the Creative Commons Attribution 4.0 License.
the Creative Commons Attribution 4.0 License.
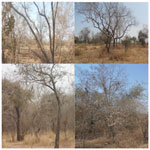
Effects of environmental factors and soil properties on soil organic carbon stock in a natural dry tropical area of Cameroon
Nérine Mabelle Moudjie Noubissie
Estelle Lionelle Tamto Mamdem
Simon Djakba Basga
Dieudonne Lucien Bitom Oyono
Research carried out on soil organic carbon stock (SOCS) in the Sudano-Sahelian region of Cameroon is very rare. The few existing studies are mostly available in reports and concern in most cases carbon stocks in plant biomass. In order to contribute to the documentation on soils in this part of the country, the present work was designed to evaluate the SOCS in the main soil types and the influence of environmental factors and soil properties on these stocks under the natural dry tropical area of the Sudano-Sahelian zone of Cameroon. The study was undertaken in four sites, including three natural forest reserves (Laf, Zamai, Kosohon) and one national park (Mozogo), located at different latitudes. Three replicates were collected at each site, giving rise to three sampling points chosen per site, from 0 to 75 cm depth, for the determination of SOCS. At each sampling point, soils were sampled using depth increments of 25 cm from the surface. The studied area is covered by Haplic Vertisols, Dystric Arenosols, Dystric Leptosols and Dystric Planosols. Total SOCS (T-SOCS) content, which refers to a depth of 75 cm, decreases with increasing latitude, with 249±26.26 Mg ha−1 in Vertisols at Laf forest reserve most southerly located, 199±8.00 Mg ha−1 in Arenosols at Zamai forest reserve, 166±16.63 Mg ha−1 in Leptosols at Kosohon forest reserve and 161±8.88 Mg ha−1 in Planosols at Mozogo national park most northerly located, regardless of the altitude. No significant correlation was noted between T-SOCS and the altitude. A good correlation was noted between precipitation which decreases with increasing latitude and T-SOCS, indicating the importance of climate in the distribution of T-SOCS in the study area, which directly influences the productivity of the vegetation. More than 60 % of the SOCS was stored below the first 25 cm from the soil surface, a peculiarity of SOCS in drylands. The SOCS in the Sudano-Sahelian area of Cameroon is mainly influenced by climate and vegetation.
- Article
(3081 KB) - Full-text XML
- BibTeX
- EndNote
Soil is the largest carbon (C) pool, and its content in the first 100 cm is estimated at about 1500 Pg (1 Pg = 1015 g), which represents more C than what is currently contained in the atmosphere and vegetation combined (Boulmane et al., 2010; Lehmann and Kleber, 2015). Soil organic C stocks are crucial for a wide range of ecosystem services such as climate regulation through atmospheric CO2 storage (Olson et al., 2016; Greiner et al., 2017; Mayer et al., 2019). As a result of photosynthesis, vegetation fixes about 120 Pg of C per year from the atmosphere, and half of it is returned to the atmosphere by plants (Bernoux and Chevalier, 2013). Part of the atmospheric C drawn by plants is stored in biomass and soil in the form of organic matter, consisting of plant and animal detritus at various stages of decomposition, cells and tissues of soil microbes, and substances that soil microbes synthesize (Eswaran et al., 1993; Mayer et al., 2019). Terrestrial ecosystems thus have the potential to constitute a C sink, slowing down the increase of CO2 in the atmosphere (Mayer et al., 2019). The interaction between the root system and the soil profile has profound impact on soil C accumulation, where the root system can contribute to soil organic carbon (SOC) stocks (Olson and Al-Kaisi, 2015).
Soil organic matter (SOM), which contains more than 50 % C by weight, plays a fundamental role in the overall behaviour of soils and the ecosystems they support, especially the physical qualities of soils, the stimulation of biological activity of soil, the storage and provision of water and nutrients for plants, and the regulation of pollutants (Haygarth and Ritz, 2009; Fernandez et al., 2009; Liu et al., 2012). It is generally considered a primary indicator of soil quality for agricultural and environmental functions (Plaza et al., 2018). Thus, loss of SOC or SOM results in the loss of soil quality and impaired associated functions including soil degradation, decline in agronomic productivity and food insecurity, resulting in malnutrition and starvation (Haygarth and Ritz, 2009; Brown and Huggins, 2012; Olson and Al-Kaisi, 2015; Olson et al., 2016; Plaza et al., 2018). Increasing the SOC or SOM directly improves the quality of the soil and hence contributing to the resilience and sustainability of agriculture and consequently to the food security of societies as C is sequestered. Nowadays, many phenomena such as climate change, land degradation and loss of biodiversity make soils and their C storage one of the most vulnerable resources (Bardgett, 2005; Maestre et al., 2013; Soleimani et al., 2019). Currently, the storage of SOC is a topic of paramount importance in international negotiations to fight against climate change through a reduction in greenhouse gas emissions that contribute to global warming (FAO, 2017). Despite the importance of soils in C storage, decisions in the political sphere in terms of climate change mitigation have long focused on the industrial, transport and energy sectors only. The impact of forestry and agricultural activities on C sequestration has until recently been neglected, making agriculture and SOC the poor parents of international negotiations (Bernoux and Chevalier, 2013). It was after the 2008 and 2009 food prices crises and hunger riots in Africa that international debates focused on the soil issue. SOC is now at the centre of global environmental issues, especially in the framework of the United Nations Agreements on Climate Change, Convention on Biological Diversity and the United Nations Convention on Desertification (Bernoux and Chevalier, 2013).
In dry areas, SOC content is low (less than 1 % of the soil mass), whereas in the temperate zone it reaches 4 % to 5 % in grassland soils or under forest (Bernoux and Chevalier, 2013; Lehmann and Kleber, 2015; Plaza et al., 2018). This low SOC content in dry regions is due to several anthropogenic and natural factors that led to soil C losses (Lal, 2004; Pineiro et al., 2010; Bernoux and Chevalier, 2013; Wang et al., 2020). Anthropogenic factors include overgrazing and deforestation (Lal, 2004; Plaza et al., 2018; Soleimani et al., 2019; Wang et al., 2020). Natural factors that contribute to SOC degradation/loss are poor weather conditions characterized by low rainfall and high temperatures, disappearance of vegetation, aggressive rains, erosion and high mineralization (Von Lutzow et al., 2006; Olson et al., 2016; Wang et al., 2020). Despite this low C concentration in dryland soils, it is important to study the C stock in this area for several reasons. Soils in dry areas cover about 41 % of the global land area, and their C stock should be carefully considered for good environmental management and sustainability of the agroecosystem (Haygarth and Ritz, 2009; Lal, 2009; Hounkpatin et al., 2018). Moreover, their stock deserves to be studied because of its permanence, i.e. the duration during which C is stored in dryland soils in relation to wetland soils and if these soils are treated sustainably. Soil has the potential to sequester large amounts of C, which contributes to the adaptation and mitigation of climate change (Plaza et al., 2018). It is known that increasing C content in terrestrial ecosystems is one of the main approaches to mitigate anthropogenic production of atmospheric CO2 (Jiang et al., 2019). However, SOC storage and dynamics depend on region, parent material, time, cover vegetation, topography, and soil properties such as texture and the cation exchange capacity (Muñoz-Rojas et al., 2012; Jiménez-González et al., 2020; Reyna-Bowen et al., 2020). Therefore, local studies are necessary to appraise the potential of soil to store C properly (Reyna-Bowen et al., 2020).
