TP, NF and AC, see Appendix A1–A2 and on different soil types, see Appendix A3–A4). SD = standard derivation; CEC = cation exchange capacity; SOC = soil organic carbon.
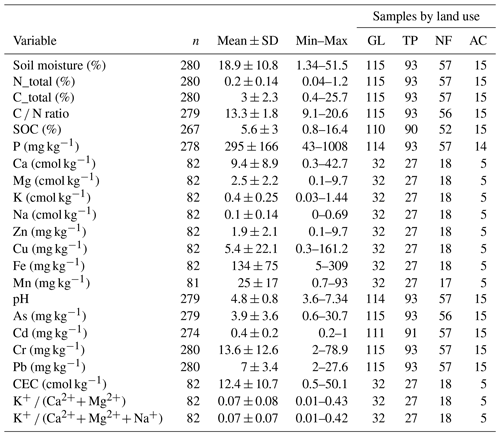
During a country-wide survey from December 2015 to March 2016, we collected 280 topsoil samples of 0–10 cm depth from 101 plots (50×50 m in GLs and AC; 100×100 m in NFs and TPs) distributed at 28 monitoring sites throughout Uruguay, South America (Fig. 1a–b; Table 1), using a stratified random design. In the first step, we randomly selected monitoring sites across the country. In the second step, we contacted landowners to explore their willingness to establish a long-term monitoring site. If the owner agreed, plot selection was stratified by different rural land use types: GLs, TPs of Pinus and Eucalyptus species, NFs and AC. NFs cover mainly riverine and park forests. The later are a savanna-like transition zones between riverine forests and the open GLs. We subdivided GL plots according to the intensity of use: (i) undisturbed GLs (without grazing), (ii) partially grazed GLs (with sporadic grazing and low animal charge), and (iii) highly grazed GLs (with high animal charge). Land use change from 1986 to 2017 follows basically four different trajectories: (i) the expansion of TPs over GLs, leading to a disaggregation of GLs by TPs; (ii) cropland expansion where crop cover maintains the open landscape character of former GLs; (iii) GL conservation of large and regularly interconnected riverine forests in a landscape dominated by GLs (Ramírez and Säumel, 2021); and (iv) GL intensification changing from natural GL to so-called “improved” or artificial GLs (Modernel et al., 2016; Jaurena et al., 2021). Fertilization and application of other agrochemicals is standard procedure for TPs, artificial GLs and industrial agriculture. We sampled topsoil three times at each land use at the edges of the plot, and stored samples below 7 ∘C until laboratory processing. The plots are placed in homogenous areas to avoid edge effects.
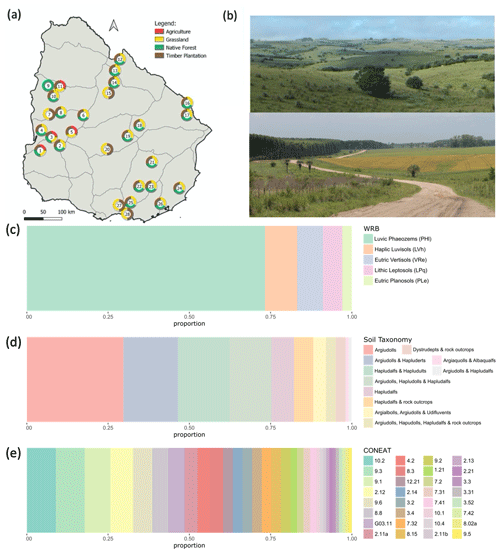
Figure 1Study design and sampling. (a) Location of the 28 monitoring sites across Uruguay including land use types sampled (GL b); TPs, NFs and AC. Proportion of plots with particular category of soil classification according to the World Reference Base (WRB, c), Soil Taxonomy (d) and CONEAT (e). The Uruguayan CONEAT index provides a detailed classification that takes into account soil type, texture, natural vegetation, altitude and geology (see details in Sect. 2.3). Photos: RuralFutures.
2.2 Analysis of soil samples
For gravimetric determination of soil moisture, the topsoil samples were dried at 105 ∘C until constant weight. Next, lumps in the samples were broken down and the remaining plant material was removed before sieving (2 mm) and then ground.
We analyzed 280 samples regarding macronutrients, pH and trace metals and 80 samples for soluble cations and micronutrients (Table 1; Sadzawka et al., 2006; Zagal and Sadzawka, 2007). Among fertility-related variables, we measured the total amount of the macronutrients phosphorus (P), soil organic carbon (SOC) and nitrogen (N), so obtaining the C N ratio. To determine total carbon and nitrogen, the samples were sieved again (0.5 mm) and analyzed using dry combustion with a LECO TruSpec CN (USA) at a combustion temperature of 950 ∘C with ultra-pure oxygen. In addition, the presence of carbonates was tested for by adding concentrated hydrochloric acid, which was negative for all soil samples analyzed.
We determined concentrations of the soluble cations calcium (Ca2+), magnesium (Mg2+), potassium (K+) and sodium (Na+) and the micronutrients copper (Cu2+), zinc (Zn2+), manganese (Mn2+) and iron (Fe) by atomic spectroscopy (Unicam AAS Solaar 969, Thermo Electron Corporation, USA), extracting with ammonium acetate (1 mol L−1, pH 7) and with DTPA–CaCl2–TEA at (pH 7.3). We calculated the cation exchange capacity (CEC). Acidity was measured by adding calcium chloride (0.01 M) to the samples at a 2.5:1 proportion, and after shaking and 2 h rest, read with a pH meter (HI2550 meter, Hanna Instruments, USA). For these variables, each 10th sample was duplicated. We categorized acidity using the USDA Natural Resources Conservation Service classification (Kellogg, 1993).
To determine the concentrations of arsenic (As), cadmium (Cd), chromium (Cr) and lead (Pb), samples were further sieved as before (0.5 mm), weighed out into a digesting container, and extracted with a mixture of nitric acid (70 %) and hydrochloric acid (30 %) in a 1:3 proportion. The digestion took place in a Titan MPS (Perkin Elmer, USA) microwave at a program suitable for this digestion (Method 3051A). During the proceedings, only deionized water Type I ASTM1193 (EC max 0.06 to 0.1 µS cm−1) was used. Reagents were used to eliminate traces of other materials and to avoid contamination of the samples. The trace metals were determined by inductively coupled plasma optical emission spectroscopy using the Optima 8000 (Perkin Elmer) with metal-associated wavelengths of 193.696 nm for As, 228.802 nm for Cd, 267.716 nm for Cr and 220.353 nm for Pb.
Total P concentration was determined calorimetrically after microwave-assisted digestion with a Unicam spectrometer at a wavelength of 660 nm. For all these variables, a repetition for each round of the microwave digestion was made.
2.3 Soil classification
We intersected the coordinates of the center of the plots with maps containing geospatial information on the classification of Uruguayan soils using ArcGIS 10.3 (ESRI, 2018). For soil group classification, we used World Reference Base for Soil Resources (WRB; IUSS Working Group, 2015); for soil orders, USDA Soil Taxonomy (Soil Survey Staff, 1999); and for local Uruguayan classification, CONEAT soil group categories, which includes the productive capacity of cattle and sheep (MGAP, 2020). The CONEAT groups are defined by their productive capacity in terms of beef, mutton and wool expressed by an index relative to the average productive capacity of the country, to which the index 100 corresponds. The classification is based on photo-interpretation at a scale of 1:40 000, field verifications and physico-chemical analysis of the soils. The productivity indices correspond to soil groups. The CONEAT groups have been defined by the dominant and associated soils according to the Soil Classification of Uruguay. The groups are related to the units of the Soil Reconnaissance Chart of Uruguay at a scale of 1:1 000 000. For each group, some important soil properties and associated landscape characteristics are indicated. The nomenclature of the CONEAT groups correlates with the Soil Use and Management Zones of Uruguay. The soil groups are superimposed on the rural parcel and are represented in the CONEAT cartography at a scale of 1:20 000 (for more details see MGAP, 2020).
