the Creative Commons Attribution 4.0 License.
the Creative Commons Attribution 4.0 License.
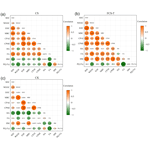
Effects of returning corn straw and fermented corn straw to fields on the soil organic carbon pools and humus composition
Yifeng Zhang
Sen Dou
Batande Sinovuyo Ndzelu
Rui Ma
Dandan Zhang
Xiaowei Zhang
Shufen Ye
Hongrui Wang
In our previous studies, we filtered out fungus (Trichoderma reesei) to have the best ability to transform corn straw into a humic-acid-like substance through laboratory incubation experiments. In order to further verify our former findings, we set up a 360 d field experiment that included three treatments applied under equal carbon (C) mass: (i) corn straw returned to the field (CS), (ii) fermented corn straw treated with Trichoderma reesei returned to the field (FCS-T), and (iii) blank control treatment (CK). Soil organic carbon (SOC), soil labile organic C components, soil humus composition, and the management levels of SOC pools under the three treatments were analyzed and compared. The results showed that the SOC content of CS and FCS-T treatments increased by 12.71 % and 18.81 %, respectively, compared with CK at 360 d. The humic acid carbon (HA-C) content of the FCS-T treatment was 0.77 g kg−1 higher than in the CS treatment. Applying FCS-T appeared to promote a significant increase in SOC content, carbon pool activity index, and carbon pool management index through the accumulation of HA-C, humin carbon, and easily oxidizable organic carbon. Applying fermented corn straw treated with Trichoderma reesei (FCS-T) is more valuable and conducive to increasing soil easily oxidizable organic C (EOC) and humus C content than direct application of corn straw.
- Article
(5278 KB) - Full-text XML
- BibTeX
- EndNote
Recycling and returning crop residues as soil amendments is an important prospect for increasing soil organic carbon (SOC) content and crop yield (Villamil et al., 2015) and managing crop straw residues. However, the decomposition process is slow when crop residues are directly applied to the soil (Zhang et al., 2019), and it is still not fully known how crop residues are transformed into stable SOC when applied to the soil (Cotrufo et al., 2013; Lehmann and Kleber, 2015; Zhang et al., 2015a). Information about the decomposition and stability of carbon (C) is needed for long-term soil C sequestration (Cotrufo et al., 2013; Ndzelu et al., 2020a). Contrary to direct crop residue application and conventional composting methods, pre-treatment of crop residues with microbial inoculants is an effective method for reusing crop residues as eco-friendly amendments to improve soil fertility and increase soil organic matter (SOM; Bhattacharjya et al., 2021; Organo et al., 2022). This strategy accelerates crop residue degradation and humification (Vargas-Garcia et al., 2006; Ahmed et al., 2019; Nigussie et al., 2021; Sajid et al., 2022) by significantly halving the time needed for the compost to reach maturity (Organo et al., 2022). The microbial-inoculant-based fermentation product contains both labile organic substances from the degraded portion and relatively stable humic-like substances (Huang et al., 2008). This favors the accumulation and production of SOM when applied to the soil (Vargas-Garcia et al., 2006; Ahmed et al., 2019).
The labile organic C components are sensitive and respond promptly to changes in soil management practices (Blair et al., 1995; Xu et al., 2011). Hence, the labile organic C fractions such as water-extractable organic carbon (WEOC), easily oxidizable organic carbon (EOC), and microbial biomass carbon (MBC) are effectively used to detect small changes in soil quality (Chen et al., 2009; Sainepo et al., 2018). Chen et al. (2017) and Ma et al. (2021) reported a significant increase in MBC and WEOC contents after crop straw residues were returned to the soil. In another study, Ndzelu et al. (2020b) also found that 5 years of corn straw application increased soil EOC, WEOC, and MBC contents by 34.09 %, 41.38 %, and 49.09 % in the 0–20 cm depth, respectively. Therefore, assessing labile SOC fractions after crop straw applications may provide information about the formation of SOC (Chen et al., 2009; Huang et al., 2018; Liu et al., 2019; Ma et al., 2021). The carbon pool management index incorporates the carbon pool index and carbon pool activity index and is an important and sensitive index widely used to determine changes in SOC and C sequestration in the soil (Blair et al., 1995; Duval et al., 2019). A high carbon pool management index indicates that soil management practices have a greater potential to promote soil C sequestration (Duval et al., 2019).
The humic substance is the most stable fraction of SOM and contributes the largest proportion to the total SOC (Olk et al., 2019; Dou et al., 2020). As a result, studying changes in soil humus components and the labile organic C fractions after corn straw application could inform about the formation and stabilization of SOC during crop residue decay. Over the years, extensive studies have been conducted to investigate the effects of crop residues on SOM and its pools (Atiyeh et al., 2002; Romero et al., 2007; Zhang et al., 2015a; Ng et al., 2016; Yang et al., 2020). However, there are still conflicting reports, and there is no consensus about the effects of crop residue application on the formation and composition of SOM. For instance, recent studies have found that corn straw application significantly increases soil humus content and enriches soil humic acid structure with aromatic compounds (Fan et al., 2018; Zhang et al., 2019). In contrast, other studies reported increased aliphatic compounds in soils amended with corn straw (Yang et al., 2020; Ndzelu et al., 2020a). These diverging reports indicate that the magnitude and the influence of corn straw residues on SOM composition are unclear and site-specific.
Trichoderma-mediated straw fermentation is gaining attention as a soil amendment and nutrient source (Gaind and Nain, 2007; Siddiquee et al., 2017) because of the role Trichoderma-mediated straw plays in improving crop yield (Islam et al., 2014), promoting plant development, and alleviating biotic and abiotic stresses in crops (Sarmistha et al., 2021). In our previous studies (Yang et al., 2019; Zhang et al., 2020, 2021), we observed in laboratory incubation experiments that the Trichoderma reesei (T. reesei) had the best ability to form humic-acid-like substances during corn straw decomposition when compared with other fungi (Phanerochaete chrysosporium and Trichoderma harzianum). However, there is limited knowledge on the potential effects of T. reesei fermented corn straw on SOM formation and accumulation. In particular, the dynamic change process of different SOC fractions has not been sufficiently reported after soils are amended with T. reesei fermented corn straw. In this study, we quantified differences in soil organic carbon pools and humus composition between treatments. The purpose of this study was to verify whether the application of fermented corn straw treated with T. reesei is more effective in forming a relatively stable SOC fraction in a field experiment compared to the direct application of corn straw. We hypothesized that (1) application of fermented corn straw treated with T. reesei (FCS-T) will be the most efficient in increasing soil humus content and soil C storage, due to the increase in aromatic C compounds; (2) application of FCS-T may also increase soil labile organic C components (WEOC, EOC, and MBC); and (3) application of FCS-T may increase carbon pool management index level more than direct corn straw application.