In Cameroon, the few existing studies were mainly achieved in the humid tropical part of the country (Amougou et al., 2016; Tsozué et al., 2019). Studies on the Sudano-Sahelian part of the country are scare, and the few that exist are mainly available in unpublished reports or are more concerned with C stocks in plant biomass (Ibrahim and Habib, 2008; Tchobsala et al., 2014, 2016). Moreover, many studies have ignored deeper SOC in the world (Ruiz Sinoda et al, 2012; Tornquist et al., 2009; Nyameasem et al., 2020; Schaefer et al., 2020; Mabicka et al., 2021). The present work aims to evaluate the SOC stock (SOCS) in the main soil types under the natural dry tropical area in the Sudano-Sahelian zone of Cameroon. More specifically, it focused on (i) the identification of the main soil types in the natural ecosystems that constitute the study area, (ii) the assessment of the SOCS in each soil type, (iii) the influence of soil depth in the storage of SOC, and (iv) the evaluation of the influence of environmental factors and soil properties on these stocks. To achieve this goal, four sites were chosen to conduct the study in the Far North Region of Cameroon, namely Laf, Zamai, Kosohon and Mozogo.
2.1 Study area
The study was conducted in the Far North Region of Cameroon (10–13∘ N; 13–15∘ E) which covers an area of about 3 424 600 ha (Fig. 1). The climate is dry tropical in nature and characterized by two highly contrasted seasons with a long dry season from October to May and a short rainy season from June to September. The mean annual rainfall and temperature are 800–1000 mm and 26–28 ∘C, respectively. The geologic substratum consists of Precambrian formations (gneiss, migmatite, mica schist, granite, syenite), volcanic formations (basalts, trachyte, rhyolite) and alluvial deposits (Ngounouno et al., 2000; Tamen et al., 2015; Gountié Dedzo et al., 2019). Two morphological units are distinguished in the study area: mountain (600–1000 m as an average altitude) and plain (400–600 m as an average altitude). The vegetation of the area belongs to the Sudano-Sahelian domain with woody arboreous or scrubby vegetation with abundant perennial grasses. The characteristic species are summarized in Table 1. However, a major part of the natural vegetation has been degraded and the soil subjected to cultivation and fallowing. Natural vegetation only exists now in dry forest reserves and national parks.
2.2 Experimental design and sampling
The study was undertaken in four sites, including three natural forest reserves (Laf, Zamai, Kosohon) and one national park (Mozogo), located along a latitudinal gradient and corresponding respectively to low-density shrub savannah, herbaceous savannah, moderately densified shrub savannah and wooded savannah (Figs. 1 and 2). The characteristics of the studied sites are summarized in Table 1. After the description of the physical characteristics of the studied sites (relief, geologic substrate, vegetation), soil classification was done with the International Union of Soil Sciences (IUSS) (IUSS Working Group WRB 2015), and results are presented in Table 2. SOCS was thereafter evaluated at 75 cm depth since SOC in dryland is believed to reside in topsoil (Ciais et al., 2011; Plaza et al., 2018). Two replicates were made, giving rise to three sampling points chosen per site, separated from each other by a minimum distance of 100 m. The soil samples (about 500 g of disturbed samples and undisturbed clods) were taken from three soil sections of 25 cm (S1: 0–25 cm, S2: 25–50 cm and S3: 50–75 cm), corresponding to a total of 36 disturbed samples and 36 undisturbed clods, in order to establish a better comparison between the different study sites. Undisturbed clods were taken in the same vicinity as disturbed samples. They were collected for bulk density analysis. Clods were first roughly sized in order to fit into the cell ( cm) of the clod box.
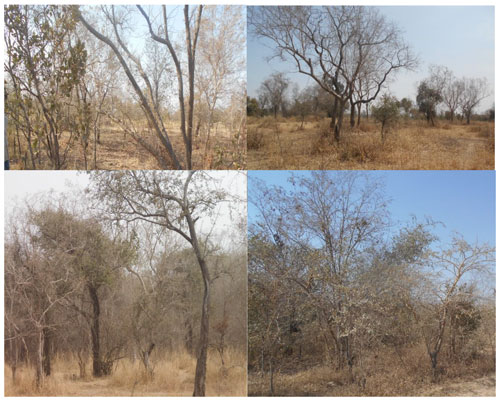
Figure 2From left to right clockwise: low-density shrub savannah (Laf), herbaceous savannah (Zamai), moderately densified shrub savannah (Kosohon) and wooded savannah (Mozogo).
2.3 Laboratory analysis
In the laboratory, after sieving the soil through 2 mm meshes, physical and chemical analyses were carried out. Bulk density was measured by the paraffin-coated clods method (USDA, 2004). The particle size distribution was analysed by sieving and using Robinson's pipette method (USDA, 2004). Soil pH was measured with pH meter equipped with a glass electrode in 1 : 2.5 soil–water suspensions (Guitian and Carballas, 1976). Exchangeable cations were extracted by 1 N NH4OAc at pH 7, and their concentrations were determined by atomic absorption spectrometry (Perkin Elmer) for Ca and Mg and by flame emission spectrometry for K and Na. Cation exchange capacity (CEC) was also determined using the ammonium acetate method at pH 7, by a direct continuation using a 1 N potassium chloride (KCl) saturation solution. SOC was determined by dichromate oxidation using the Walkley–Black method (Walkley and Black, 1934). SOM content was obtained using a conversion factor of 1.724 (Walkley and Black, 1934). Total nitrogen (N) was determined with the Kjeldahl method (Bremner, 1996), and the C : N ratio calculated by dividing the SOC concentration by the N concentration. Available phosphorus was extracted from the soil using Bray-2 solution as extractant (Bray and Kurtz, 1945).
SOC stock (SOCS), expressed for a specific depth in Mg ha−1, was computed as the product of SOC concentration, bulk density, depth and gravels, according to IPCC (2003) as follows: SOCS = (SOC concentration × BD × d × (1−δ2 mm %) × 10), where SOC is the organic C content (g kg−1), d is the thickness of the control section (m), δ2 mm is the ratio of gravel larger than 2 mm in size in the soil, and BD the soil bulk density (Mg m−3). Total SOC stock (T-SOCS), referring to 75 cm of depth (Mg ha−1), was calculated according to IPCC (2003) as follows: T-SOCS = ∑soil section SOC Stocksoil section.
2.4 Statistical analysis
The normality of the distribution was studied by the Anderson–Darling normality test. Descriptive statistics (mean values, standard deviations and correlation) were used to characterize the general trends of soil properties, using Excel 2007. A Spearman rank correlation coefficient was carried out in order to assess the possible connection between the soil properties and SOC in each site. Kruskal–Wallis statistical test was used to identify the statistical significance of the differences in each soil variable, among each sampling point within the site and among the sites. Significance was considered at p<0.05. All analyses were performed using XSLSAT 2008.6.03 software for Excel.
3.1 Physical characteristics of the studied soils
According to the International Soil Classification System, soils of the study area show a sandy clay loamy (SCL) to sandy loamy (SL) texture. Sand is the most abundant fraction. The highest sand contents were observed in the 0–25 cm depth interval in Vertisols (71±12.12 %), Leptosols (71.33±2.88 %) and Arenosols (76.66±3.21 %). It decreased from the soil surface to the base of soil in the 50–75 cm intervals (Table 3). In the Planosols, however, high sand content (79±6.42 %) was observed at the middle 25–50 cm interval, in line with the classification of the studied soil. Clay content increased from the surface to the bottom of the soil, except in the Planosols where the low clay content (15±6.42 %) was noted in the middle part of the soil, in the 25–50 cm interval (Table 3). Silt contents were low, ranging between 4.0±1.73 and 9.3±2.51 %. Bulk density (BD) values increased with depth except in Vertisols where similar values were observed in the two upper sections. Similarly, in Planosols, as for the sand content, the high value of bulk density (1.68±0.11 Mg m−3) was observed in the 25–50 cm interval. With regard to soil pH values, Arenosols, Leptosols and Planosols were acidic (4.7±0.47 to 5.6±0.4), and Vertisols were close to neutral (6.8±0.59 to 7.9±0.84). However, a very acid value of 4.7±0.47 was noted in the 25–50 cm interval of Planosols (Table 3). Gravel content in the studied soil was consistently low (<3 %). A value of 7 % was, however, obtained in the Vertisols, in line with the presence of calcareous nodules in these soils. Generally, there was no significant difference between main soil physical parameters within each site and between sites.
Table 3Main soil physical and chemical properties (mean ± SD*) in the study area.