2.4 Data analysis
In a first step, we explored and prepared our database for further analysis. Exploring the distribution of the soil parameters in R (R Core Team, 2021), ruled out the normality of the data using the Shapiro–Wilk test and the homogeneity of variances with the Fligner–Killeen test. We tested for outliers using the 1.5–3 interquartile range threshold and the function outlierTest from the R package car (Fox and Weisberg, 2019), reviewing the flagged observations case by case in the experimental context. The outliers were removed (Supplement Fig. S2). The variables of soils characteristics showed generally positive skewed distributions, in some cases multimodal, and tests showed evidence contrary to the assumptions of spatial autocorrelation, homoscedasticity and normality in most cases (Supplement Tables S1–S2; Figs. S1–S2).
Spearman's rank correlations (ρ) were calculated to explore linear associations between soil parameters across all single samples and within different land uses. We used the adonis function of the R package vegan v2.5-7 (Oksanen et al., 2022) with a Euclidean dissimilarity matrix from our normalized soil data to perform PERMANOVA tests with 9999 permutations to analyze the multivariate homogeneity of group dispersions based on differences in soil parameters between land uses.
To compare the general effects of land use type on topsoil parameters, non-parametric Kruskal–Wallis tests were carried out in R. When significant (p≤0.05), we used pairwise Wilcoxon rank sum tests with Benjamini and Hochberg correction (1995) to evaluate pairwise differences among land uses.
We used non-metric multidimensional scaling (NMDS) as a robust unconstrained ordination method to visualize patterns of topsoil characteristics among all samples and within subsamples (intersected with different soil orders) across different land uses using the Bray–Curtis dissimilarity matrix. The matrix was constructed with the vegan package to depict patterns of all soil parameters in two dimensions (Fig. 3a, c–f) and for the dataset without soluble cations and micronutrient variables comparing subcategories within single land use types (i.e., within GLs for undisturbed, partially grazed and highly grazed plots; TPs for Eucalyptus and Pinus plots; Fig. 3b).
Nearly 75 % of the sampled 101 plots intersected with the soil group category Luvic Phaeozem (WRB; IUSS Working Group, 2015). A quarter of our plots were distributed among four other groups (i.e., Haplic Luvisols, Eutric Vertisols, Planosols, Lithic Leptosols; Fig. 1c). Half of our plots intersected with the orders Argiudolls or Argiudolls and Hapluderts (Soil Survey Staff, 1999), and about a quarter with Hapludalfs and Hapludults or Argiudolls, Hapludolls and Hapludalfs (Fig. 1d). Our plots intersected with 32 different CONEAT categories (MGAP, 2021). The most frequent categories were 10.2, 9.3 and 2.12 (25 % of our plots) and another quarter with 9.1, 8.8, 9.6 and G03.11 (Fig. 1e).
3.1 General characteristics of Uruguayan topsoils
The measured topsoil parameters vary widely across Uruguay, between the different land uses and classification into different soil orders (Tables 1, A1–A4). The SOC in topsoils ranged between 0.8 % and 16 %, and was highest in NFs and lowest in TPs. The mean of the C N ratio was about 13, and lowest in AC soils. Phosphorous ranged between 43 and 1009 mg kg−1. We also observed a high variability in the micro- and macronutrients and trace metals. The average cation exchange capacity (CEC) was 12.41 cmol kg−1, highest in the topsoils of NFs, followed by AC and GLs and TPs (Tables 1, A1–A2).
For the whole dataset, high correlation was found between P and SOC and between P and Zn (ρ=0.82 and 0.76, respectively), and between Mg and Ca and between Mg and Na (ρ=0.82 and 0.76, respectively; Fig. 2). Similar results were observed within particular land uses, although in NFs, a negative moderate correlation between Ca and Fe was observed (Fig. S3). There was high correlation between pH and Ca (ρ=0.89; Fig. 2). In NFs, we also found a similar correlation with other soluble cations like Mg and K (Fig. S3). In park forest topsoils, there was a negative correlation between pH and As and between pH and Pb ( and , respectively). In highly grazed pastures and AC, pH was highly correlated with Cr, and in AC also with As (ρ=0.93; Fig. S3). Among trace metals, the Spearman correlation was moderate between As and Cr and between As and Pb and high between Cr and Pb (Fig. 2). These correlation trends increased in GLs, TPs and AC. We also found a high correlation between cadmium and Cr in AC (ρ=0.81). Phosphorus was highly correlated with Cr and with As in pine TPs, while in soybean crops a high correlation was found between P and Cr and between P and Pb (Fig. S3).
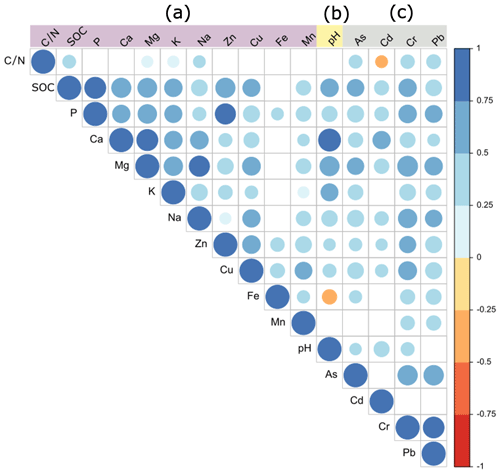
Figure 2Spearman's rank correlations for parameters of topsoils (n=80) regarding (a) fertility proxies, (b) acidity and (c) trace metals. The size of the circle refers to the absolute value of the correlation coefficient (ρ) and the color refers to the direction (positive or negative) of the correlation. Empty slots show correlations with p>0.05.
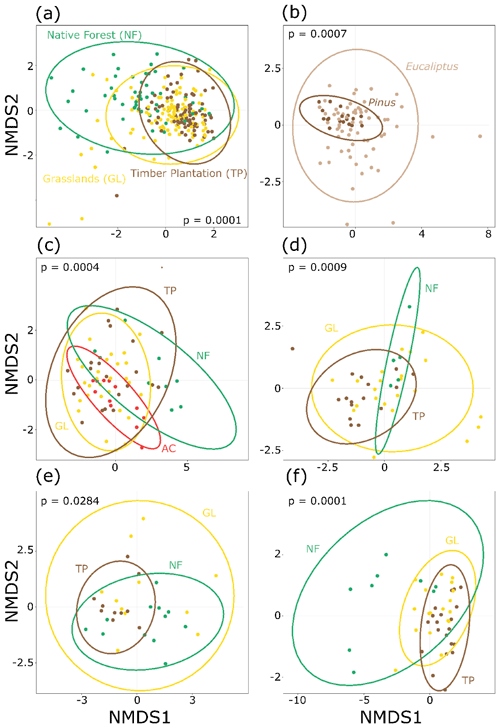
Figure 3Non-metric multidimensional scaling showing significant clustering differences among samples from (a) GLs, TPs and NFs; (b) among samples from Pinus and Eucalyptus TPs; (c) among land uses (including AC) in Argiudolls; (d) among land uses in Argiudolls and Hapluderts; (e) among land uses in Argiudolls, Hapludolls and Hapludalf and (f) among land uses in Hapludalfs and Hapludults.
3.2 Topsoil characteristics clustered by land use
We found differences in the multivariate distribution of samples according to the different land uses (i.e., GLs, TPs or NFs; Fig. 3a–f; Tables S3–S8). All pairwise comparisons of land uses showed significant differences with all variables (p=0.0001; Fig. 3a; Table S3). We analyzed subcategories within a land use type using the dataset without soluble cation and micronutrient variables, only finding significant differences between Eucalyptus and Pinus stands (p=0.0001; Fig. 3b; Table S4) but not among different GL subtypes. We also found differences when analyzing subsamples of different soil order classifications over the different land uses (Fig. 3c–f; Tables S5–S8). The samples intersected with Argiudolls included all plots on AC, and we found significant differences in all pairwise comparisons of land uses except between GLs and TPs (p=0.0004; Fig. 3c; Table S5). We further found differences between TPs and NFs at soils of the Argiudolls and Hapluderts orders (p=0.0009; Fig. 3d; Table S6) and between TPs and GLs or NFs in soils of the Argiudolls, Hapludolls and Hapludalf orders (p=0.0284; Fig. 3e; Table S7). Results for samples in Hapludalfs and Hapludults soils were similar to those obtained at the country scale (p=0.0001; Fig. 3f; Table S8).