2.1 Site description
A 360 d field experiment was conducted in a corn monocropping experimental field located at Jilin Agricultural University in Northeast China ( N, E). Since 2005, monocropping of corn (Zea mays L.) has been the main cropping system in the region. The area is in a semi-humid region and receives a mean annual rainfall of 618 mm, with the highest precipitation occurring in the months of July and August. Soils in the study area are classified as Argiudolls according to the United States Department of Agricultural Soil Taxonomy (Soil Survey Staff, 2014). The basic soil characteristics are presented in Table 1.
2.2 Preparation and description of corn straw and fermentation of corn straw
Corn straw was collected from the adjacent cropland of corn (Zea mays L.) located at Jilin Agricultural University in Northeast China ( N, E). The corn cultivar of Zhongjin 368 type (Beijing Golden Grain Seed Co., Ltd.) was planted at the end of April 2018 and harvested in early October 2018. After harvest, the whole corn straw residue was cut at the bottom and air-dried and thereafter shredded into 0.5 cm segments. A portion of the shredded corn straw was regarded as CS material.
The fermentation of corn straw was prepared by the fungal strains (Trichoderma reesei (T. reesei) MCG77), which were purchased from the American Type Culture Collection. The strains of fungi were inoculated on a medium containing 30 mL of potato dextrose agar and placed in an incubator at 28 ∘C for 72 h to obtain mature microbial spores (mycelium). This process was carried in a BIOTECH-30SS solid fermentation tank (Shanghai Baoxing Biological Engineering Equipment Co., Ltd). A KQ-C-type automatic steam generator (Shanghai Fengxian Xiexinji Power Plant) was used to generate steam for sterilization, and 2 kg of air-dried corn straw (particle size =0.5 cm) was sterilized in a solid fermenter. The sterilization process was conditioned for 25 min at 121 ∘C. After sterilization, the T. reesei liquid containing the spore mycelia (0.8 L) and a mineral salt solution (5 L) was mixed with sterilized corn straw. The spore solution and a mineral salt solution (pH =5) used were prepared similarly as described by Zhang et al. (2020), and the ratio was adjusted to 25:1 using a mineral salt nutrient solution. The mineral salt nutrient solution (g L−1) was prepared as a mixture of 28 g KH2PO4, 9.6 g (NH4)2SO4, 4.2 g MgSO4, 4.2 g CoCl2, 2.2 g (NH2)2CO, 0.07 g FeSO4•7H2O, 0.028 g CaCl2, 0.021 g MnSO4, 0.019 g ZnSO4, and pH =5. The fermentation process lasted 90 d and was carried out at 30 ∘C, 60 % humidity, and 6.0 rpm. The final fermented product after 90 d was designated as fermented corn straw treated with T. reesei (FCS-T) material. The basic elemental properties of the CS and FCS-T materials are presented in Table 2 and were determined with an element analyzer (Vario-EL-III Hanau, Germany).
2.3 Field procedures and sampling
2.3.1 Experimental layout: field plot settings and specifications
The field experiment was set up to have nine plots and three treatments, namely CS, FCS-T, and CK (as a control), applied under equal C mass. Each treatment was replicated three times and arranged in a completely randomized design. The size of each plot was 0.6 m ×0.6 m. The specific scheme of soil treatment is shown in Fig. 1.
The CS return treatment was prepared by mixing 360 g of corn straw residues (equivalent to 1 kg m−2) in the 0–20 cm surface soil layer. Exactly 5.975 g CH4N2O was added to adjust the ratio to 25:1 (suitable for soil microbial growth; Chapin et al., 2011). After that, the base fertilizer (17.68 g of CH4N2O and 7.92 g of KH2PO4) was applied to the 0–20 cm soil layer.
Preparation of FCS-T treatment was done by mixing 428 g (the same amount of C mass as the C of the CS material) of fermented corn straw treated with T. reesei material (equivalent to 1.189 kg m−2) in the topsoil layer of 0–20 cm. The same amount of base fertilizer as in the CS treatment (17.68 g of CH4N2O and 7.92 g of KH2PO4) was applied in the 0–20 cm depth of the FCS-T plots.
The blank control (CK) treatment was prepared by only mixing 17.68 g of CH4N2O and 7.92 g of KH2PO4 fertilizer in the 0–20 cm soil depth.
2.3.2 Soil sampling and analysis
Five topsoil samples (0–20 cm) were collected from each plot at 0, 30, 60, 90, 180, and 360 d using a stainless-steel soil auger (5 cm in diameter). For each soil sampling day, all visible corn straw materials in CS and FCS-T soils were picked out with tweezers and returned to their respective plots. The collected fresh soil was immediately divided into two sub-samples and passed through a 2 mm sieve. One subsample was then placed in a refrigerator (4 ∘C) to later analyze MBC in soil. The remaining subsample was air-dried to determine SOC, EOC, WEOC content, and humus composition.
2.4 Analytical methods
2.4.1 Labile soil organic carbon fractions
The SOC content was determined by the potassium dichromate oxidation method (Nelson and Sommers, 1982). The WEOC content was obtained by successively extracting 5 g of air-dried soil samples with distilled water in a 1:6 ratio of soil to water. The soil-solution mixture was shaken on a reciprocal shaker at 25 ∘C for 60 min and then centrifuged at 4500 rpm for 20 min. The solution was filtered through a 0.45 µm filter membrane (Changtingny et al., 2010). The EOC content was determined using the KMnO4 (333 mM) oxidation procedure (Lefroy et al., 1993). Fresh soil equivalent to 10 g of oven-dried soil was fumigated with CHCl3 for 24 h, and the other 10 g of soil was not fumigated. Both fumigated and unfumigated soils were then extracted with 0.5 mol L−1 K2SO4. The MBC content was estimated from the increase in organic C in the 0.5 mol L−1 K2SO4 extracts of CHCl3 fumigated soils as described by Vance et al. (1987). The soil WEOC and MBC contents were determined by a TOC analyzer (Shimadzu TOC-VCPH, Japan). MBC was calculated as below Eq. (1):
where Fc is the difference between the amount of CO2 released by fumigated and unfumigated soil (control) during the cultivation period, and kc is the conversion coefficient.
The carbon-available ratio (CAR) of labile organic C contents (WEOC, EOC, and MBC) was calculated as follows:
The carbon pool index (CPI), carbon pool activity (CPA), carbon pool activity index (CPAI), and carbon management pool index (CPMI) were calculated, according to Blair et al. (1995) and Jiang et al. (2021), as follows:
where SOCTreatment represents the SOC content (g kg−1) in soil of a given treatment (CS, FCS-T, or CK), and represents the SOC content (g kg−1) in soil of CK at 0 d.
NLOC represents the non-labile organic C content (g kg−1), which is the difference between the SOC content and EOC content.
where CPATreatment represents the CPA in soil of a given treatment (CS, FCS-T, or CK), and represents the CPA in soil of CK at 0 d.
2.4.2 Humus composition
Humus composition was sequentially analyzed following the International Humic Substances Society procedure (Kumada, 1987) described in detail by Dou (2010). Briefly, 5 g of air-dried soil was extracted with a 30 mL mixture of 0.1 M alkali solution (NaOH + Na4P2O7) under permanent shaking at 70 ∘C for 1 h and centrifuged. The remaining soil residue was humin, and the mixture, which is humus extract, was acidified with 0.5 M sulfuric acid to separate humic acid and fulvic acid. The carbon contents of the humus extract (HE-C), humic acid (HA-C), and humin (HM-C) were determined. Then the C content of fulvic acid (FA-C) was calculated as the difference between HE-C and HA-C. The humification degree (PQ) was calculated as the HA-C / HE-C ratio (Sugahara and Inoko, 1981).