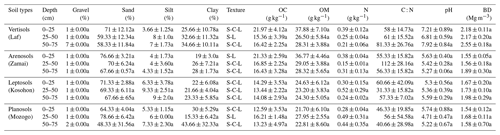
* Standard deviation; BD: bulk density; S-C-L: sandy clay loam; S-L: sandy loam. Numbers followed by different lower-case letters within the same column have significant differences (p<0.05) at different depths, considering the same topographic position.
3.2 Soil organic matter, nitrogen and C : N ratio
The average SOM values varied between 21.7±6.10 and 37.9±7.10 g kg−1 (Table 3). The average SOM values ranged between 26.50±5.84 and 37.88±7.10 g kg−1 in Vertisols and Arenosols and between 21.70±6.10 and 27.95±2.55 g kg−1 in Leptosols and Planosols. The SOM content decreased with soil depth at Zamai in Arenosols and at Kosohon in Leptosols. In Vertisols at Laf, there was a decrease of SOM from the soil surface in the 0–25 cm interval to 25–50 cm depth and an increase from this depth to the base of soils in the 50–75 cm interval, whereas in Planosols SOM exhibited an opposite trend (Table 3). Total nitrogen (TN) contents were low. TN decreased with soil depth in Vertisols from 0.39 g kg−1 in the 0–25 cm interval to 0.21 g kg−1 in the 50–75 cm interval at the base of the soil profile. In Arenosols, there was a decrease of TN content from the soils surface to the subsurface of the soil and an increase to the base of the soil. TN content in Leptosols and Planosols exhibited an opposite trend to TN contents in Arenosols. The C : N ratios ranged between 31±15.82 and 112±28.16. Besides the high value of C : N ratio in the 25–50 cm interval of Arenosols (112±28.16), the highest ratios were observed in Vertisols, while the lowest rations were noted in Planosols (Table 3). A significant negative correlation was observed between SOM and silt fraction (−0.70, p<0.05) (Table 4).
3.3 Evolution of SOC and SOCS contents in the studied soils
The highest SOC content in the study area was observed in the 0–25 cm interval in Vertisols (21.97±4.12 g kg−1). The lowest one was also observed in the same interval but in Planosols (12.59±3.53 g kg−1) (Table 5). In fact, besides Arensols where a regular decrease of SOC with depth was observed, a zigzag evolution with depth was observed for the other three soil types, with the trend in vertisols opposing to that observed in Leptosols and Planosols (Table 5). The T-SOC was high in Vertisols and Arenosols (53.75±3.25 and 54.61±2.71 g kg−1 respectively) and low in Leptosols and Planosols (41.21±2.89 and 42.03±3.33 g kg−1 respectively). With respect to SOCS, a high content was observed in the 0–25 cm interval in Vertisols (102.04±30.59 Mg ha−1) (Fig. 3). SOCS content decreased with depth, reaching 70.99±21.68 Mg ha−1 in the 25–50 cm interval and increased thereafter reaching 75.57±26.50 Mg ha−1 in the 50–75 cm interval (Table 5). This trend was similar in Arenosols and Leptosols but differed from that of Planosols where SOCS content increased from the soil surface to the middle part of the soil (47.03±4.73 to 61.82±1.93 Mg ha−1) and decreased thereafter reaching 52.10±19.98 Mg ha−1 in the 50–75 cm at the base of the soil. The highest T-SOCS was obtained in Vertisols most southerly located, while the lowest was obtained in Planosols most northerly located. It appears that T-SOCS content decreased with increasing latitude, with 248.60±26.26 Mg ha−1 in Vertisols at Laf, 199.04±8.00 Mg ha−1 in Arenosols at Zamai, 166.52±16.63 Mg ha−1 in Leptosols at Kosohon and 160.95±8.88 Mg ha−1 in Planosols at Mozogo (Table 4 and Fig. 1), irrespective of the altitude. In fact, a very weak correlation was noted between the altitudinal gradient and SOCS (R2=0.13), meaning that there was no influence of the altitudinal gradient on the distribution of T-SOCS in the studied area (Fig. 4). A good correlation was noted, on the contrary, between precipitation which decreased with increasing latitude and SOCS (r=0.64), indicating the importance of the climate in the distribution of T-SOCS in the study area (Fig. 5). Overall, 58.94 %, 57.87 %, 64.23 % and 70.77 % of SOC respectively in Vertisols, Arenosols, Leptosols and Planosols was stored below the first 25 cm from the soil surface.
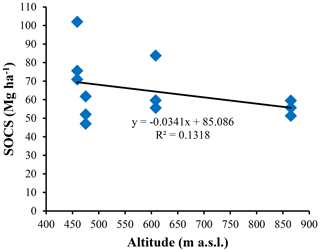
Figure 4Plots of the soil organic carbon stock (SOCS) versus altitudinal gradient in the study area.
Table 5Soil organic carbon (SOC) content and soil organic carbon stock (SOCS) (mean ± SD*) in the study area.
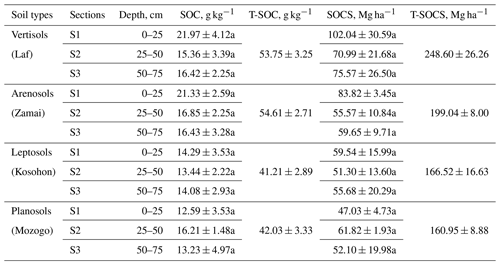
* Standard deviation. Numbers followed by different lower-case letters within the same column have significant differences (p<0.05) at different depths, considering the same topographic position.
4.1 Soil physical properties
Clay content increased with depth in Vertisols and Arenosols. This might be due to eluviation and illuviation processes as consequences of vertical movement of water through the soil profile. The clay content was almost similar in the three increments in Leptosols, in line with the weak development and differentiation of the soil. Contrastingly in Planosols, the presence of rusty and greyish spots in addition to the abrupt variation of sand and clay fractions contents had already been reported elsewhere (Driessen et al., 2001; Van Ranst et al., 2011). Bulk density is high, especially in Vertisols. Similar values were obtained by Azinwi et al. (2011). This might be due to the Sudano-Sahelian climate with 8 to 9 months of dry season which leads to soil compaction and sealing (Tsozué et al., 2014). Bulk density increases with soil depth in Vertisols and Arenosols in line with increase of clay fraction. In Planosols, the evolution of bulk density with depth follows that of sand. Bulk density is known to vary under the influence of particle size distributions in a soil layer (Carter, 1990; Tuttle et al., 1988). The increase of bulk density with soil depth regardless of the texture in Leptosols might be linked to the progressive weathering of the parent rock. High pH values are noted in Vertisols. The slightly acid to neutral nature of studied Vertisols is unfailingly attributed to the presence of free calcium from calcareous nodules or base-rich parent materials from which they were developed. In fact, weathering of base-rich parent materials could increase the content of alkali and alkaline earth elements (Ca2+, Mg2+, K+ or Na+) in soils which would consequently increase the soil pH. Similar results were obtained by Nguetnkam (2004) in the Far North Region of Cameroon and Moustakas (2012) in the north-eastern part of Greece. Low pH in the bleached layer of Planosols might be due to ferrolysis processes which have been suggested to explain oxides (Mn-Fe) segregation and gleying in such environments (Singh et al., 1998; Barbiero et al., 2010) and textural variations (O'Geen et al., 2008; Van Ranst et al., 2011; da Silva et al., 2019). It is common for Planosols to present pH < 5.5 due to acidification and destruction of clay minerals, leaving only quartz as the major constituent (Brinkman, 1970; Spiau and Pedro, 1986; Van Breemen and Buurman, 2002).