3.3 Differences in fertility proxies
We found significantly higher fertility proxies for SOC, P, Ca, Mg and Zn in the topsoils of NFs compared to GLs and TPs (Fig. 4b–e, h). Phosphorous was significantly higher in the topsoils of GLs compared to those of TPs (Fig. 4c). Potassium was significantly higher in the topsoils of NFs compared to TPs (Fig. 4f). At the subcategories level, we found significantly higher amounts of K in partially used GLs in comparison to samples from highly grazed pastures, and higher SOC (p=0.002), P (p=0.059), Na (p=0.043), K (p=0.012) and Zn (p=0.048) in Eucalyptus TPs to Pinus TPs (Fig. S4). Among NFs, samples from riverine forests contain more Mg (p=0.023) and Na (p=0.023) in comparison to park forests.
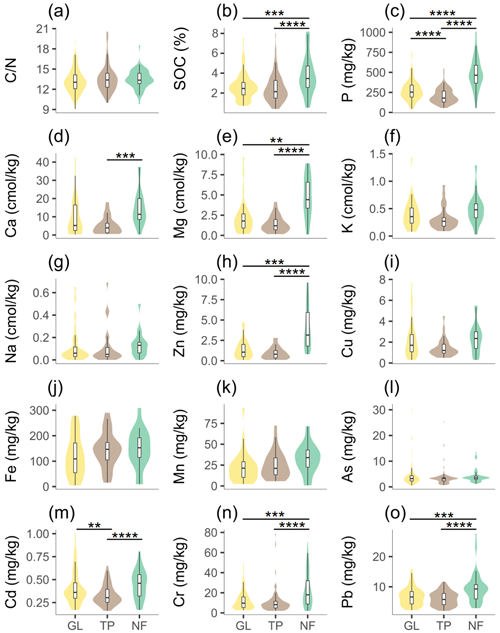
Figure 4Violin boxplots for significant Kruskal–Wallis tests across evaluated land uses (i.e., GL, TP and NF) for each variable. Significance in posterior Wilcoxon pairwise comparisons is depicted as p<0.05 (*), p<0.01 (), p<0.001 (), p<0.0001 ().
Considering all samples within the order Argiudolls, C N ratio in agricultural topsoils are lower compared to all other land uses. Phosphorus was higher in the topsoils of NFs and AC compared to GLs and TPs. SOC was highest in NFs (Fig. 5a–o; Tables A3–A4).
3.4 Soil acidification
Topsoil samples showed a markedly acid profile (median pH = 4.66), with nearly 75 % classified as “extremely acidic” and “very strongly acidic” (Fig. 6a). We found significant differences across land uses, with less acidic NFs, and lower pH in TPs (Fig. 6b). Comparing between land uses, we found more samples with neutral acidity in GLs and more with higher acidity in TPs (Fig. 6b–c). In addition, we found lower pH in samples from Pinus compared to Eucalyptus stands (p=0.018). Results of analysis inside soil orders showed similar variations observed at country scale, with TPs being more acidic and NFs closer to neutral pH (Fig. 6d–f).
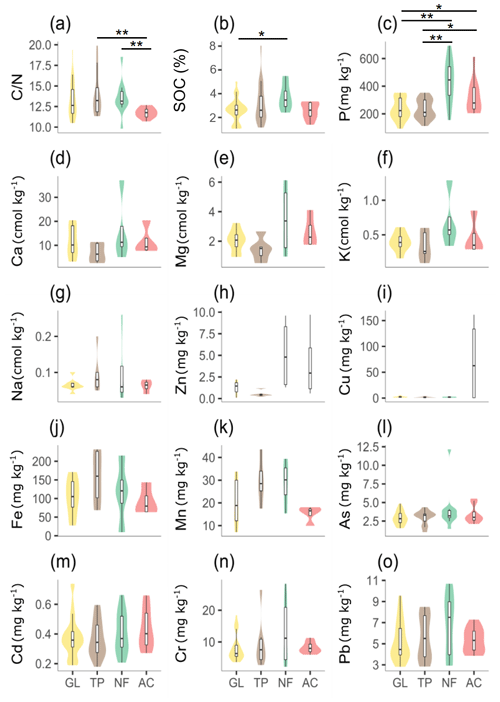
Figure 5Violin boxplots for significant Kruskal–Wallis tests in the Argiudolls soil taxonomy category for fertility variables across available land uses (GL, TP, NF, AC). Significance in posterior Wilcoxon pairwise comparisons is depicted as p<0.05 (*), p<0.01 (), p<0.001 (), p<0.0001 ().
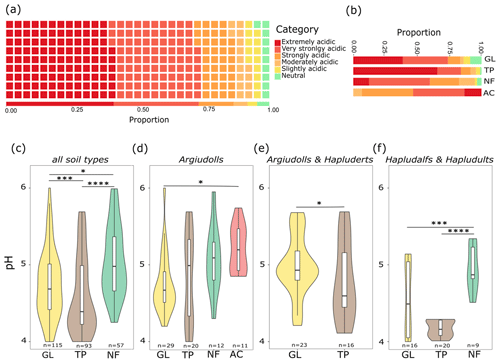
Figure 6Acidity of all topsoil samples (a) and (b) according to different land use (GL, TP, NF, AC). Proportion of samples with a given acidic category (Kellogg, 1993). pH of topsoil given as violin boxplots for significant Kruskal–Wallis tests across different land uses independent from soil order (c) and separated by soil order (d–f). Sample number (n) is given. Significance in posterior Wilcoxon pairwise comparisons is depicted as p<0.05 (*), p<0.01 (), p<0.001 (), p<0.0001 ().
3.5 Trace metal accumulation across land uses
For As, Cd, Cr and Pb, we found significantly higher concentrations in topsoils originating from NFs compared to the GL and TP samples (Figs. 4l–o and 5l–o; Tables A1–A4). At the same time, samples from Eucalyptus TPs had higher concentrations of both Cr (p<0.005) and Pb (p<0.005) than Pinus topsoils, while the same was observed for Cr (p<0.001) and Pb (p<0.05) in riverine forests compared to park forests (Fig. S5).
The vicious circle between the wish to stop soil degradation and concurrent increases in land productivity to satisfy the increasing demand for food, fiber and energy has not been broken since the Green Revolution. Socioeconomic and conventional management practices that drive soil degradation have generated several traps, such as the “inputs trap” where a reduced yield per area is followed by higher fertilizer application or the “credit or poverty trap” where economic pressure forces farmers to practice intensification (Gomiero, 2016). Caught in this loop, the soils of the temperate GLs of Uruguay have suffered strong degradation from erosion, acidification, contamination, salinification and compaction (Zurbriggen et al., 2020). This is clearly reflected in the results of our topsoil survey, which also adds interesting insights from TPs, GLs and NFs to an existing database consisting mainly of cropland and pastures samples from 2002–2014, which demonstrated the loss of organic matter by 25 % and an increasing loss of nutrients (Beretta-Blanco et al., 2019). We contribute deeper insights on fertility, acidification and trace metal accumulation in topsoils from a wide range of different land uses, which is, to our knowledge, unique for the region since the CONEAT classification (CONEAT Index, 1976).