2.5 Statistical analysis
Microsoft Office Excel 2017 was used for data processing, and the statistical analysis was performed by SPSS Statistics 22.0 (IBM Statistics 21.0). Significant differences among treatment means were evaluated using the least significant difference test with Tukey's adjustment at P<0.05. Principal component analysis (PCA) was performed with Minitab 18 software (Pennsylvania, USA) to check for similarities between treatments. The graphs were compiled using Origin 2019 software (OriginLab Corporation).
3.1 Changes in SOC contents
At 0 d, the SOC content did not differ significantly between the three treatments but differed significantly from 30 to 360 d among the three treatments (Fig. 2). The FCS-T treatment showed significantly higher SOC content than all treatments, whereas the CK had significantly lower SOC content throughout the study period. The CS and FCS-T treatments showed the most significant increase in SOC content with the increase in the duration of the study. In contrast, SOC content in the CK treatment did not change significantly throughout the 360 d period. At the 360 d, the SOC content of CS and FCS-T was 12.71 % and 18.81 % higher than that of CK, respectively.
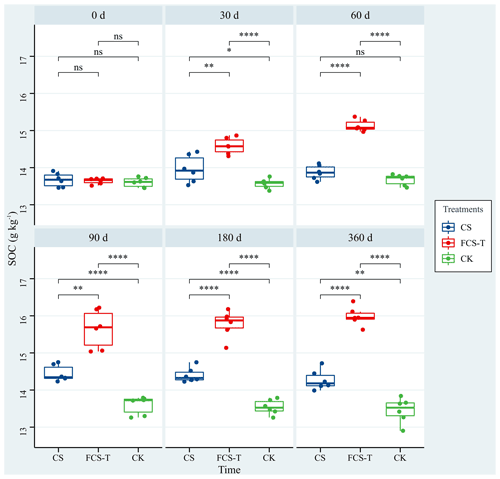
Figure 2Soil organic carbon content of the three different treatments during the 360 d experimental period. The upper, middle, and lower horizontal lines of the box represent the upper quartile, the median, and the lower quartile, respectively. The values represented by the upper- and lower-line segments refer to the maximum and minimum values of the data, and the points outside the box represent outliers. The symbol on the figure indicates the P value between two variables. The number of “*” indicates the degree of significance. For example, “*” means P<0.05, “” means P<0.01, “” means P<0.001, `” means P<0.0001, and “ns” means no significance. CS, corn straw returned to the field; FCS-T, fermented corn straw treated with T. reesei returned to the field; CK, blank control treatment.
3.2 Changes in soil labile organic carbon fractions and carbon-available ratios
In the 360 d field experiment, the WEOC, EOC, and MBC contents of CK, CS, and FCS-T treatments showed a similar changing trend (Fig. 3). The content of these attributes increased from 0 to 90 d and then gradually decreased to the 360th day in the CS and FCS-T treatments. Water-extractable organic C, EOC, and MBC contents of CS and FCS-T treatments were highest at 90 d. The WEOC, EOC, and MBC contents of CK appeared to decrease slightly with the duration of the experiment. Comparing all treatments, the contents of WEOC, EOC, and MBC did not differ significantly at 0, 30, 180, and 360 d between CS and FCS-T treatments.
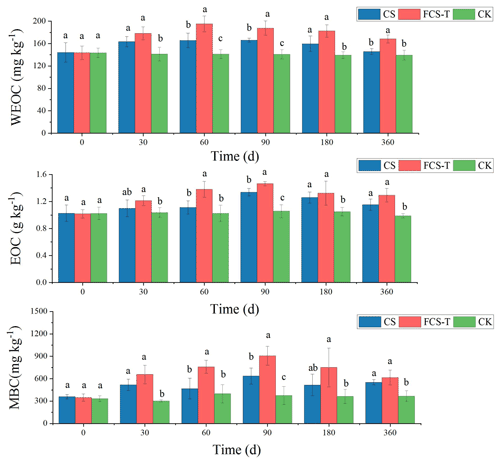
Figure 3Effects of corn straw returned (CS), fermented corn straw treated with T. reesei returned (FCS-T), and non-straw amended soil (CK) on soil labile organic carbon (WEOC, EOC, and MBC) concentrations in the 0–20 cm soil depth. Each bar represents the mean ± standard deviation in the figure (n=3). Different lowercase letters within the same time indicate significant differences among different treatments at P<0.05 level.
In terms of WEOC, the carbon-available ratios of CS (1.19 %) and FCS-T (1.29 %) treatments were highest at 60 d and lowest at 0 d (Table 3). Regarding EOC, the carbon-available ratios of CS (9.25 %) and FCS-T (9.34 %) treatments were significantly higher at 90 d. With respect to MBC, the carbon-available ratio of FCS-T (5.80 %) treatment was also higher at 90 d, and that of CS (2.92 %) treatment was significantly higher at 60 d. Irrespective of sampling time, the carbon-available ratios of WEOC, EOC, and MBC were always significantly higher under FCS-T and CS treatments compared with CK. These parameters did not always differ significantly between the CS and FCS-T treatments.
Table 3The carbon-available ratio (CAR) of water-extractable organic carbon (WEOC), easily oxidizable organic carbon (EOC), and microbial biomass carbon (MBC) under different treatments in the 0–360 d period. Note that values are means that do not share the same letter for a given parameter, and time (d) of experiments is significantly different (P<0.05). CS, corn straw returned to the field; FCS-T, fermented corn straw treated with T. reesei returned to the field; CK, blank control treatment.
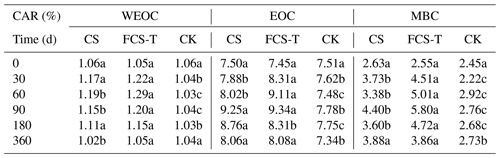
3.3 Soil carbon pool management index
The soil carbon pool management index was computed at the end of the experiment (i.e., day 360). At the 360th day, the CS and FCS-T treatments significantly increased the carbon pool management index and carbon pool activity index compared with the CK treatment. However, the carbon pool activity index of CS and FCS-T treatments did not differ significantly (Table 4). Applying CS and FCS-T significantly increased the carbon pool management index compared with CK, increasing the CPMI by 17.3 % and 31.7 %, respectively.
Table 4The carbon management indices under different treatments during the 360 d period. Note that mean values ± SE that do not share the same letter within a column of indexes are significantly different (P<0.05). CS, corn straw returned to the field; FCS-T, fermented corn straw treated with T. reesei returned to the field; CK, blank control treatment. CPI, carbon pool index; CPA, carbon pool activity index; CPAI, carbon pool activity index; CPMI, carbon pool management index.