4.2 Soil organic matter, nitrogen and C : N ratio
The studied soils are the most common under dryland ecosystems (FAO, 2004). Their average SOM values are low, ranging between 21.70 ± 6.10 and 37.88 ± 7.10 g kg−1, compared to those obtained in soils of humid-region soils by Tsozué et al. (2019), which range between 15.10 and 180.50 g kg−1. The low SOM concentrations in the study area might be due to the semi-arid climate and the sandy textures (Gallardo et al., 2000; González and Candás, 2004; Parras-Alcantara et al., 2015). This is in agreement with observations already made in dryland soils (Lal, 2004; Plaza et al., 2018). SOM influences almost all physical, chemical, and biological properties and processes in such ecosystems (Lal, 2004, 2009; Adoum et al., 2017). It promotes soil aggregation, which improves soil structure, porosity and moisture-holding capacity, thus reducing the severity of water scarcity and protecting soils from erosion and compaction (Ruiz Sinoga et al., 2012; Yüksek, 2012). Nitrogen (N) is an essential nutrient used in relatively large amounts by all living organisms. Generally, the concentration of N is high in areas where SOM and SOC are high (Sakin, 2012), indicating that N nutrition of plants greatly depends on the maintenance of SOM and SOC level. In the study area, no significant correlation was noted between N and SOM. Except in Planosols where the evolution of SOC with soil depth is modelled on that of N, the absence of correlation between N and SOM implies that in the studied area, N and SOC act independently. The C : N ratios are high, ranging between 31.33±15.82 and 112±28.16, suggesting an absence of reactive and readily biodegradable SOM. The wide variation of C : N ratios across the studied area might be due to the tropical dry climate, natural vegetation, intrinsic soil properties and soil drainage (Yoh, 2001; Cools et al., 2013; Zinn et al., 2018). Since C : N ratio can reflect the degree of decomposition of SOM, this might indicate that the C fractions in the studied soils are still composed of various C components with different properties (Chen et al., 2020).
4.3 SOC and SOCS
SOC content was highest in the top 25 cm in Vertisols, Arenosols and Leptosols. This is in line with Batjes (1996), who reported that the amount of SOC in the topsoil was higher than in subsoil. SOM could be concentrated, and the mechanisms of C mineralization and immobilization are more active in the soil's top 30 cm (Hiederer, 2009). Similar results were also obtained by Reyna-Bowen et al. (2020) in Hinojosa del Duque in Spain where they noted that trees' influence on SOC is strongly related to their roots in the A horizon. In Planosols, there was an increase in SOC content in the bleached layer. This is in agreement with observations made by da Silva et al. (2019) in three Brazilian Planosols. The low SOC content in the 0–25 cm interval of Planosols might be due to the fact that these soils are periodically flooded. In fact, in periodically flooded soils, aerobic periods promote higher microbial activity and more rapid decomposition of SOM, which is also poorly stabilized by sorption in the sandy surface horizons (Vepraskas, 2001; da Silva et al., 2019). In the study area, a high T-SOC value is observed in Vertisols and Arenosols (53.75 ± 3.25 and 54.61 ± 2.71 g kg−1 respectively) and a low one is observed in Leptosols and Planosols (41.21 ± 2.89 and 42.03 ± 3.33 g kg−1 respectively). The T-SOC content in the studied Vertisols (53.75 g kg−1) is 50 % of the T-SOC content obtained in the semi-evergreen medium forest Vertisols (95.20 g kg−1), but 200 % of the quantity obtained in the flooded low rainforest zone Vertisols (28.60 g kg−1), all in the warm subhumid equatorial climate of Yucatán Peninsula in Mexico characterized by dry winters (Tsao, 2017). It is, however, lower than that obtained in Spain by Muñoz-Rojas et al. (2015). This difference can be attributed to their richness in clay and the climatic conditions. In fact, climatic conditions in the study area are close to those of Yucatán Peninsula in Mexico (tropical climate), but in Spain, low temperature would slowdown the microbiological activities leading to an increase in SOC contents. Also, the wetting and drying cycle plays a crucial role in aggregation and C stabilization in Vertisols (Rahman et al., 2018). On the other hand, the T-SOC content in Arenosols is 54.61 ± 2.71 g kg−1. This SOC content is higher than that found by Batjes (2008) on sandy marine sediments on freely drained Arenosols in the lower Congo. This difference can be attributed not only to parent materials but also to drainage conditions (freely drained sediments) that favour the leaching of SOM and SOC (Batjes, 2008; Torn et al., 2009). The T-SOC value of 41.21 ± 2.89 g kg−1 in Leptosols here is lower than that obtained in Umbric Leptosols of the temperate zone (52.40 ± 7.05 g kg−1) but higher than the value obtained in Mollic Leptosols under similar climatic conditions in Southern Spain (38.95 ± 6.41 g kg−1) studied by Parras-Alcantara et al. (2015), meaning that climate is not responsible for the variation of SOC contents in Spanish Leptosols.
The trend of SOCS contents with depth in the studied area follows that of SOC, in line with observations of Batjes (1996), Hiederer (2009) and da Silva et al. (2019). SOCS in the upper 30 cm in the studied Vertisols is 96.87 Mg ha−1. This value is higher than that obtained in the upper 30 cm of Vertisols (56.4 Mg ha−1) by Tornquist et al. (2009) in Brazil. It is also relatively higher than those obtained by Tsao (2017) in different land covers in Yucatán Peninsula in Mexico, with 70.70 Mg ha−1 in flooded low rainforest zone Vertisols, 80.03 Mg ha−1 in semi-deciduous low rainforest, 88.43 Mg ha−1 in Semi-evergreen low rainforest and 154.16 Mg ha−1 in semi-evergreen medium forest Vertisols. High T-SOCS in the studied Vertisols might be due to the fact that Vertisols contain smectitic clay minerals, with a high cation exchange capacity dominated by Ca2+ ions, which favours SOC stabilization and accumulation (Muneer and Oades, 1989; Tornquist et al., 2009). This is in line with high correlation between CEC and SOC commonly demonstrated worldwide. T-SOCS values are higher in Arenosols and Leptosols than those obtained in Mexico by Tsao (2017), irrespective of altitude. It might also be related to the nature of clay mineral as montmorillonite in Vertisols. They are also higher than the values documented in Europe by De Vos et al. (2015), with 102 Mg ha−1 in Arenosols and 134 Mg ha−1 in Leptosols. Another comparison could be made with Andosols from the eastern part of the African continent (Jones et al., 2013) and Andosols from the humid mountainous zone of south Cameroon (Tsozué et al., 2019), where locally they present elevated stocks reaching respectively 150 and 302 Mg ha−1. Soluble substances and labile compounds of litter are rapidly degraded in the early stages of decomposition by fast-growing microorganisms that might lead to the loss of SOC. Also, cellulose and lignin, the most abundant components of litter in Europe, for example, are decomposed slowly (Fioretto et al., 2005) and might negatively impact the SOC content. In the studied Planosols on the contrary, SOCS was high in the 0–25 cm interval, and the T-SOCS value was low in the study area (160.95 ± 8.88 Mg ha−1). This T-SOCS value, although low in the study area, was higher than that obtained by Batjes (1996) in the world (77 Mg ha−1 with coefficient of variation of 0.56), De Vos et al. (2015) in Europe (67 Mg ha−1 with coefficient of variation of 0.35) and Batjes (2002) in central and eastern Europe (108 Mg ha−1 with coefficient of variation of 0.18). The high T-SOCS value in the studied Planosols might be attributed to the very low slope gradient (2 %) and the possible presence of biocrusts which take C and N from the atmosphere and might protect soil from wind and water erosion in the studied area (Plaza et al., 2018). Globally, the obtained T-SOCS values in the studied area are close to those obtained by Adoum et al. (2017) (173.9 to 241.0 Mg ha−1) in polder soils under semiarid climate in Lake Chad.