4.1 Translocation of elements in topsoils within landscapes
Our data demonstrate the high amounts of organic carbon (OC), nutrients and trace metals in topsoil samples from riverine forests, suggesting transport of soil particles from the surrounding land uses (e.g., GLs, AC or TPs) to the borders of rivers, streams and creeks. Therefore, we assume that OC, nutrients and trace metals are displaced within the landscape and accumulate in the floodplains. Regional soil erosion models estimate the loss of 2–5 t ha−1 yr−1 for one-third of the country depending on precipitation, topography, soil erodibility and land management (Carrasco-Letelier and Beretta-Blanco, 2017). One possible direct impact is the increasing eutrophication reported for larger local rivers, although the models used by these authors did not link Chlorophyll a concentrations with agricultural land use (see Beretta-Blanco and Carrasco-Letelier, 2021, and replies). However, other vertical processes and differences across land uses can be hidden deeper in the soil profile, and have not been analyzed in this study.
Organic carbon content and the exchangeable cations are strongly reduced in the topsoils of GLs, TPs and AC compared to NFs (Figs. 4b, d–h and 5b, d–h). Of all the fertility proxies assessed, phosphorus in topsoil was most significantly affected by different land uses, being highest in NFs (Figs. 4c and 5c). The cation exchange capacity (CEC) was highest in NFs (more than 160 % of the CEC in GLs) and lowest in TPs, reaching only half of the CEC in GLs (Tables A1–A2). Lower average nutrient concentrations and corresponding CEC reported for two TPs in the east and one in the northwest of Uruguay (Hernández et al., 2016, 2009; Céspedes-Payret et al., 2012) may result from a combined effect of topsoil degradation in TPs due to management practices and soil texture (Sandoval-Lopez et al., 2020). The trees' uptake and the general export of nutrients from fast growing TPs through harvesting is higher than the natural input into those systems (Merino et al., 2005). This effect is particularly relevant because TPs in Uruguay are on “forestry priority soils”, which are generally soils with low fertility, of superficial to moderate depth and with good drainage (OAS, 1994), so afforestation might reduce even more their soil fertility.
Comparing neighboring GL and afforested GL sites, our dataset shows no significant depletion of nutrients by TPs (p=0.208) but a slightly higher average of CEC at GLs (Table A1). In general, plants are expected to compensate for the extraction of cations from upper soil by the “uplift of nutrient” from deeper horizons (Jobbagy and Jackson, 2004). This has been questioned for Eucalyptus TPs on sandy soils, where, for example, an increase of potassium in the topsoil was not observed compared to the neighboring GL (Céspedes-Payret et al., 2012). Phosphorous and cations decline with sand content, so physicochemical factors such as the percentage of sand and organic matter influence soil fertility (Sandoval-Lopez et al., 2020). A study of Eucalyptus TPs in southeast Brazil did not show a significant depletion of nutrients and carbon; in contrast, carbon, potassium and calcium increased in the topsoil after 12 years over one or two harvest and fertilization cycles (McMahon et al., 2019). To sum up, the patterns observed in the various sites in our survey and the other studies indicate very complex interactions of numerous factors. Removal of nutrients by high-yield TPs may exceed the capacity of nutrient exchange or turnover of forest litter and wastes. Both stand management and environmental conditions (e.g., precipitation) influence nutrient and carbon stocks (McMahon et al., 2019; Sandoval-Lopez et al., 2020).
The C N ratio in topsoils ranged within values reported for GLs and TPs of the region (see Berthrong et al., 2012). In contrast to that study, however, we observed no differences between GLs and TPs (Fig. 4a), but between topsoils of TPs, NFs and AC (Fig. 5a). Organic matter and nitrogen decrease in topsoils after GL afforestation (Berthrong et al., 2009) and the C N ratio increases with plantation age and decreases with precipitation (Berthrong et al., 2012). The topsoils of TPs have, on average, lower N concentrations compared to other land uses (Table A2) and data in literature (Jobbagy and Jackson, 2003).
Although nutrients are regularly provided by increased application of fertilizers in croplands (Beretta-Blanco et al., 2019), TPs are usually fertilized only in the first year of planting (e.g., Binkley et al., 2017; Sandoval-Lopez et al., 2020). The CEC and the average ratio between K+(Ca Mg in AC and GLs are in the ranges reported by Beretta-Blanco et al. (2019) with lower availability of potassium for crops. A shortage of potassium and calcium is also most likely for future TPs after harvest, especially if the logging process do not include bark stripping on site (Hernández et al., 2009) and due to the short time spans between harvest and new planting in the same area (Sandoval-Lopez et al., 2020, but see contrasting results of McMahon et al. 2019). The extraction of nutrients and biomass due to grazing (Fernández et al., 2017), timber (Hernández et al., 2016, 2009) and crop production (Beretta-Blanco et al., 2019) lowers organic matter content and exchangeable cations, affects the physical, chemical and biological soil properties and drives degradation.
4.2 Acidification in Uruguayan topsoils across land uses
A further dimension of the soil degradation directly linked to cation extraction is the acidification of soils. This has been demonstrated for crops and pastures (Beretta-Blanco et al., 2019) and a study site with Eucalyptus (Céspedes-Payret et al., 2012) and is now broadly supported by our topsoil samples originating from a wide range of different land uses across Uruguay. The pH of our topsoil samples are mainly in the category of very strongly to extremely acidic and is lowest in TPs (Fig. 6), which are below the means reported so far (Jobbagy and Jackson, 2003; Céspedes-Payret et al., 2012). Moreover, as our data on topsoil pH fall short compared to the values estimated by the Food and Agriculture Organization of the United Nations and the Intergovernmental Technical Panel on Soils (Caon and Vargas, 2017), acidification and the deterioration of topsoil quality continues. Acidification results from intensified land use with nitrogen fertilization, with biological N fixation by legumes both used in improved pastures (Modernel et al., 2016) or with cation extraction by crop or timber harvest (Jobbagy and Jackson, 2003). So far, although forest soils tend to be more acidic than agricultural soils due to acid-neutralizing treatments in the latter (Baize and van Oort, 2014), we found no differences between the topsoils of croplands and NFs (Fig. 6d) as the high SOC content in NFs buffers the process to a certain extent.
4.3 Riverine forest soils as sink for trace metals
In general, the average concentration of Zn, Cu, Cd, Cr, As and Pb in our topsoils were within the expected ranges of samples from croplands, pastures and GL in the region (Lavado et al., 1998, 2004; Roca, 2015). Some samples, especially from orchards and croplands, exceeded the background values of copper, cadmium and arsenic (Table A2; Roca, 2015). Little is known about the pedo-geochemical background of the Pampean soils (Roca, 2015): for example, high arsenic concentrations in Uruguayan groundwater have been hypothesized to be due to quaternary ash deposits (Wu et al., 2021). In addition, the lateral heterogeneity of Pampean soils over short distances makes separating geochemical and anthropic signatures difficult (Roca, 2015). However, that the main risk of soil contamination in the region is from the application of fertilizers and agrochemicals is uncontested (Kaushal et al., 2018).
To our knowledge, there has been no regional study of trace metals in native riverine forests or TPs. Our work thus expands the evidence base for these land uses. The topsoils of riverine forests accumulate more trace metals compared to those of TPs and croplands (Fig. 4l–o). The higher amount of soil organic matter in riverine forests favors the retention of cations, including trace metals. Although the origin of potentially harmful elements in forest soils has been primarily attributed to atmospheric deposition (Baize and van Oort, 2014), atmospheric deposition only plays a major role in the vicinity of urban or industrial developments, and our data from rural sites suggest a different entry path from the surrounding land use to riverine forests. High acidification and low amounts of organic matter reduce the retention of trace metals in the soil of TPs, and elements leach out of the soil towards the water table (Baize and van Oort, 2014). Acidification strongly contributes to the overall mobility of base cations into the “chemical cocktail of the Anthropocene” (Kaushal et al., 2018), including trace metals. We thus observe positive feedback in already impoverished soils with high acidity favoring cation solubility, in addition to the uptake by trees intensifying this effect. TPs extract trace metals from the soil and accumulate them in bark or leaves, so they have been used for phytoremediation (Li et al., 2020). This may explain the higher concentration of cadmium in GLs compared to TPs (Fig. 4m). Differences between Eucalyptus and Pinus stands may be related to different age classes, as the later may have extracted more lead and chromium from the soil due to their older stand age, with rotation periods of about 20 years (Li et al., 2020).