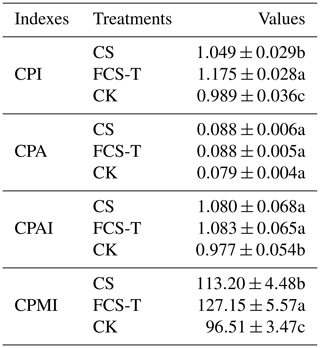
Table 5Changes of relative content of each humic substance component and the PQ values in different treatments during the 0–360 d period. Note that values are means ± SE. Means that do not share the same letter within a column of a given parameter and time (d) of the experiment are significantly different (P<0.05). HA, humic acid; FA, fulvic acid; HM, humin; CS, corn straw returned to the field; FCS-T, fermented corn straw treated with T. reesei returned to the field; CK, blank control treatment.
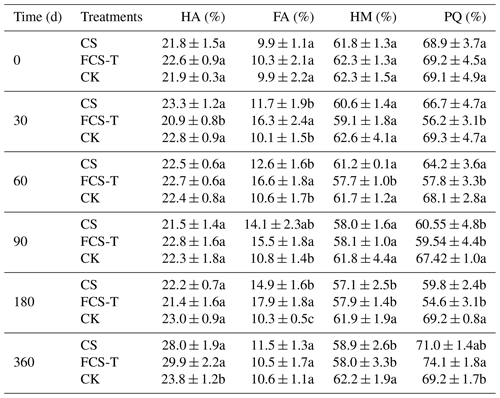
3.4 Humus composition and C content in soil under different treatments
At 0 d of the experiment, there was no significant difference in the relative content (Table 5) and composition of humus C among the three treatments (Fig. 4). With the application of CS and FCS-T, the HE-C and HM-C contents in the soil increased with the duration of the experiment. Compared with CK, the CS and FCS-T treatments increased HE-C content in the soil. At 360 d, the HE-C content of the FCS-T treatment was significantly higher than that of the CS treatment, and the HE-C of the FCS-T and CS treatments increased by 1.99 and 1.31 g kg−1, respectively, when compared with that at 0 d. The HM-C content of the FCS-T treatment increased significantly when compared with other treatments throughout the experiment, with a cumulative increase of 0.79 g kg−1 at 360 d (Fig. 4). Throughout the experiment, no significant difference was observed between CS and CK treatments with respect to HM-C content.
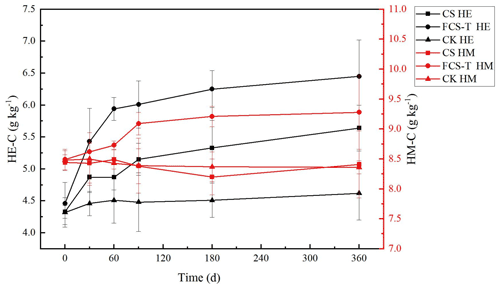
Figure 4The carbon content of humus extracted (HE-C) and humin (HM-C) of the three different treatments during the 360 d period. The error bar shows the standard deviations of triplicate averages. CS, corn straw returned to the field; FCS-T, fermented corn straw treated with T. reesei returned to the field; CK, blank control treatment.
Compared with CK, the application of CS and FCS-T increased the FA-C content in the soil throughout the experiment (Fig. 5). The highest FA-C content in the CS and FCS-T treatments was measured at 180 d, and the lowest FA-C content was recorded at 0 d. The content of HA-C under CS and FCS-T treatments increased with the duration of the experiment. The highest HA-C content in the CS and FCS-T treatments was measured at 360 d, and the lowest HA-C content was recorded at 0 d. The content of HA-C in the FCS-T treatment at 360 d was 0.77 g kg−1 higher than in the CS treatment.
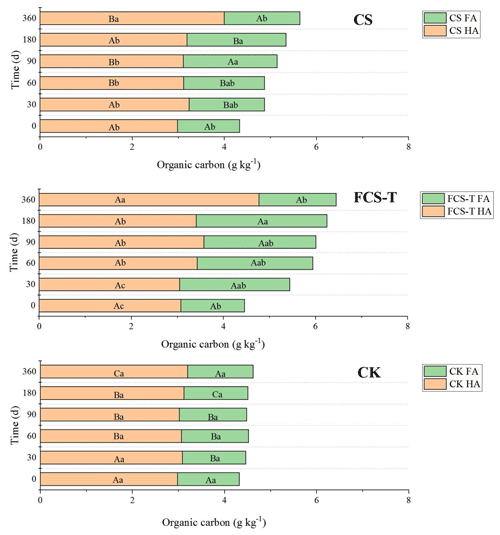
Figure 5The carbon contents of humic acid (HA) and fulvic (FA) isolated from different treatments. Each bar represents the mean of HA and FA in the figure (n=3). Different uppercase letters mean significant difference (P<0.05) for either HA or FA among the treatments in a particular time (d) of the experiment. Different lowercase letters mean significant difference (P<0.05) for either HA or FA among different experimental times (d) for a given treatment.
3.5 Multivariate analysis
The relationship between SOC parameters and humus components, shown according to PCA (Fig. 6), was clearly confirmed by Pearson's correlation analysis (Fig. 7). Figure 6 indicated that under all the treatments, the HA-C, HM-C, and EOC contents exhibited significant correlations with SOC content, carbon pool activity index, and carbon pool management index, whereas WEOC and MBC contents were significantly correlated with the FA-C content. The PCA clearly separated the three treatments, implying that each treatment had a distinct influence on SOC content, CPMI, and humus component characteristics. The correlation between SOC and carbon pool management index was more pronounced under the CS and FCS-T treatments. The correlation between MBC and SOC was stronger under the CS treatment, and the correlations between WEOC, MBC, and FA-C were more pronounced under the FCS-T treatment.
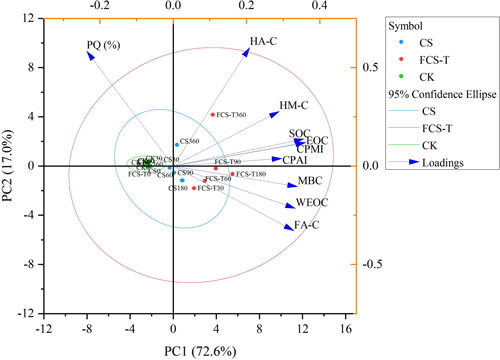
Figure 6Principal component analysis (PCA) of soil organic carbon parameters and humus components affected by different treatments during 360 d of the experiment. PC, principal component; SOC, soil organic carbon; CPMI, carbon pool management index; CPAI, carbon pool activity index; WEOC, water-extractable organic carbon; EOC, easily oxidizable organic carbon; MBC, microbial biomass carbon; HA, humic acid; FA, fulvic acid; HM, humin; PQ, humification degree; CS, corn straw returned to the field; FCS-T, fermented corn straw treated with T. reesei returned to the field; CK, blank control treatment.