4.4 Effect of environmental factors on SOCS
There was a decrease of SOCS with latitude in the studied area. This agrees with the findings by Jenny (1930), which revealed that the major reservoirs of soil C change with latitude. Most southerly, only a small fraction of the T-SOC is stored in surface detritus, and most of the C is in the mineral soil. Most northerly, slow litter decay leads to large accumulations of detrital organic material, and relatively little organic C is in the mineral soil. This is in agreement with a high T-SOCS content obtained in the Laf Vertisols (248.60 ± 26.26 Mg ha−1) most southerly located and a low T-SOCS obtained in the Mozogo Planosols (160.95 ± 8.88 Mg ha−1) most northerly located in the studied area. In fact, there is a decrease in total precipitation and an increase in the mean air temperature as one moves away from the Equator towards the Sahara, and thus with increasing latitude in this part of the tropical zone. This implies that T-SOCS storage responds negatively to increasing temperature and decreasing precipitation along with the latitude gradient. Similar results were obtained by Tang et al. (2020) in China. Climate appears to be the principal factor explaining the low SOC contents in the studied soils as already observed by Plaza et al. (2018), with precipitation explaining 41.15 % of the variability of SOCS (R2=0.4115) along the soil profiles. Similar observations were noted by Lozano-García et al. (2017) in the semiarid Mediterranean part of southern Spain and also by Jiménez-González et al. (2020) in the humid subtropical, Mediterranean and oceanic temperate part of Spain. Moreover, 58.94 %, 57.87 %, 64.23 % and 70.77 % of SOC respectively in Vertisols, Arenosols, Leptosols and Planosols are stored below the first 25 cm from the soil surface. This is a peculiarity of SOCS under dryland ecosystems. It is essentially climate dependent (Gray et al., 2016; Plaza et al., 2018). Globally, temperature and precipitation negatively and positively (respectively) affected SOCS, because they affect the balance between C inputs from plant residues and C outputs caused by microbial decomposition of SOM (Post et al., 1982; Wang et al., 2020).
Spearman correlation between different soil parameters of the study area show that there was no correlation between the different soil parameters and SOM apart from the negative relationship with silt contents. The lack of correlation between SOM and particle size fractions in different soils can be explained by the fact that the alteration in dry intertropical zone is essentially geochemical. The influence of the parent rock in the accumulation of SOM in the study area therefore seems negligible, in line with Plaza et al. (2018) observations. However, the availability of polyvalent cations such as Ca2+ from the weathering of a parent rocks in the soil, might be an important factor in the chemical protection of SOC (Briedis et al., 2012). In fact, Ca2+ is frequent in the soil solution and precipitates in the form of calcareous nodules at the base of soils in the study area.
The vegetation cover and/or the type of vegetation influences the amount of SOC stored in soils (Torn et al., 2009; Reyna-Bowen et al., 2020). The site of Laf most southerly located had the highest T-SOCS, while the site of Mozogo most northerly located had the lowest T-SOCS. The difference in T-SOCS contents might be due to the type of vegetation in the various sites. The Laf site would therefore have more productive vegetation due to high precipitation and thus provide more SOC than the other sites. This is also valid for the site of Kosohon which, although located at higher altitude (865 m a.s.l.) and therefore likely to have a high T-SOCS, shows a lower T-SOCS than that obtained in Vertisols at Laf. The influence of the vegetation might occur through deep root distribution and the chemical nature of litter (Bird and Torn, 2006; Zhang et al., 2008; Torn et al., 2009; Reyna-Bowen et al., 2020). Generally, decomposition of SOM involves multiple microbial processes catalysed by various enzymes (Zhang et al., 2020). Changes in microbial community and enzyme activity are therefore expected to influence the decomposition rate (Chenu et al., 2019; Wang et al., 2020; Zhang et al., 2020). It is demonstrated that soil acidification depresses the decomposition of SOM, both by decreasing microbial activity and by increasing protection of SOC by mineral phases (Zhang et al., 2020). Soil pH decreases with latitude and SOCS, thus reinforcing the fact that the productivity of the vegetation due to precipitation is a main factor controlling the distribution of SOC in the study area.
With respect to topography, T-SOCS contents decrease with increasing latitude, regardless of the altitude. Moreover, no significant correlation was noted between T-SOCS and the altitude. This implies that topography has no impact on the T-SOCS in the studied Sudano-Sahelian zone of Cameroon.
The role of topography and parent rock in the storage of SOC seems to be negligible in the studied area. The availability of polyvalent cations such as Ca2+ is linked to geochemical processes induced by the climate. The distinguishing factors affecting SOCS are thus the climate and the vegetation. According to Plaza et al. (2018), these two factors are the main soil-forming factors driving the SOM storage and consequently the SOCS in such dryland ecosystems.
The present work was designed to evaluate SOCS in the main soil types under the natural dry tropical area in the Sudano-Sahelian zone of Cameroon. The main soil types are Haplic Vertisols, Dystric Arenosols, Dystric Leptosols and Dystric Planosols. The C : N ratios are high, suggesting the absence of reactive and readily biodegradable SOM. Vertisols recorded the highest T-SOCS (248.60 ± 26.26 Mg ha−1), followed by Arenosols (199.04 ± 8.00 Mg ha−1), Leptosols (166.52 ± 16.63 Mg ha−1) and Planosols (160.95 ± 8.88 Mg ha−1). More than 60 % of SOCS is stored below the first 25 cm from the soil surface. The T-SOCS is controlled by climate and vegetation, with precipitation explaining 41.15 % of the variability of SOCS. The influence of the climate is manifested by the decrease in the SOCS as the latitude increases, in line with the increase of the temperature and the decrease of the rainfall as one move away from the Equator. The climate affects the primary productivity of the vegetation, which influences the quality and quantity of inputs to the SOM, as attested by the good correlation between precipitation and T-SOCS. No significant correlation was noted between T-SOCS and the altitude, meaning that there is no influence of altitude gradation in the repartition of T-SOCS in the studied area.
The data used in this study are available upon request from the corresponding author.
Remaining soil samples are logged and barcoded at the Department of Earth Sciences at the University of Maroua, Cameroon. Soil samples can be made available upon request through the corresponding author.
The conceptualization of the study was done by DT, with input from NMMN, ELTM, SDB and DLBO. The data acquisition, investigation, methodology and visualization for the paper were performed by DT, NMMN and DLBO, with substantial input from ELTM and SDB. DT, NMMN, ELTM and SDB wrote the initial draft, and DT and DLBO were involved in the reviewing, editing and the validation of the paper.
The authors declare that they have no conflict of interest.
Publisher’s note: Copernicus Publications remains neutral with regard to jurisdictional claims in published maps and institutional affiliations.
Authors thank the traditional and administrative authorities in the study area who provided both guides and a labourer for field work. Anonymous reviewers and the editor are also gratefully thanked for their comments and suggestions on the article.
This paper was edited by Paul Hallett and reviewed by Jennifer Wardle and one anonymous referee.
Adoum, A. A., Moulin, P., and Brossard, M.: Pioneering assessment of carbon stocks in polder soils developed in inter-dune landscapes in a semiarid climate, Lake Chad, C.R. Geosci., 349, 22–31, https://doi.org/10.1016/j.crte.2016.08.003, 2017.
Amougou, J. A., Bembong Ebokona, D. L., Batha, R. A. S., Mala, A. W., and Ngono, H.: Estimation du stock de carbone dans deux unités de terre en zone de savane du Cameroun, Regardsuds, 28–45, available at: https://regardsuds.org/1827-2 (last access: 5 October 2021), 2016.
Azinwi, T. P., Djoufac, W. E., Bitom, D., and Njopwouo, D.: Petrological, Physico-Chemical and Mechanical Characterization of the Topomorphic Vertisols from the Sudano-Sahelian Region of North Cameroon, Open Geol. Jo., 5, 33–55, 2011.
Barbiero, L., Mohan Kumar, M. S., Violette, A., Oliva, P., Braun, J. J., Kumar, C., Furian, S., Babic, M., Riotte, J., and Valles, V.: Ferrolysis induced soil transformation by natural drainage in Vertisols of sub-humid South India, Geoderma, 156, 173–188, https://doi.org/10.1016/j.geoderma.2010.02.014, 2010.
Bardgett, R. D.: The Biology of Soil, Oxford University Press, Oxford, UK, 2005.