4.4 Carbon storage in topsoils of Eucalyptus plantations
Our study provides evidence that the loss of SOC limits not only the productivity of croplands, but also potential carbon sequestration in the region (Beretta-Blanco et al., 2019). GL conversion to cropland decreases SOC storage compared to adjacent native GLs (Hernandez-Ramirez et al., 2021).
Afforestation of croplands has been also discussed as a carbon sequestration measure to proactively address and effectively mitigate ongoing climate change within a person's lifetime (Hernandez-Ramirez et al., 2021; Mayer et al., 2020). The carbon stocks of four Eucalyptus stands in the Brazilian Cerrado increased and did not change in four Eucalyptus stands within the Atlantic Forest (McMahon et al., 2019); however, this may not be the case for short rotation of Eucalyptus TPs in Campos GLs. In our topsoil samples originating from 28 different stands across Uruguay, OC is lowest in the topsoils of TPs (Fig. 4b). Similar amounts have been reported for a TP in the east of Uruguay (Céspedes-Payret et al., 2012) and in northeastern Argentina (Sandoval-Lopez et al., 2020). Our data therefore provide clear evidence that, rather than contributing to carbon sequestration in the topsoil, the carbon released from the transformation of native GLs to TPs with these fast-growing species has several adverse effects depending on precipitation and soil type (reviewed by Mayer et al., 2020).
Several trade-offs between carbon sequestration through afforestation and local water yield and soil fertility have been demonstrated, including nutrient and soil organic matter depletion, acidification and biodiversity loss and corresponding challenges for landscape conservation (e.g., Jackson et al., 2005; Veldman et al., 2015; Friggens et al., 2020). Soil carbon changes dynamically during the first decade of afforestation. Remaining GL carbon declines, while tree carbon gain starts (e.g., Paul et al., 2002). Although a net gain of carbon is expected when the new forests approached equilibrium after some decades (Hernández-Ramirez et al., 2021), in contrast to long-lasting forests, Eucalyptus harvest in Uruguay takes place after less than a decade (approx. 7–10 years). SOC does not differ between Eucalyptus TPs and neighboring GLs (Appendix A; Tables A1 and A2). Another study near our sites 12 and 13 also did not find a significant difference between the SOC of the upper soil (0–30 cm) compared to afforested versus native GLs (Hernández et al., 2016). A study near our sites 7, 8 and 10 reported higher topsoil carbon in GL compared with afforested sites in their vicinity only if the sand content is lower than 60 % (Sandoval-Lopez et al., 2020). McMahon et al. (2019) identified a greater carbon gain under Eucalyptus stands compared to (potential) carbon losses in neighboring degraded Cerrado GLs. There is evidence that the retention of residues after harvest increases the carbon stock in soil (Mayer et al., 2020). Long-term monitoring of carbon stocks is needed to verify the influences of management and environmental changes. A regional study on Eucalyptus TPs across different biomes in Brazil shows both decreases and no changes depending on precipitation in the dry season, clay content and on the initial stock of carbon in the soil (Cook et al., 2016)
The simplistic solution of huge TPs to compensate for anthropogenic CO2 emissions has been challenged in the last decade, and some crucial lessons learned have been summarized (Di Sacco et al., 2020). Since GLs are more sustainable carbon sinks compared to climate-vulnerable monocultures such as TPs (Dass et al., 2018), avoiding afforestation of previously non-forested lands is important. This is the case for Eucalyptus afforestation in the originally forestless GLs of Uruguay: models of carbon sequestration and dynamics in Mollisols and Oxisols under South American GLs estimated a higher carbon retention efficiency under GLs compared to afforested sites, suggesting that silvopastoral systems are a potential solution for soil carbon sequestration in tropical soils (Berhongaray and Alvarez, 2019).
Connecting or expanding existing forests and using native species for plantings (Di Sacco et al., 2020) is also recommended (see also Alfaro et al., 2020; Ortiz et al., 2020). Our topsoil data indicate that carbon sequestration occurs mainly in the topsoils of native riverine forests that cover less than 5 % of Uruguayan territory. Consequently, the expansion of NFs and the use of native species in forestry projects for long-term establishment can reduce the adverse effects of TPs. While there is preliminary evidence that N-fixing tree species can help increase local C stocks in afforestation, because of their potential for invasion, exotic N-fixers should be avoided (Mayer et al., 2020).
Recent studies indicated that novel techniques such as perennial grain cropping can help to turn cropland degradation into beneficial cropland aggradation by using the advantage of perennial vegetation of conserving and even enhancing short- and long-term soil carbon storage and other ecosystems services (Kim et al., 2022).
4.5 Back to more conservative grassland management?
Our soil survey data show strong soil degradation of Uruguayan black soils from erosion, acidification and contamination and suggest a translocation of nutrients and OC across the landscape from GLs, TP and AC to the riverine forests. The potential of GLs as cropland reserves has been largely overestimated (Lambin et al., 2013) and they have already degraded during the last decades by inappropriate land management techniques (Jaurena et al., 2021; Carvalho et al., 2021), lack of mainstreaming soil conservative techniques (García-Préchac, 2004; Fernandez et al., 2017), decoupling of crop and livestock (Carvalho et al., 2021) and climate change impacts with storm water events and drought, all of which trigger soil erosion (Wingeyer et al., 2015). From the although very limited point of view on topsoils, the concept of conserving “old growth grasslands” with extensive use (Veldman et al., 2015) appears to be a more promising strategy to put the “grasslands at the core” in the Campos region than the use intensification strategies envisioned by Jaurena et al. (2021).
The land use intensification in Uruguay associated with increasing inputs of energy, nutrients and pesticides leads to an overall loss of soil fertility and increasing toxicity related to acidification, salinization and trace metal contaminants. Our data demonstrate the high amounts of OC, nutrients and trace metals in the topsoil samples from riverine forests, suggesting transport of soil particles from the surrounding GLs, TP and AC to the borders of rivers, streams and creeks. Of all the fertility proxies assessed, phosphorus in topsoil was most significantly affected by different land uses, being highest in NFs. Cation exchange capacity was also highest in NFs and lowest in TPs, where only half that of GLs was measured. Our study highlights that soil acidification is ongoing and probably also mobilizing trace metals and their accumulation in riverine forest topsoils.
Table A1Descriptive statistics of topsoil variables for all single soil types in GLs and NFs. Number of samples (n), mean, standard deviation (SD), minimum (Min) and maximum (Max) of each variable are given.
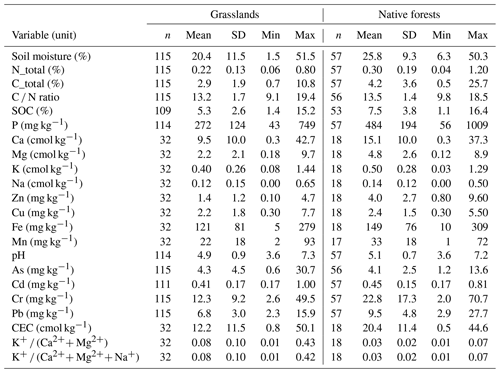
Table A2Descriptive statistics of topsoil variables for all single soil types at TPs and AC. Number of samples (n), mean, standard deviation (SD), minimum (Min) and maximum (Max) of each variable are given.
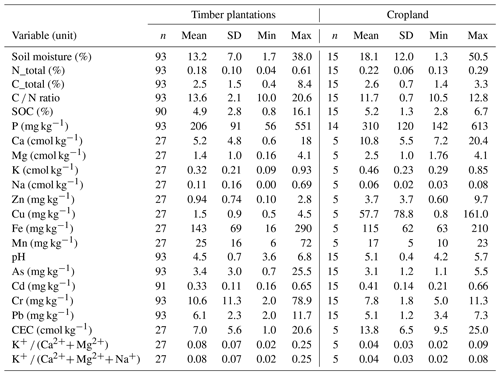
Table A3Descriptive statistics of topsoil variables for Argiudolls overall and for GLs and NFs with Argiudolls. Number of single samples (n), mean, standard deviation (SD), minimum (Min) and maximum (Max) of each variable are given.