4.1 Effects of different treatments on SOC, soil labile organic carbon fractions, and humus fractions
A large number of studies have shown that the application of organic materials is beneficial to the accrual of SOC (Ros et al., 2006; Zhang et al., 2015b) and the distribution of labile organic C components (Blair, 2000; Chen et al., 2009; Sainepo et al., 2018). This is consistent with the results of our study, which showed that the application of CS and FCS-T increased SOC content (Fig. 2), MBC, WEOC, and EOC contents (Fig. 3). Although applied under equal C mass input, the FCS-T treatment sequesters more organic C in the soil than the CS treatment. This may be because the FCS-T used in the present study was produced by fermentation with T. reesei and had a lower ratio (Table 2). Studies show that part of the organic matter input is converted into CO2 and other substances during fermentation. The remaining residue is converted into the stable organic matter like humic substances (Atiyeh et al., 2002; Romero et al., 2007). The closer the substrate's ratio is to the microorganisms' ratio, the more significant the fraction of substrate C that remains in the soil (Hessen et al., 2004). Furthermore, according to Sprunger et al. (2019), a low ratio of organic residues promotes the accumulation of SOM, whereas organic inputs applied to the soil with a large ratio (such as the CS treatment in the case of our study) may lose more C in turnover compared with organic amendments with a small ratio (Dannehl et al., 2017). The ratio of organic amendments and the C fate in the soil had a negative connection (Dannehl et al., 2017), which is consistent with the results of our study.
Our results further showed that after FCS-T and CS application, the concentrations of WEOC, EOC, and MBC in the soil increased at the initial stages of the experiments (i.e., 0–90 d) and then gradually decreased towards the end of the experiment (Fig. 3). In contrast, the HE-C and HM-C contents appeared to increase with the duration of the experiment, with a greater increase reported in the FCS-T treatment (Figs. 4 and 5). This result is consistent with the findings of Guan et al. (2015). The reason for this phenomenon may be because the WEOC and EOC are easily oxidizable and are the first organic compounds utilized by soil microorganisms (Haynes et al., 2005). Corn straw contains aromatic C compounds (Roldán et al., 2011; Zhang et al., 2020), which are more difficult to decompose and tend to accumulate as humic substances (Kuzyakov et al., 2009; Pan et al., 2016; Dou et al., 2020).
Compared with other treatments, the FCS-T treatment appeared to have a significantly higher WEOC content, but the EOC content did not consistently differ significantly between the FCS-T and CS treatments during the duration of the experiment (Fig. 3). Ma et al. (2021) reported similar findings where the WEOC content in the soil amended with barley treated with microbial inoculant was significantly higher than that of barley residue without microbial inoculant, but the EOC content differed seldom. The higher EOC content in FCS-T treatment than in CS treatment (Table 3) suggests that organic matter after microbial treatment is likely converted into EOC. During the entire duration of the present experiment, the MBC content of FCS-T treatment was also higher than that of CS treatment but not always significant, which is consistent with Ng et al. (2016). This may be because crop residues treated with microbial inoculants are easily assimilated by soil microorganisms (Gaind and Nain, 2007; Vargas-Garcia et al., 2006; Pan et al., 2016), thereby promoting the sequestration of organic C in organic materials.
The WEOC and EOC of the soil largely depend on the SOC content (Guan et al., 2018). This was also confirmed by the results from the present study, which showed SOC content to be positively correlated with WEOC, EOC, and MBC contents (Fig. 7). This means the WEOC, EOC, and MBC can be used as the best proxies to detect changes in SOC content, since these fractions respond promptly to changes in soil management practices. In the present study, the correlation of SOC content with MBC and EOC was more pronounced under FCS-T treatment than CS and CK treatments. This may be likely due to differences in the chemical composition of these treatments. Vanlauwe et al. (2005) and Mandal et al. (2007) found that changes in soil C are mainly influenced by the chemical composition of the applied organic matter.
Compared with other treatments, the FCS-T treatment significantly increased the content of HA-C and FA-C throughout the 360 d period (Fig. 5). This is because the FCS-T material contains relatively higher alkyl, aromatic C contents, and humic-like substances (see Zhang et al., 2021), compounds resistant to direct microbial degradation. Accumulating microbial-resistant compounds promotes the formation of soil humic substances during the humification process (Huang et al., 2008; Roldán et al., 2011). During the humification process, parts of the humic-like substances in the FCS-T treatment gradually formed new FA and HA in the soil, possibly through microbial ex vivo modification and in vivo turnover (Liang et al., 2017). Zhang et al. (2019) further found that fermented corn straw was more conducive to the increase of HA-C, and the CS treatment was more conducive to the increase of FA-C when applied to the soil. In other studies, Gaind and Mathur (2001) and Gaind and Nain (2007) reported a significant increase in humus C content in the soil amended with wheat straw compost treated with T. reesei. Analyzing the changes of humic substances components in different periods in our study, we found that the FCS-T treatment significantly and continuously increased FA relative content for up to 180 d, while the increase in the HA relative content lasted up to 360 d (Table 5). Therefore, the application of FCS-T materials is more conducive to increasing humus C content and HA relative content (including more aromatic C compounds), which is important for the long-term storage of SOC.
4.2 Relationships between SOC, soil labile organic carbon fractions, humus components, and CPMI
The results of this study showed that the increase in SOC content was mainly due to the increase in EOC, HA-C, and HM-C contents, rather than the accumulation of WEOC and MBC. The possible explanation is that WEOC and MBC are more easily utilized by soil microorganisms, and their ratio in SOC is much lower (Blair, 2000; Haynes, 2005). The carbon pool management index is a comprehensive index to evaluate SOC variation rates in response to soil management practices. For instance, a high carbon pool management index indicates that the soil management practices have a stronger potential to promote soil C sequestration (Blair et al., 1995). In the present study, the FCS-T treatment showed a significantly higher carbon pool management index and carbon pool index than CS and CK treatments (Table 4). This suggests that the FCS-T treatment was more conducive to the accumulation of organic C in the soil. This result may be due to the fact that soil C accumulation is mainly driven by increased plant residue input, which increases SOC content. The MBC was positively correlated with FA-C and SOC (Figs. 6 and 7), providing evidence that the activity of microorganisms affects the accumulation of FA in the soil, thereby promoting the increase of SOC.
In this 360 d field experiment, we applied corn straw (CS) and fermented corn straw treated with Trichoderma reesei (FCS-T) under equal C input and a blank control treatment (CK) for comparison. The following conclusions were drawn.
-
The change of SOC content mainly depends on the C content of the stable soil components, i.e., aromatic compounds (HA-C and HM-C). The FCS-T material has a lower ratio, higher alkyl and aromatic C content, and humic-like substances. When the FCS-T material is applied to the soil, it is more advantageous in promoting the soil humification process and increasing soil HA-C and HM-C content. Compared with direct corn straw application (i.e., CS treatment), the FCS-T treatment increased the SOC content by 1.715 g kg−1 on the 360th day and increased the PQ value to 74.1 %. The application of FCS-T material with a lower ratio sequestered more SOC than the application of CS, which supported the idea that the ratio in the organic amendments is negatively correlated with SOC content.
-
The application of FCS-T significantly improves the release of soil labile carbon components. In particular, the WEOC content could maintain a high level for a long time, while the EOC and MBC contents of FCS-T treatment increased significantly more than CS treatment on the 60th and 90th days.