Batjes, N. H.: Total carbon and nitrogen in the soils of the world, Eur. J. Soil Sci., 47, 151–163, https://doi.org/10.1111/j.1365-2389.1996.tb01386.x, 1996.
Batjes, N. H.: Carbon and nitrogen stocks in the soils of Central and Eastern Europe, Soil Use Manage., 18, 324–329, https://doi.org/10.1111/j.1475-2743.2002.tb00248.x, 2002.
Batjes, N. H.: Mapping soil carbon stocks of Central Africa using SOTER, Geoderma, 146, 58–65, https://doi.org/10.1016/j.geoderma.2008.05.006, 2008.
Bernoux, M. and Chevallier, T.: Le carbone dans les sols des zones sèches. Des fonctions multiples indispensables, Les dossiers thématiques du CSFD, No. 10, CSFD/Agropolis International, Montpellier France, 2013.
Bird, J. A. and Torn, M. S.: Fine roots vs. needles: A comparison of 13C and 15N dynamics in a ponderosa pine forest soil, Biogeochemistry, 79, 361–382, https://doi.org/10.1007/s10533-005-5632-y, 2006.
Boulmane, M., Makhloufi, M., Bouillet, J. P., Badr Satrani, L. S. A., Halim, M., and Elantry-Tazi, S.: Estimation du stock de carbone organique dans la chênaie verte du Moyen Atlas marocain, Acta Bot. Gallica, 157, 451–467, https://doi.org/10.1080/12538078.2010.10516222, 2010.
Bray, R. H. and Kurtz, L. T.: Determination of total organic and available forms of phosphorus in soils, Soil Sci., 59, 39–46, https://doi.org/10.1097/00010694-194501000-00006, 1945.
Bremner, J. M.: Nitrogen Total, in: Methods of Soil Analysis Part 3: Chemical Methods, edited by: Sparks, D. L., SSSA Book Series 5, Soil Science Society of America, Madison, Wisconsin, 1085–1122, 1996.
Briedis, C., de Moraes Sá, J. C., Caires, E. F., de Fátima Navarro, J., Inagaki, T. M., Boer A., Neto, C. Q., de Oliveira Ferreira, A., Canalli, L. B., and dos Santos, J. B.: Soil organic matter pools and carbon-protection mechanisms in aggregate classes influenced by surface liming in a no-till system, Geoderma, 170, 80–88, https://doi.org/10.1016/j.geoderma.2011.10.011, 2012.
Brinkman, R.: Ferrolysis, a hydromorphic soil forming process, Geoderma, 3, 199–206, https://doi.org/10.1016/0016-7061(70)90019-4, 1970.
Brown, T. T. and Huggins, D. R.: Soil carbon sequestration in the dryland cropping region of the Pacific Northwest, J. Soil Water Conserv., 67, 406–415, https://doi.org/10.2489/jswc.67.5.406, 2012.
Carter, M.: Relationship of strength properties to bulk density and macroporosity in cultivated loamy sand to loam soils, Soil Till. Res., 15, 257–268, https://doi.org/10.1016/0167-1987(90)90082-O, 1990.
Chen, A., Wang, Z., Lin, Y., Wang, X., Li, Y., Zhang, Y., Tao, Z., Gao, Q., and Tang, G.: Temporal variation of soil organic carbon pools along a chronosequence of reforested land in Southwest China, Catena, 194, 104650, https://doi.org/10.1016/j.catena.2020.104650, 2020.
Chenu, C., Angers, D. A., Barré, P., Derrien, D., Arrouays, D., and Balesdent, J.: Increasing organic stocks in agricultural soils: knowledge gaps and potential innovations, Soil Till. Res., 188, 41–52, https://doi.org/10.1016/j.still.2018.04.011, 2019.
Ciais, P., Bombelli, A., Williams, M., Piao, S. L., Chave, J., Ryan, C. M., Henry, M., Brender P., and Valentini R.: The carbon balance of Africa: synthesis of recent research studies, Philos. T. R. Soc. A, 369, 2038–2057, https://doi.org/10.1098/rsta.2010.0328, 2011.
Cools, N., Vesterdal, L., De Vos, B., Vanguelova, E., and Hansen, K.: Tree species is the major factor explaining C : N ratios in European forest Soils, Forest Ecol. Manage., 311, 3–16, https://doi.org/10.1016/j.foreco.2013.06.047, 2013.
da Silva, L. F., Fruett, T. Zinn, Y. L., Inda, A. V., and do Nascimento, P. C.: Genesis, morphology and mineralogy of Planosols developed from different parent materials in southern Brazil, Geoderma, 341, 46–58, https://doi.org/10.1016/j.geoderma.2018.12.010, 2019.
De Vos, B., Cools, N., Ilvesniemi, H., Vesterdal, L., Vanguelova, E., and Carnicelli, S.: Benchmark values for forest soil carbon stocks in Europe: results from a large scale forest soil survey, Geoderma, 251–252, 33–46, https://doi.org/10.1016/j.geoderma.2015.03.008, 2015.
Driessen, P., Deckers, J., Spaargaren, O., and Nachtergaele, F.: Lecture notes on the major soils of the World. World Soil Resources Report 94, FAO, Rome, 2001.
Eswaran, H., Den Berg, E. V., and Reich, P.: Organic carbon in soils of the world, Soil Sci. Soc. Am. J., 57, 192–194, https://doi.org/10.2136/sssaj1993.03615995005700010034x, 1993.
FAO: Carbon Sequestration in Dryland Soils, World Soil Resources Reports 102, FAO, Rome, Italy, 2004.
FAO: Soil Organic Carbon: the hidden potential, Food and Agriculture Organisation of the United Nations, Rome, 2017.
Fernandez, J. M., Plaza, C., Garcia-Gil, J. C., and Polo, A.: Biochemical properties and barley yield in a semiarid Mediterranean soil amended with two kinds of sewage sludge, Appl. Soil Ecol., 42, 18–24, https://doi.org/10.1016/j.apsoil.2009.01.006, 2009.
Fioretto, A., Di Nardo, C., Papa, S., and Fuggi, A.: Lignin and cellulose degradation and nitrogen dynamics during decomposition of three leaf litter species in a Mediterranean ecosystem, Soil Biol. Biochem., 37, 1083–1091, https://doi.org/10.1016/j.soilbio.2004.11.007, 2005.
Gallardo, A., Rodríguez-Saucedo, J., Covelo, F., and Fernández-Ales, R.: Soil nitrogen heterogeneity in dehesa ecosystem, Plant Soil, 222, 71–82, https://doi.org/10.1023/A:1004725927358, 2000.
González, J. and Candás, M.: Materia orgánica de suelos bajo encinas: mineralización de carbono y nitrógeno, Invest. Agrar., 3, 75–83, 2004.
Gountié Dedzo, M., Asaah, A. N. E., Fozing, E. M., Tchamabé, B. C., Zangmo, G. T., Dagwai, N., Seuwui, D. T., Kamgang, P., Aka, F. T., and Ohba, T.: Petrology and geochemistry of lavas from Gawar, Minawao and Zamay volcanoes of the northern segment of the Cameroon volcanic line (Central Africa): Constraints on mantle source and geochemical evolution, J. Afr. Earth Sci., 153, 31–41, https://doi.org/10.1016/j.jafrearsci.2019.02.010, 2019.
Gray, J. M., Bishop, T. F. A., and Wilson, R. B.: Factors controlling soil organic carbon with deph in Eastern Australia, Soil Sci. Soc. Am., 79, 1741–1751, https://doi.org/10.2136/sssaj2015.06.0224, 2016.
Greiner, L., Keller, A., Grêt-Regamey, A., and Papritz, A.: Soil function assessment: review of methods for quantifying the contributions of soils to ecosystem services, Land Use Pol., 69, 224–237, https://doi.org/10.1016/j.landusepol.2017.06.025, 2017.
Guitian, F. and Carballas, T.: Tecnicas de Analisis de suelos, 2, a ed. Pico Sacro, Santiago de Compostela, 1976.