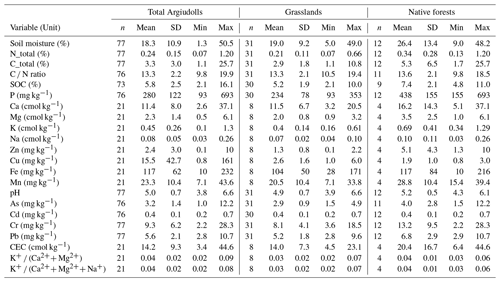
All data generated or analyzed in this study are included in this article (and its Supplement). Further data are available from the corresponding authors upon reasonable request.
The supplement related to this article is available online at: https://doi.org/10.5194/soil-9-425-2023-supplement.
IS, LRR, ST and MB conceptualized the study, performed the soil sampling and undertook the data analysis. EZ advised in the laboratory analyses. IS wrote the initial draft. LRR, ST, MB and EZ contributed to generating and reviewing the subsequent versions of the manuscript. IS received the funding for the study.
The contact author has declared that none of the authors has any competing interests.
Publisher’s note: Copernicus Publications remains neutral with regard to jurisdictional claims in published maps and institutional affiliations.
In alphabetical order, we thank Juan Barreneche, Lucia Gaucher, Sören Miehe, Nicolas Silvera and Matias Zarucki for their assistance in field work. We thank Manuel Garcia and Meica Valdivia for the pre-processing of the samples. We thank the staff of the soil laboratory from the Department of Soil and Natural Resources, University of Concepción, Chile. We thank Diego de Panis for help with statistical analysis and visualization and valuable comments on a previous version of this manuscript. Thanks to Vera Krause, Serafina Bischoff, Sophia Reitzug, Rhea Rennert and Diego Nicolas Rojas for support with the final editions of plots and maps. We also thank all landowners for access permission to establish our monitoring sites on their land and their hospitality and willingness to discuss land use goals concerning all dimension of sustainability. The study was funded by the German Federal Ministry of Education and Research (BMBF; 01LN1305A). Special thanks go to Amal Chatterjee for improving our English.
This research has been supported by the Bundesministerium für Bildung und Forschung (grant no. 01LN1305A).
This open-access publication was funded by the Humboldt-Universität zu Berlin.
This paper was edited by Nikolaus J. Kuhn and reviewed by three anonymous referees.
Alfaro, M., Dube, F., and Zagal, E.: The Influence of Overmature, Degraded Nothofagus Forests with Strong Anthropic Disturbance on the Quality of an Andisol and its Gradual Recovery with Silvopasture in Southwestern South America, in: Agroforestry for Degraded Landscapes, edited by: Dagar, J. C., Gupta, S. R., and Teketay, D., Springer, Singapore, 67–85, https://doi.org/10.1007/978-981-15-6807-7_3, 2020.
Baize, D. and van Oort, F.: Potentially Harmful Elements in Forest Soils, in: PHEs, Environment and Human Health, edited by: Bini, C. and Bech, J., Springer Netherlands, Dordrecht, 151–198, https://doi.org/10.1007/978-94-017-8965-3_4, 2014.
Benjamini, Y. and Hochberg, Y.: Controlling the False Discovery Rate: A Practical and Powerful Approach to Multiple Testing, J. Roy. Stat. Soc. Ser. B, 57, 289–300, 1995.
Beretta-Blanco, A., Pérez, O., and Carrasco-Letelier, L.: Soil quality decrease over 13 years of agricultural production, Nutr. Cycl. Agroecosyst., 114, 45–55, 2019.
Beretta-Blanco, A. and Carrasco-Letelier, L.: Relevant factors in the eutrophication of the Uruguay River and the Río Negro, Sci. Total Environ., 761, 143299, https://doi.org/10.1016/j.scitotenv.2020.143299, 2021.
Berhongaray, G. and Alvarez, R.: Soil carbon sequestration of Mollisols and Oxisols under grassland and tree plantations in South America – A review, Geoderma Regional, 18, e00226, https://doi.org/10.1016/j.geodrs.2019.e00226, 2019.
Berthrong, S. T., Jobbágy, E. G., and Jackson, R. B.: A global meta-analysis of soil exchangeable cations, pH, carbon, and nitrogen with afforestation, Ecol. Appl., 19, 2228–2241, https://doi.org/10.1890/08-1730.1, 2009.
Berthrong, S. T., Piñeiro, G., Jobbágy, E. G., and Jackson, R. B.: Soil C and N changes with afforestation of grasslands across gradients of precipitation and plantation age, Ecol. Appl., 22, 76–86, https://doi.org/10.1890/10-2210.1, 2012.
Binkley, D., Campoe, O. C., Alvares, C., Carneiro, R. L., Cegatta, Í., and Stape, J. L.: The interactions of climate, spacing and genetics on clonal Eucalyptus plantations across Brazil and Uruguay. Forest Ecology And Management, Amsterdam, Elsevier Science Bv., 405, 271–283, https://doi.org/10.1016/j.foreco.2017.09.050, 2017.
Bonfante, A., Basile, A., and Bouma, J.: Targeting the soil quality and soil health concepts when aiming for the United Nations Sustainable Development Goals and the EU Green Deal, SOIL, 6, 453–466, https://doi.org/10.5194/soil-6-453-2020, 2020.
Borrelli, P., Robinson, D. A., Fleischer, L. R., Lugato, E., Ballabio, C., Alewell, C., Meusburger, K., Modugno, S., Schütt, B., Ferro, V., Bagarello, V., Oost, K. V., Montanarella, L., and Panagos, P.: An assessment of the global impact of 21st century land use change on soil erosion, Nat. Commun., 8, 2013, https://doi.org/10.1038/s41467-017-02142-7, 2017.
Caon, L. and Vargas, R.: Threats to soils: Global trends and perspectives, A Contribution from the Intergovernmental Technical Panel on Soils, Global Soil Partnership Food and Agriculture Organization of the United Nations, in: Global land outlook working paper, edited by: Pierzynski, G. and Brajendra, 28 pp., https://www.unccd.int/sites/default/files/2018-06/17.%20Threats%2Bto%2BSoils__Pierzynski_Brajendra.pdf (last access: 9 January 2022), 2017.
Carrasco-Letelier, L. and Beretta-Blanco, A.: Soil erosion by water estimated for 99 Uruguayan basins, Cien. Inv. Agr., 44, 184–194, 2017.
Céspedes-Payret, C., Piñeiro, G., Gutiérrez, O., and Panario, D.: Land use change in a temperate grassland soil: Afforestation effects on chemical properties and their ecological and mineralogical implication, Sci. Total Environ., 438, 549–557, https://doi.org/10.1016/j.scitotenv.2012.08.075, 2012.
Cook, R. L., Binkley, D., and Stape, J. L.: Eucalyptus plantation effects on soil carbon after 20years and three rotations in Brazil, Forest Ecol. Manag., 359, 92–98, https://doi.org/10.1016/j.foreco.2015.09.035, 2016.
Dass, P. et al.: Grasslands may be more reliable carbon sinks than forests in California, Environ. Res. Lett., 13, 074027, https://doi.org/10.1088/1748-9326/aacb39, 2018.
Carvalho, P. C. D. F., Savian, J. V., Chiesa, T. D., Filho, W. D. S., Terra, J. A., Pinto, P., Martins, A. P., Villarino, S., Trindade, J. K. D., Nunes, P. A. D. A., and Piñeiro, G.: land-use intensification trends in The Rio De La Plata region of South America: toward specialization or recoupling crop and livestock production, Front. Agr. Sci. Eng., 8, 97–110, https://doi.org/10.15302/J-FASE-2020380, 2021.