-
Compared to CS treatment, the FCS-T treatment significantly improved the level of carbon pool management to 13.95, primarily by promoting the simultaneous increase in the contents of EOC and stable organic carbon components (HA-C and HM-C).
The results confirmed our initial hypothesis that the application of FCS-T has a greater potential to increase soil carbon sequestration compared with direct application of CS. As a method of returning straw residues to the field, the application of FCS-T is a practice worthy of further exploration.
The data generated in this study are available from the corresponding authors upon reasonable request.
YZ designed the methodology of the research and wrote the original manuscript. SD provided conceptualization and funding acquisition. BSN polished and edited the language of the manuscript. YZ, RM, SY, DZ, and HW performed trials, conducted fieldwork, and did data curation. SD, BSN, and XZ provided comments and edited the manuscript. All authors read and approved the final paper.
Publisher's note: Copernicus Publications remains neutral with regard to jurisdictional claims in published maps and institutional affiliations.
The study was supported by the National Natural Science Foundation of China (Innovative Research Group Project (grant no. 42077022)) and the Key Research and Development Program of Jilin Province (grant no. 20200402098NC).
This paper was edited by Luisella Celi and reviewed by two anonymous referees.
Ahmed, A. A. Q., Odelade, K. A., and Babalola, O. O.: Microbial Inoculants for Improving Carbon Sequestration in Agroecosystems to Mitigate Climate Change, in: Handbook of Climate Change Resilience, edited by: Leal Filho, W., Springer, Cham, 381–401, https://doi.org/10.1007/978-3-319-93336-8_119, 2019.
Atiyeh, R. M., Lee, S., Edwards, C. A., Arancon, N. Q., and Metzger, J. D.: The influence of humic acids derived from earthworm-processed organic wastes on plant growth, Bioresour. Technol., 84, 7–14, https://doi.org/10.1016/s0960-8524(02)00017-2, 2002.
Bhattacharjya, S., Sahu, A., Phalke, D. H. Manna, M. C., Thakur, J. K., Mandal, A., Tripathi, A. K., Sheoran, P., Choudhary, M., Bhowmick, A., Rahman, M. M., Naidu, R., and Patra, A. K.: In situ decomposition of crop residues using lignocellulolytic microbial consortia: a viable alternative to residue burning, Environ. Sci. Pollut. Res., 28, 32416–32433, https://doi.org/10.1007/s11356-021-12611-8, 2021.
Blair, G., Lefroy, R., and Lisle, L.: Soil carbon fractions based on their degree of oxidation, and the development of a carbon management index for agricultural systems, Aust. J. Agric. Res., 46, 1459–1466, https://doi.org/10.1071/AR9951459,1995.
Blair, N.: Impact of cultivation and sugar-cane green trash management on carbon fractions and aggregate stability for a Chromic Luvisol in Queensland, Australia, Soil. Till. Res., 55, 183–191, https://doi.org/10.1016/s0167-1987(00)00113-6, 2000.
Changtingny, M. H., Curtin, D., Beare, M. H., and Greenfield, L. G.: Influence of Temperature on Water-Extractable Organic Matter and Ammonium Production in Mineral Soils, Soil. Sci. Soc. Am. J., 74, 517–524, https://doi.org/10.2136/sssaj2008.0347, 2010.
Chapin, F. S., Matson, P. A., and Vitousek, P. M.: Plant Nutrient Use, in: Principles of Terrestrial Ecosystem Ecology, Springer, New York, 229–258 https://doi.org/10.1007/978-1-4419-9504-9_8, 2011.
Chen, H. Q., Hou, R. X., Gong, Y. S., Li, H. W., Fan, M. S., and Kuzyakov, Y.: Effects of 11 years of conservation tillage on soil organic matter fractions in wheat monoculture in Loess Plateau of China, Soil. Till. Res., 106, 85–94, https://doi.org/10.1016/j.still.2009.09.009, 2009.
Chen, Z., Wang, H., Liu, X., Zhao, X., Lu, D., Zhou, J., and Li, C.: Changes in soil microbial community and organic carbon fractions under short-term straw return in a rice-wheat cropping system, Soil. Till. Res., 165, 121–127, https://doi.org/10.1016/j.still.2016.07.018, 2017.
Cotrufo, M. F., Wallenstein, M. D., Boot, C. M., Denef, K., and Paul, E.: The Microbial Efficiency-Matrix Stabilization (MEMS) framework integrates plant litter decomposition with soil organic matter stabilization: do labile plant inputs form stable soil organic matter?, Glob. Change Biol., 19, 988–995, https://doi.org/10.1111/gcb.12113, 2013.
Dannehl, T., Leithold, G., and Brock, C.: The effect of C:N ratios on the fate of carbon from straw and green manure in soil, Eur. J. Soil. Sci., 68, 988–998, https://doi.org/10.1111/ejss.12497, 2017.
Dou, S.: Soil organic matter, Science Press, Beijing, China, ISBN: 9787030260710, 2010 (in Chinese).
Dou, S., Shan, J., Song, X., Cao, R., Wu, M., Li, C., and Guan, S.: Are humic substances soil microbial residues or unique synthesized compounds? A perspective on their distinctiveness, Pedosphere, 30, 159–167, https://doi.org/10.1016/s1002-0160(20)60001-7, 2020.
Duval, M. E., Martinez, J. M., Galantini, J. A., and Aitkenhead, M.: Assessing soil quality indices based on soil organic carbon fractions in different long-term wheat systems under semiarid conditions, Soil. Use. Manag., 36, 71–82, https://doi.org/10.1111/sum.12532, 2019.
Fan, W., Wu, J., Li, J., and Hu, J.: Comparative effects of different maize straw returning modes on soil humus composition and humic acid structural characteristics in Northeast China, Chem. Ecol., 34, 355–370, https://doi.org/10.1080/02757540.2018.1437147, 2018.
Gaind, S. and Mathur, R. S.: Influence of Insitu incorporation of organic waste on chemical and biochemical properties of soil under rice–wheat cropping system, Ecol. Env. Cons., 7, 269–272, 2001.
Gaind, S. and Nain, L.: Chemical and biological properties of wheat soil in response to paddy straw incorporation and its biodegradation by fungal inoculants, Biodegradation, 18, 495–503, https://doi.org/10.1007/s10532-006-9082-6, 2007.
Guan, S., Dou, S., Chen, G., Wang, G., and Zhuang, J.: Isotopic characterization of sequestration and transformation of plant residue carbon in relation to soil aggregation dynamics, Appl. Soil. Ecol., 96, 18–24, https://doi.org/10.1016/j.apsoil.2015.07.004, 2015.
Guan, S., An, N., Zong, N., He, Y. T., Shi, P. L., Zhang, J. J., and He, N. P.: Climate warming impacts on soil organic carbon fractions and aggregate stability in a Tibetan alpine meadow, Soil. Biol. Biochem., 116, 224–236, https://doi.org/10.1007/s10532-006-9082-6, 2018.
Haynes, R. J.: Labile organic matter fractions as central components of the quality of agricultural soils: an overview, Adv. Agron., 85, 221–268, https://doi.org/10.1016/s0065-2113(04)85005-3, 2005.