Haygarth, P. and Ritz, K.: The future of soils and land use in the UK: soil systems for the provision of land-based ecosystem services, Land Use Pol., 26, 187–197, https://doi.org/10.1016/j.landusepol.2009.09.016, 2009.
Hiederer, R.: Distribution of Organic Carbon in Soil Profile Data, EUR 23980 EN, Office for Official Publications of the European Communities, Luxembourg, https://doi.org/10.2788/33102, 2009.
Hounkpatin, O. K. L., Op de Hipt, F., Bossa, A. Y., Welp, G., and Amelung, W.: Soil organic carbon stocks and their determining factors in the Dano catchment (Southwest Burkina Faso), Catena, 166, 298–309, https://doi.org/10.1016/j.catena.2018.04.013, 2018.
Ibrahim, A. and Habib, F.: Estimation du stock de carbone dans les faciès arborées er shrubs des savanes soudano guinéennes de Ngaoundéré, Cameroun, Cameroun J. Exp. Biol., 4, 1–11, https://doi.org/10.4314/cajeb.v4i1.37970, 2008.
IPCC: Supplementary methods and good practice guidance arising from the Kyoto Protocol, in: Intergovernmental Panel on Climate Change: Good Practice Guidance for Land Use, edited by: Penman, J., Gytarsky, M., Hiraishi, T., Krug, T., Kruger, D., Pipatti, R., Buendia, L., Miwa, K., Ngara, T., Tanabe, K., Wagner, F., Land Use Change and Forestry, IPCC/OECD/IEA/IGES, Hayama, Japan, 2003.
IUSS Working Group WRB: World Reference Base for Soil Resources 2014, update 2015, International soil classification system for naming soils and creating legends for soil maps, World Soil Resources Reports No. 106, FAO, Rome, 2015.
Jenny, H.: A study on the influence of climate upon the nitrogen and organic matter content of soils. University of Missouri College of Agriculture, Columbia, Res. Bull., 152, 1–67, 1930.
Jiang, R., Gunina, A., Qu, D., Kuzyakov, Y., Yu, Y., Hatano, R., Frimpongi, K. A., and Li, M.: Afforestation of loess soils: Old and new organic carbon in aggregates and density fractions, Catena, 177, 49–56, https://doi.org/10.1016/j.catena.2019.02.002, 2019.
Jiménez-González, M. A., Álvarez, A. M., Carral, P., and Almendros, G.: Influence of soil forming factors on the molecular structure of soil organic matter and carbon levels, Catena, 189, 104501, https://doi.org/10.1016/j.catena.2020.104501, 2020.
Jones, A., Breuning Madsen, H., Brossard, M., Dampha, A., Deckers, J., Dewitte, O., Gallali, T., Hallett, S., Jones, R., Kilasara, M., Le Roux, P., Micheli, E., Montanarella, L., Spaargaren, O., Thiombiano, L., Van Ranst, E., Yemefack, M., and Zougmore', R. (Eds.): Soil Atlas of Africa, European Commission, Publications Office of the European Union, Luxembourg, 2013.
Lal, R.: Carbon sequestration in dryland ecosystems, Environ. Manage., 33, 528–544, https://doi.org/10.1007/s00267-003-9110-9, 2004.
Lal, R.: Challenges and opportunities in soil organic matter research, Eur. J. Soil Sci., 60, 158–169, https://doi.org/10.1111/j.1365-2389.2008.01114.x, 2009.
Lehmann, J. and Kleber, M.: The contentious nature of soil organic matter, Nature, 528, 60–68, https://doi.org/10.1038/nature16069, 2015.
Letouzey, R.: Notice explicative de la carte phytogéographique du Cameroun au 1/500000, Institut de la Carte Internationale de la Végétation, Toulouse, France, 1985.
Liu, Z.-P., Shao, M.-A., and Wang, Y.-Q.: Estimating soil organic carbon across a large-scale region: a state-space modeling approach, Soil Sci., 177, 607–618, https://doi.org/10.1097/SS.0b013e318272f822, 2012.
Lozano-García, B., Muñoz-Rojas, M., and Parras-Alcántara, L.: Climate and land use changes effects on soil organic carbon stocks in a Mediterranean semi-natural area, Sci. Total Environ., 579, 1249–1259, https://doi.org/10.1016/j.scitotenv.2016.11.111, 2017.
Mabicka, O. R. G., Musadji, N.-Y., Ndongo, A., Soumaho, J., Mouha, E. D.-L., Abaker, M. G., Ondo, J. A., Ravire, E., and Mbina, M. M.: Carbon and nitrogen stocks under various land cover in Gabon, Geoderma Reg., 25, e00363, https://doi.org/10.1016/j.geodrs.2021.e00363, 2021.
Maestre, F. T., Escolar, C., Ladron de Guevara, M., Quero, J. L., Lazaro, R., Delgado-Baquerizo, M., Ochoa, V., Berdugo, M., Gozalo, B., and Gallardo, A.: Changes in biocrust cover drive carbon cycle responses to climate change in drylands, Glob. Change Biol., 19, 3835–3847, https://doi.org/10.1111/gcb.12306, 2013.
Mayer, S., Kühnel, A., Burmeister, J., Kögel-Knabner, I., and Wiesmeier, M.: Controlling factors of organic carbon stocks in agricultural topsoils and subsoils of Bavaria, Soil Till. Res., 192, 22–32, https://doi.org/10.1016/j.still.2019.04.021, 2019.
Moustakas, N. K.: A study of vertisol genesis in North Eastern Greece, Catena, 92, 208–215, https://doi.org/10.1016/j.catena.2011.12.011, 2012.
Muneer, M. and Oades, J. M.: The role of Ca–organic interactions in soil aggregate stability: III. Mechanisms and models, Aust. J. Soil Sci., 27, 411–423, https://doi.org/10.1071/SR9890411, 1989.
Muñoz-Rojas, M., Jordán, A., Zavala, L. M., De la Rosa, D., Abd-Elmabod, S. K., and Anaya-Romero, M.: Organic carbon stocks in Mediterranean soil types under different land uses (Southern Spain), Solid Earth, 3, 375–386, https://doi.org/10.5194/se-3-375-2012, 2012.
Muñoz-Rojas, M., Jordán, A., Zavala, M., de la Rosa, D., Abd-Elmabod, S. K., and Anaya-Romero, M.: Impact of land use and land cover changes on organic carbon stocks in mediterranean soils (1956–2007), Land Degrad. Dev., 26, 168–179, https://doi.org/10.1002/ldr.2194, 2015.
Ngounouno, I., Durelle, B., and Demaiffe, R.: Petrology of the Biomodal Cenozoic Volcanism of the Kapsiki plateau Northern most Cameroun, Central Africa, J. Volcanol. Geoth. Res., 12, 21–44, https://doi.org/10.1016/S0377-0273(00)00180-3, 2000.
Nguetnkam, J. P.: “Clays of Vertisols and fersiallitic Soils of Far North Cameroon: Genesis, Crystallochemical and Textural Properties, Typology and Application in Vegetable Oil Discolouration”, Thèse Doct. d'Etat, University of Yaoundé 1, Yaoundé, Cameroon, 2004.
Nyameasem, J. K., Reinsch, T., Taube, F., Domozoro, C. Y. F., Marfo-Ahenkora, E., Emadodin, I., and Malisch, C. S.: Nitrogen availability determines the long-term impact of land use change on soil carbon stocks in grasslands of southern Ghana, SOIL, 6, 523–539, https://doi.org/10.5194/soil-6-523-2020, 2020.
O'Geen, A. T., Hobson, W. A., Dahlgren, R. A., and Kelley, D. B.: Evaluation of soil properties and hydric soil indicators for vernal pool catenas in California, Soil Sci. Soc. Am. J., 72, 727–740, https://doi.org/10.2136/sssaj2007.0123, 2008.
Olson, K. R. and Al-Kaisi, M. M.: The importance of soil sampling depth for accurate account of soil organic carbon sequestration, storage, retention and loss, Catena, 125, 33–37, https://doi.org/10.1016/j.catena.2014.10.004, 2015.