Di Sacco, A., Hardwick, K., Blakesley, D., Brancalion, P. H. S., Breman, E., Cecilio Rebola, L., Chomba, S., Dixon, K., Elliott, S., Ruyonga, G., Shaw, K., Smith, P., Smith, R. J., and Antonelli, A.: Ten Golden Rules for Reforestation to Optimise Carbon Sequestration, Biodiversity Recovery and Livelihood Benefits, Glob. Change Biol., 27, 1328–1348, https://doi.org/10.1111/gcb.15498, 2020.
Durán, A., Morrás, H., Studdert, G., and Liu, X.: Distribution, properties, land use and management of Mollisols in South America, Chin. Geogr. Sci., 21, 511–530, https://doi.org/10.1007/s11769-011-0491-z, 2011.
ESRI: ArcGIS Desktop: Release 10.3, Redlands, CA, Environmental Systems Research Institute, https://www.nrcs.usda.gov/Internet/FSE_DOCUMENTS/nrcs142p2_051232.pdf (last access: 18 April 2021), 2018.
Fernández, R., Frasier, I., Noellemeyer, E., and Quiroga, A.: Soil quality and productivity under zero tillage and grazing on Mollisols in Argentina – A long-term study, Geoderma Regional, 11, 44–52, https://doi.org/10.1016/j.geodrs.2017.09.002, 2017.
Foley, J. A., DeFries, R., Asner, G. P., Barford, C., Bonan, G., Carpenter, S. R., Chapin, F. S., Coe, M. T., Daily, G. C., Gibbs, H. K., Helkowski, J. H., Holloway, T., Howard, E. A., Kucharik, C. J., Monfreda, C., Patz, J. A., Prentice, I. C., Ramankutty, N., and Snyder, P. K.: Global Consequences of Land Use, Science, 309, 570–574, https://doi.org/10.1126/science.1111772, 2005.
Fox, J. and Weisberg, S.: An R Companion to Applied Regression,SAGE Publications Inc, ISBN: 978-1-5443-3647-3, 608 pp., 2019.
Friggens, N. L., Hester, A. J., Mitchell, R. J., Parker, T. C., Subke, J., and Wookey, P. A.: Tree planting in organic soils does not result in net carbon sequestration on decadal timescales, Glob. Change Biol., 26, 5178–5188, https://doi.org/10.1111/gcb.15229, 2020.
Garcıìa-Préchac, F., Ernst, O., Siri-Prieto, G., and Terra, J. A.: Integrating no-till into crop–pasture rotations in Uruguay, Soil Till. Res., 77, 1–13, https://doi.org/10.1016/j.still.2003.12.002, 2004.
Gomiero, T.: Soil Degradation, Land Scarcity and Food Security: Reviewing a Complex Challenge, Sustainability, 8, 281, https://doi.org/10.3390/su8030281, 2016.
Hernaìndez, J., del Pino, A., Salvo, L., and Arrarte, G.: Nutrient export and harvest residue decomposition patterns of a Eucalyptus dunnii Maiden plantation in temperate climate of Uruguay, Forest Ecol. Manag., 258, 92–99, https://doi.org/10.1016/j.foreco.2009.03.050, 2009.
Hernández, J., del Pino, A., Vance, E. D., Califra, Á., Del Giorgio, F., Martínez, L., and González-Barrios, P.: Eucalyptus and Pinus stand density effects on soil carbon sequestration, Forest Ecol. Manag., 368, 28–38, https://doi.org/10.1016/j.foreco.2016.03.007, 2016.
Hernandez-Ramirez, G., Sauer, T. J., Chendev, Y. G., and Gennadiev, A. N.: Nonlinear turnover rates of soil carbon following cultivation of native grasslands and subsequent afforestation of croplands, SOIL, 7, 415–431, https://doi.org/10.5194/soil-7-415-2021, 2021.
IUSS Working Group WRB: World Reference Base for Soil Resources 2014, update 2015 International soil classification system for naming soils and creating legends for soil maps, World Soil Resources Reports No. 106, FAO, Rome, https://www.fao.org/3/i3794en/I3794en.pdf (last access: 5 November 2020), 2015.
Jackson, R. B., Jobbágy, E. G., Avissar, R., Roy, S. B., Barrett, D. J., Cook, C. W., Farley, K. A., le Maitre, D. C., McCarl, B. A., and Murray, B. C.: Trading Water for Carbon with Biological Carbon Sequestration, Science, 310, 1944–1947, https://doi.org/10.1126/science.1119282, 2005.
Jaurena, M., Durante, M., Devincenzi, T., Savian, J. V., Bendersky, D., Moojen, F. G., Pereira, M., Soca, P., Quadros, F. L. F., Pizzio, R., Nabinger, C., Carvalho, P. C. F., and Lattanzi, F. A.: Native Grasslands at the Core: A New Paradigm of Intensification for the Campos of Southern South America to Increase Economic and Environmental Sustainability, Front. Sustain. Food Syst., 5, 547834, https://doi.org/10.3389/fsufs.2021.547834, 2021.
Jobbagy, E. G. and Jackson, R. B.: Patterns and mechanisms of soil acidification in the conversion of grasslands to forests, Biogeochemistry, 64, 205–229, 2003.
Jobbagy, E. G. and Jackson, R. B.: The uplift of soil nutrients by plants: biogeochemical consequences across scales, Ecology, 85, 2380–2389, 2004.
Kaushal, S. S., Gold, A. J., Bernal, S., Johnson, T. A. N., Addy, K., Burgin, A., Burns, D. A., Coble, A. A., Hood, E., Lu, Y., Mayer, P., Minor, E. C., Schroth, A. W., Vidon, P., Wilson, H., Xenopoulos, M. A., Doody, T., Galella, J. G., Goodling, P., Haviland, K., Haq, S., Wessel, B., Wood, K. L., Jaworski, N., and Belt, K. T.: Watershed “chemical cocktails”: forming novel elemental combinations in Anthropocene fresh waters, Biogeochemistry, 141, 281–305, https://doi.org/10.1007/s10533-018-0502-6, 2018.
Kellogg, C. E.: Soil survey division staff: soil survey manual, Chap. 3, United States Department of Agriculture, Washington, 1993.
Kim, K., Daly, E. J., Gorzelak, M., and Hernandez-Ramirez, G.: Soil organic matter pools response to perennial grain cropping and nitrogen fertilizer, Soil Till. Res., 220, 105376, https://doi.org/10.1016/j.still.2022.105376, 2022.
Lambin, E. F., Gibbs, H. K., Ferreira, L., Grau, R., Mayaux, P., Meyfroidt, P., Morton, D. C., Rudel, T. K., Gasparri, I., and Munger, J.: Estimating the world's potentially available cropland using a bottom-up approach, Glob. Environ. Change, 23, 892–901, 2013.
Lanfranco, B. and Sapriza, G.: El índice CONEAT como medida de productividad y valor de la tierra, Serie Técnica No. 17, ISBN: 978-9974-38-307-4, http://www.ainfo.inia.uy/digital/bitstream/item/2643/1/18429020511102255.pdf (last acces: 24 December 2021), 2011.
Lavado, R. S., Rodrírguez, M. B., Scheiner, S. D., Taboada, M. A., Rubio, G., Alvarez, R., Alconada, M., and Zubillaga, M. S.: Heavy metals in soils of Argentina: comparison between urban and agricultural soils, Commun. Soil Sci. Plant Anal., 29, 1913–1917, 1998.
Lavado, R. S., Zubillaga, M. S., Alvarez, R., and Taboada, M. A.: Baseline levels of potentially toxic elements in pampas soils, Soil and Sediment Contamination: An International Journal, 13, 329–339, 2004.
Li, H., Jiang, L., You, C., Tan, B., and Yang, W.: Dynamics of heavy metal uptake and soil heavy metal stocks across a series of Masson pine plantations, J. Clean. Prod., 269, 122395, https://doi.org/10.1016/j.jclepro.2020.122395, 2020.