Hessen, D. O., Ågren, G. I., Anderson, T. R., Elser, J. J., and de Ruiter, P. C.: Carbon sequestration in ecosystems: the role of stoichiometry, Ecology, 85, 1179–1192, http://www.jstor.org/stable/3450161 (last access: 15 September 2022), 2004.
Huang, H., Zeng, G., Tang, L., Yu, H., Xi, X., Chen, Z., and Huang, G.: Effect of biodelignification of rice straw on humification and humus quality by Phanerochaete chrysosporium and Streptomyces badius, Int. Biodeterio. Biodegra., 61, 331–336, https://doi.org/10.1016/j.ibiod.2007.06.014, 2008.
Huang, R., Tian, D., Liu, J., Lu, S., He, X., and Gao, M.: Responses of soil carbon pool and soil aggregates associated organic carbon to straw and straw-derived biochar addition in a dryland cropping mesocosm system, Agric. Ecosyst. Environ., 265, 576–586, https://doi.org/10.1016/j.agee.2018.07.013, 2018.
Islam, M. A., Mostafa, M. G., and Rahman, M. R.: Conversion of solid organic waste into compost using Trichoderma spp. and its application on some selected vegetables. Int. J. Environ. Waste. Manag., 14, 211–221, https://doi.org/10.1504/IJEWM.2014.064570, 2014.
Jiang, X., Xu, D., Rong, J., Ai, X., Ai, S., Su, X., Sheng, M., Yang, S., Zhang, J., and Ai, Y.: Landslide and aspect effects on artificial soil organic carbon fractions and the carbon pool management index on road-cut slopes in an alpine region, Catena, 199, 105094, https://doi.org/10.1016/j.catena.2020.105094, 2021.
Kumada, K.: Chemistry of Soil Organic Matter, Band 17 der Reihe Developments in Soil Science, Elsevier/Japan Scientific Societies Press, Amsterdam, 260 p., ISBN: 0-444-98936-6, 1987.
Kuzyakov, Y., Subbotina, I., Chen, H., Bogomolova, I., Xu, X.: Black carbon decomposition and incorporation into soil microbial biomass estimated by 14C labeling, Soil. Biol. Biochem., 41, 210–219, https://doi.org/10.1016/j.soilbio.2008.10.016, 2009.
Lefroy, R. D. B., Blair, G., and Stong, W. M.: Changes in soil organic matter with cropping as measured by organic carbon fractions and 13C natural isotope abundance., Plant. Soil., 155, 399–402, https://doi.org/10.1007/BF00025067, 1993.
Lehmann, J. and Kleber, M.: The contentious nature of soil organic matter, Nature, 528, 60–68, https://doi.org/10.1038/nature16069, 2015.
Liang, C., Schimel, J. P., and Jastrow, J. D.: The importance of anabolism in microbial control over soil carbon storage, Nat. Microbiol., 2, 1–6, https://doi.org/10.1038/nmicrobiol.2017.105, 2017.
Liu, Z., Gao, T., Liu, W., Sun, K., Xin, Y., Liu, H., Wang, S., Li, G., Han, H., Li, Z., and Ning, T.: Effects of part and whole straw returning on soil carbon sequestration in C3–C4 rotation cropland. J. Plant. Nutr. Soil Sci., 50, 73–85, https://doi.org/10.1002/jpln.201800573, 2019.
Ma, L. J., Lv, X. B., Cao, N., Wang, Z., Zhou, Z. G., and Meng, Y. L.: Alterations of soil labile organic carbon fractions and biological properties under different residue-management methods with equivalent carbon input, Appl. Soil. Ecol., 161, 103821, https://doi.org/10.1016/j.apsoil.2020.103821, 2021.
Mandal, B., Majumder, B., Bandyopadhyay, P. K., Hazra, G. C., Gangopadhyay, A., Samantarayr, N. A., Mishra, K., Chaudhury, J., Saha, M. N., and Kundu, S.: The potential of cropping systems and soil amendments for carbon sequestration in soils under long-term experiments in subtropical India, Glob. Change Biol., 13, 357–369, https://doi.org/10.1111/j.1365-2486.2006.01309.x, 2007.
Ndzelu, B. S., Dou, S., and Zhang, X.: Changes in soil humus composition and humic acid structural characteristics under different corn straw returning modes, Soil. Res., 58, 452–460, https://doi.org/10.1071/sr20025, 2020a.
Ndzelu, B. S., Dou, S., and Zhang, X.: Corn straw return can increase labile soil organic carbon fractions and improve water-stable aggregates in Haplic Cambisol, J. Arid. Land., 12, 1018–1030, https://doi.org/10.1007/s40333-020-0024-7, 2020b.
Nelson, D. W. and Sommers, L. E.: Total carbon, organic carbon and organic matter, in: Methods of Soil Analysis, Part 2: Chemical and Microbiological Properties, edited by: Page, A. L., American Society Agronomy, Madison, WI, 539–579, https://doi.org/10.2134/agronmonogr9.2.2ed.c29, 1982.
Ng, L. C., Sariah, M., Radziah, O., Abidin, M. A. Z, and Sariam, O.: Development of microbial-fortified rice straw compost to improve plant growth, productivity, soil health, and rice blast disease management of aerobic rice, Compost. Sci. Util., 24, 86–97, https://doi.org/10.1080/1065657x.2015.1076750, 2016.
Nigussie, A., Dume, B., Ahmed, M., Mamuye, M., Ambaw, G., Berhium, G., Biresaw, A., and Aticho, A.: Effect of microbial inoculation on nutrient turnover and lignocellulose degradation during composting: A meta-analysis, Waste Manage., 125, 220–234, https://doi.org/10.1016/j.wasman.2021.02.043, 2021.
Olk, D., Perdue, E., McKnight, D., Chen, Y., Farenhorst, A., Senesi, N., Chin, Y. P., Schmitt, K. P., Hertkorn, N., and Harir, M.: Environmental and agricultural relevance of humic fractions extracted by alkali from soils and natural waters, J. Environ. Qual., 48, 217–232, https://doi.org/10.2134/jeq2019.02.0041, 2019.
Organo, N. D., Granada, S. M. J. M., Pineda, H. G. S., Sandro, J. M., Nguyen, V. H., and Gummert, M.: Assessing the potential of a Trichoderma-based compost activator to hasten the decomposition of incorporated rice straw, Sci. Rep., 12, 448, https://doi.org/10.1038/s41598-021-03828-1, 2022.
Pan, F., Li, Y., Chapman, S. J., Khan, S., and Yao, H.: Microbial utilization of rice straw and its derived biochar in a paddy soil, Sci. Total. Environ., 559, 15–23, https://doi.org/10.1016/j.scitotenv.2016.03.122, 2016.
Roldán, M. L., Corrado, G., Francioso, O., and Sanchez-Cortes, S.: Interaction of soil humic acids with herbicide paraquat analyzed by surface-enhanced Raman scattering and fluorescence spectroscopy on silver plasmonic nanoparticles, Anal. Chim. Acta, 699, 87–95, https://doi.org/10.1016/j.aca.2011.05.001, 2011.