Liu, X., Lee Burras, C., Kravchenko, Y. S., Durán, A., Huffman, T., Morras, H., Studdert, G., Zhang, X., Cruse, R. M., and Yuan, X.: Overview of Mollisols in the world: Distribution, land use and management, Can. J. Soil. Sci., 92, 383–402, https://doi.org/10.4141/cjss2010-058, 2012.
Mayer, M., Prescott, C. E., Abaker, W. E. A., Augusto, L., Cécillon, L., Ferreira, G. W. D., James, J., Jandl, R., Katzensteiner, K., Laclau, J.-P., Laganière, J., Nouvellon, Y., Paré, D., Stanturf, J. A., Vanguelova, E. I., and Vesterdal, L.: Influence of forest management activities on soil SOC stocks: A knowledge synthesis, Forest Ecol. Manag., 466, 118127, https://doi.org/10.1016/j.foreco.2020.118127, 2020.
McMahon, D. E., Vergütz, L., Valadares, S. V., da Silva, I. R., and Jackson, R. B.: Soil nutrient stocks are maintained over multiple rotations in Brazilian Eucalyptus plantations, Forest Ecol. Manag., 448, 364–375, https://doi.org/10.1016/j.foreco.2019.06.027, 2019.
Merino, A., Balboa, M. A., Rodríguez Soalleiro, R., and González, J. G. Á.: Nutrient exports under different harvesting regimes in fast-growing forest plantations in southern Europe, Forest Ecol. Manag., 207, 325–339, https://doi.org/10.1016/j.foreco.2004.10.074, 2005.
MGAP (Ministerio de Ganadería, Agricultura y Pesca): CONEAT, https://www.gub.uy/ministerio-ganaderia-agricultura-pesca/politicas-y-gestion/coneat (last access: 6 March 2021), 2020.
Modernel, P., Rossing, W. A. H., Corbeels, M., Dogliotti, S., Picasso, V., and Tittonell, P.: Land use change and ecosystem service provision in Pampas and Campos grasslands of southern South America, Environ. Res. Lett., 11, 113002, https://doi.org/10.1088/1748-9326/11/11/113002, 2016.
OAS (Organization of American States, Department of Sustainable Development): Uruguay – Proyecto Regional de Alternativas para la Inversión Forestal, http://www.oas.org/dsd/publications/unit/oea20s/oea20s.pdf (last access: 9 March 2021), 1994.
Oksanen, J., Blanchet, F. G., Friendly, M., Kindt, R., Legendre, P., McGlinn, D., Minchin, P. R., O'Hara, R. B., Simpson, G. L., Solymos, P., Stevens, M. H. H., Szoecs, E., and Wagner, H.: vegan: Community Ecology Package, R package version 2.6-4, https://CRAN.R-project.org/package=vegan (last access: 29 March 2021), 2022.
Ortiz, J., Dube, F., Neira, P., Panichini, M., Stolpe, N. B., Zagal, E., and Martínez-Hernández, P. A.: Soil Quality Changes within a (Nothofagus obliqua) Forest Under Silvopastoral Management in the Andes Mountain Range, South Central Chile, Sustainability, 12, 6815, https://doi.org/10.3390/su12176815, 2020.
Paul, K. I., Polglase, P. J., Nyakuengama, J. G., and Khanna, P. K.: Change in soil carbon following afforestation, Forest Ecol. Manag., 168, 241–257, https://doi.org/10.1016/S0378-1127(01)00740-X, 2002.
Piñeiro, D. E.: Land grabbing: concentration and “foreignisation” of land in Uruguay, Can. J. Dev. Stud., 33, 471–489, https://doi.org/10.1080/02255189.2012.746216, 2012.
R Core Team: R: A Language and Environment for Statistical Computing, R Foundation for Statistical Computing, Vienna, Austria, https://www.R-project.org/, last access: 2 March 2021.
Ramírez, L. R. and Säumel, I.: Beyond the boundaries: Do spatio-temporal trajectories of land-use change and cross boundary effects shape the diversity of woody species in Uruguayan native forests?, Agr. Ecosyst. Environ., 323, 107646, https://doi.org/10.1016/j.agee.2021.107646, 2022.
Richter, D. D.: Game Changer in Soil Science. The Anthropocene in soil science and pedology, J. Plant Nutr. Soil Sci., 183, 5–11, https://doi.org/10.1002/jpln.201900320, 2020.
Ritchie, H. and Roser, M.: Land Use, Our World in Data, https://ourworldindata.org/land-use (last access: 6 May 2021), 2013.
Roca, N.: Heavy metal background levels in rural soils: a case study in Pampean soils (Argentina), Ciencia del Suelo, 33, 283–292, http://www.scielo.org.ar/pdf/cds/v33n2/v33n2a12.pdf (last access: 15 May 2021), 2015.
Sadzawka, A., Carrasco, R. M. A., Grez, Z. R., Mora, G. M, Flores, P. H., and Neaman, A.: Métodos de análisis recomendados para los suelos de Chile, Santiago, Chile, Serie Actas – Instituto de Investigaciones Agropecuarias, https://hdl.handle.net/20.500.14001/8541 (last access: 22 October 2020), 2006.
Sandoval López, D. M., Arturi, M. F., Goya, J. F., Pérez, C. A., and Frangi, J. L.: Eucalyptus grandis plantations: effects of management on soil carbon, nutrient contents and yields, J. Forest Res., 31, 601–611, https://doi.org/10.1007/s11676-018-0850-z, 2020.
Soil Survey Staff: Soil taxonomy: A basic system of soil classification for making and interpreting soil surveys, 2nd Edn., https://www.nrcs.usda.gov/Internet/FSE_DOCUMENTS/nrcs142p2_051232.pdf (last access: 15 October 2020), 1999.
Veldman, J. W., Overbeck, G. E., Negreiros, D., Mahy, G., Le Stradic, S., Fernandes, G. W., Durigan, G., Buisson, E., Putz, F. E., and Bond, W. J.: Where Tree Planting and Forest Expansion are Bad for Biodiversity and Ecosystem Services, BioScience, 65, 1011–1018, https://doi.org/10.1093/biosci/biv118, 2015.
Waters, C. N., Zalasiewicz, J., Summerhayes, C., Barnosky, A. D., Poirier, C., Ga uszka, A., Cearreta, A., Edgeworth, M., Ellis, E. C., Ellis, M., Jeandel, C., Leinfelder, R., McNeill, J. R., Richter, D. d., Steffen, W., Syvitski, J., Vidas, D., Wagreich, M., Williams, M., Zhisheng, A., Grinevald, J., Odada, E., Oreskes, N., and Wolfe, A. P.: The Anthropocene is functionally and stratigraphically distinct from the Holocene, Science, 351, aad2622, https://doi.org/10.1126/science.aad2622, 2016.
Wingeyer, A. B., Amado, T. J. C., Peìrez-Bidegain, M., Studdert, G. A., Perdomo Varela, C. H., Garcia, F. O., and Karlen, D. L.: Soil Quality Impacts of Current South American Agricultural Practices, Sustainability, 7, 2213–2242, 2015.
Wu, R., Alvareda, E., Polya, D., Blanco, G., and Gamazo, P.: Distribution of Groundwater Arsenic in Uruguay Using Hybrid Machine Learning and Expert System Approaches, Water, 13, 527, https://doi.org/10.3390/w13040527, 2021.
Zagal, E. and Sadzawka, A.: Protocolo de métodos de análisis para suelos y lodos, https://www.sag.cl/sites/default/files/METODOS_LODOS_SUELOS.pdf (last access: 14 October 2020), 2007.
Zurbriggen, C., González-Lago, M., Baraibar, M., Baethgen, W., Mazzeo, N., and Sierra, M.: Experimentation in the Design of Public Policies: The Uruguayan Soils Conservation Plans, Iberoamericana – Nordic Journal of Latin American and Caribbean Studies, 49, 52–62, https://doi.org/10.16993/iberoamericana.459, 2020.