Romero, E., Plaza, C., Senesi, N., Nogales, R., and Polo, A.: Humic acid-like fractions in raw and vermicomposted winery and distillery wastes, Geoderma, 139, 397–406, https://doi.org/10.1016/j.geoderma.2007.03.009, 2007.
Ros, M., Pascual, J. A., Garcia, C., Hernandez, M. T., and Insam, H.: Hydrolase activities, microbial biomass and bacterial community in a soil after long-term amendment with different composts, Soil. Biol. Biochem., 38, 3443–3452, https://doi.org/10.1016/j.soilbio.2006.05.017, 2006.
Sainepo, B. M., Gachene, C. K., and Karuma, A.: Assessment of soil organic carbon fractions and carbon management index under different land use types in Olesharo catchment, Narok county, Kenya, Carbon. Balance. Manag., 13, 4–13, https://doi.org/10.1186/s13021-018-0091-7, 2018.
Sajid, S., Zveushe, O. K., de Dios, V. R., Nabi, F., Lee, Y. K., Kaleri, A. R., Ma, L., Zhou, L., Zhang, W., Dong, F., and Han, Y.: Pretreatment of rice straw by newly isolated fungal consortium enhanced lignocellulose degradation and humification during composting, Bioresour. Technol., 354, 127150, https://doi.org/10.1016/j.biortech.2022.127150, 2022.
Sarmistha, S., Harekrushna, S., Totan, A., Pratap, B., Arup, K. M., Gaurav, K., and Sayaji, T. M.: Trichoderma-mediated rice straw compost promotes plant growth and imparts stress tolerance, Environ. Sci. Pollut. Res., 21, 13701, https://doi.org/10.1007/s11356-021-13701-3, 2021.
Siddiquee, S., Shafawati, S. N., and Naher, L.: Effective composting of empty fruit bunches using potential Trichoderma strains, Biotechnol. Rep., 13, 1–7, https://doi.org/10.1016/j.btre.2016.11.001, 2017.
Soil Survey Staff: Keys to Soil Taxonomy, 12th edn., United States Department of Agriculture, Natural Resources Conservation Service, Washington, DC, ISBN: 978-0160923210, 2014.
Sprunger, C. D., Culman, S. W., Palm, C. A., Thuita, M., and Vanlauwe, B.: Long-term application of low C:N residues enhances maize yield and soil nutrient pools across Kenya, Nutr. Cycl. Agroecosyst., 114, 261–276, https://doi.org/10.1007/s10705-019-10005-4, 2019.
Sugahara, K. and Inoko, A.: Composition analysis of humus and characterization of humic acid obtained from city refuse compost, Soil Sci. Plant Nutr., 27, 213–224, https://doi.org/10.1080/00380768.1981.10431273, 1981.
Tang, H. M., Xiao, X. P., Tang, W. G., Li, C., Wang, K., Li, W. Y., Cheng, K. K., and Pan, X. C.: Long-term effects of NPK fertilizers and organic manures on soil organic carbon and carbon management index under a double-cropping rice system in southern China, Commun. Soil. Sci. Plant. Anal., 49, 1976–1989, https://doi.org/10.1080/00103624.2018.1492600, 2018.
Vance, E. D., Brookes, P. C., and Jenkinson, D. S.: An extraction method for measuring soil microbial biomass C, Soil. Biol. Biochem., 19, 703–707, https://doi.org/10.1016/0038-0717(87)90052-6, 1987.
Vanlauwe, B., Gachengo, C., Shepherd, K., Barrios, E., Cadisch, G., and Palm, C. A.: Laboratory validation of a resource quality-based conceptual framework for organic matter management, Soil. Sci. Soc. Am. J., 69, 1135–1145, https://doi.org/10.2136/sssaj2004.0089, 2005.
Vargas-García, M. C., Suárez-Estrella, F. F., López, M. J., and Moreno, J.: Influence of microbial inoculation and co-composting material on the evolution of humic-like substances during composting of horticultural wastes, Process Biochem., 41, 1438–1443, https://doi.org/10.1016/j.procbio.2006.01.011, 2006.
Villamil, M. B., Little, J., and Nafziger, E. D.: Corn residue, tillage, and nitrogen rate effects on soil properties, Geoderma, 151, 61–66, https://doi.org/10.1016/j.still.2015.03.005, 2015.
Xu, M., Lou, Y., Sun, X., Wang. W., Baniyamuddin, M., and Zhao, K.: Soil organic carbon active fractions as early indicators for total carbon change under straw incorporation, Biol. Fert. Soils., 47, 745–752, https://doi.org/10.1007/s00374-011-0579-8, 2011.
Yang, X., Meng, J., Lan, Y., Chen, W., Yang, T., Yuan, J., Liu, S., and Han, J.: Effects of maize stover and its biochar on soil CO2 emissions and labile organic carbon fractions in Northeast China, Agric. Ecosyst. Environ., 240, 24–31, https://doi.org/10.1016/j.apsoil.2020.103821, 2020.
Yang, Y. N., Wang, L. L., Zhang, Y. F., Li, L. B., Shi, X. D., Liu, X. T., Ren, X. D., and Dou, S.: Transformation of corn stalk residue to humus-like substances during solid-state fermentation, Sustainability, 11, 6711, https://doi.org/10.3390/su11236771, 2019.
Zhang, P., Wei, T., Li, Y. L., Wang, K., Jia, A. K., Han, Q. F., and Ren, X. L.: Effects of straw incorporation on the stratification of the soil organic C, total N and C:N ratio in a semiarid region of China, Soil Till. Res., 153, 28–35, https://doi.org/10.1016/j.still.2015.04.008, 2015a.
Zhang, J., Lv, B. Y., Xing, M. Y., and Yang, J.: Tracking the composition and transformation of humic and fulvic acids during vermicomposting of sewage sludge by elemental analysis and fluorescence excitation–emission matrix, Waste Manage., 39, 111–118, https://doi.org/10.1016/j.wasman.2015.02.010, 2015b.
Zhang, X., Dou, S., Ndzelu, B. S., Guan, X. W., Zhang, B. Y., and Bai, Y.: Effects of different corn straw amendments on humus composition and structural characteristics of humic acid in black soil, Commun. Soil. Sci. Plan., 51, 107–117, https://doi.org/10.1080/00103624.2019.1695827, 2019.
Zhang, Y., Dou, S., Hamza, B., Ye, S., and Zhang, D.: Mechanisms of three fungal types on humic-like substances formation during solid-state fermentation of corn straw, Intl. J. Agric. Biol., 24, 970–976, http://www.fspublishers.org/Issue.php?y=2020&v_no=23&categoryID=908 (last access: 15 September 2022), 2020.
Zhang, Y. F., Dou, S., Ye, S. F., Zhang, D. D., Ndzelu, B. S., Zhang, X. W., and Shao, M. J.: Humus composition and humic acid-like structural characteristics of corn straw culture products treated by three fungi, Chem. Ecol., 37, 164–184, https://doi.org/10.1080/02757540.2020.1855154, 2021.