Olson, K. R., Al-Kaisi, M., Lal, R., and Cihacek, L.: Impact of soil erosion on soil organic carbon stocks, J. Soil Water Conserv., 71, 61A–67A, https://doi.org/10.2489/jswc.71.3.61A, 2016.
Parras-Alcantara, L., Lozano-García, B., Brevik, E. C., and Cerda, A.: Soil organic carbon stocks assessment in Mediterranean natural areas: A comparison of entire soil profiles and soil control sections, J. Environ. Manage., 155, 219–228, https://doi.org/10.1016/j.jenvman.2015.03.039, 2015.
Pineiro, G., Paruelo, J. M., Oesterheld, M., and Jobbagy, E. G.: Pathways of grazing effects on soil organic carbon and nitrogen, Rangeland Ecol. Manag., 63, 109–119, https://doi.org/10.2111/08-255.1, 2010.
Plaza, C., Gascó, G., Méndez, A. M., Zaccone, C., and Maestre, F. T.: Chapter 2 – Soil Organic Matter in Dryland Ecosystems, in: The Future of Soil Carbon, 39–70, https://doi.org/10.1016/B978-0-12-811687-6.00002-X, Academic Press, Elsevier, 2018.
Post, W. M., Emanuel, W. R., Zinke, P. J., and Stangenberger, A. G.: Soil carbon pools and world life zones, Nature, 298, 156–159, https://doi.org/10.1038/298156a0, 1982.
Rahman, M. T., Guo, Z. C., Zhang, Z. B., Zhou, H., and Peng, X. H.: Wetting and drying cycles improving aggregation and associated C stabilization differently after straw or biochar incorporated into a Vertisol, Soil Till. Res., 175, 28–36, https://doi.org/10.1016/j.still.2017.08.007, 2018.
Reyna-Bowena, L., Fernandez-Rebollob, P., Fernández-Habas, J., and Gómez, J. A.: The influence of tree and soil management on soil organic carbon stock and pools in dehesa systems, Catena, 190, 104511, https://doi.org/10.1016/j.catena.2020.104511, 2020.
Ruiz Sinoga, J. D., Pariente, S., Romero Diaz, A., and Murillo, J. F. M.: Variability of relationships between soil organic carbon and some soil properties in Mediterranean rangelands under different climatic conditions (South of Spain), Catena, 94, 17–25, https://doi.org/10.1016/j.catena.2011.06.004, 2012.
Sakin, E.: Relationships between of carbon, nitrogen stocks and texture of the Harran Plain soils in southeastern Turkey, Bulg. J. Agric. Sci., 18, 626–634, 2012.
Schaefer, M. V., Bogie, N. A., Rath, D., Marklein, A. R., Garniwan, A., Haensel, T., Lin, Y., Avila, C. C., Nico, P. S., Scow, K. M., Brodie, E. L., Riley, W. J., Fogel, M. L., Berhe, A. A., Ghezzehei, T. A., Parikh, S., Keiluweit, M., and Ying, S. C.: Effect of Cover Crop on Carbon Distribution in Size and Density Separated Soil Aggregates, Soil Syst., 4, 6, https://doi.org/10.3390/soilsystems4010006, 2020.
Singh, L. P., Parkash, B., and Singhvi, A. K.: Evolution of the Lower Gangetic Plain landforms and soils in West Bengal, India, Catena, 33, 75–104, https://doi.org/10.1016/S0341-8162(98)00066-6, 1998.
Soleimani, A., Hosseini, S. M., Bavani, A. R. M., Jafari, M., and Francaviglia, R.: Influence of land use and land cover change on soil organic carbon and microbial activity in the forests of northern Iran, Catena, 177, 227–237, https://doi.org/10.1016/j.catena.2019.02.018, 2019.
Spiau, P. and Pedro, G.: Experimental studies of the ferrolysis process production of exchange acidity and demonstration of catalytic role of the clay minerals, Sci. Sol., 1, 43–44, 1986.
Tamen, J., Nkoumbou, C., Reusser, E., and Tchoua, F.: Petrology and geochemistry of mantle xenoliths from the Kapsiki Plateau (Cameroon Volcanic Line): Implications for lithospheric upwelling, J. Afr. Earth Sci., 101, 119–134, https://doi.org/10.1016/j.jafrearsci.2014.09.008, 2015.
Tang, Q., Han, W., Li, X., and Wang, G.: Clarifying the response of soil organic carbon storage to increasing temperature through minimizing the precipitation effect, Geoderma, 374, 114398, https://doi.org/10.1016/j.geoderma.2020.114398, 2020.
Tchobsala, Mbolo, M., and Souare, K.: Impact of wood logging on the phytomass and carbon sequestration in the guinea savanna of Ngaoundéré, Adamaoua Region, Cameroon, Global Advanced Research Journal of Environmental Science and Toxicology, 3, 38–48, 2014.
Tchobsala, Megueni, C., Souare, K., Njintang Yanou, N., Sadou, I., Patrick, P., and Palou, O.: Techniques of Domestication and Carbon Sequestration of Jatropha curcas Associated with Vigna unguiculata in Marginal Soil of Garoua Region, Cameroon, J. Life Sci., 10, 245–259, https://doi.org/10.17265/1934-7391/2016.05.007, 2016.
Torn, M. S., Swanston, C. W., Castanha, C., and Trumbore, S. E.: Storage and turnover of organic matter in soil, in: Biophysicochemical Processes Involving Natural Nonliving Organic Matter in Environmental Systems, edited by: Nicola Senesi, N., Xing, B., and Huang, P. M., John Wiley and Sons, Inc, 219–272, 2009.
Tornquist, C. G., Giasson, E., Mielniczuk, J., Cerri, C. E. P., and Bernoux, M.: Soil organic carbon stocks of Rio Grande do Sul, Brazil, Soil Sci. Soc. Am. J., 73, 975–982, https://doi.org/10.2136/sssaj2008.0112, 2009.
Tsao, C.-H.: Carbon sequestration under different land uses and soils in the state of Quintana Roo, Mexico, Master of Science Thesis, TH Köln – University of Applied Sciences, Institute for Technology and Resources Management in the Tropics and Subtropics, 2017.
Tsozué, D., Haiwe, B. R., Louleo, J., and Nghonda, J. P.: Local Initiatives of Land Rehabilitation in the Sudano-Sahelian Region: Case of Hardé Soils in the Far North Region of Cameroon, Open J. Soil Sci., 4, 6–15, https://doi.org/10.4236/ojss.2014.41002, 2014.
Tsozué, D., Nghonda, J. P., Tematio, P., and Djakba Basga, S.: Changes in soil properties and soil organic carbon stocks along an elevation gradient at Mount Bambouto, Central Africa, Catena, 175, 251–262, https://doi.org/10.1016/j.catena.2018.12.028, 2019.
Tuttle, C. L., Golden, M. S., and Meldahl, R. S.: Soil compaction effects on Pinus taeda establishment from seed and early growth, Can. J. Forest Res., 18, 628–632, https://doi.org/10.1139/x88-092, 1988.
USDA: Soil Survey Laboratory Methods Manual, Soil Survey Investigation Report No. 42, Version 4.0. USDA-NCRS, Lincoln, NE, 2004.
Van Breemen, N. and Buurman, P. (Eds.): The formation of hydromorphic soils, in: Soil Formation, Kluwer Academic Publishers, Dordrecht, 159–192, 2002.
Van Ranst, E., Dumon, M., Tolossa, A. R., Cornelis, J. T., Stoops, G., Vandenberghe, R. E., and Deckers, J.: Revisiting ferrolysis processes in the formation of Planosols for rationalizing the soils with stagnic properties in WRB, Geoderma, 163, 265–274, https://doi.org/10.1016/j.geoderma.2011.05.002, 2011.
Vepraskas, M. J.: Morphological features of seasonally reduced soils, in: Wetland Soils: Genesis, Hidrology, Landscapes and Classification, edited by: Richardson, J. L. and Vepraskas, M. J., Lewis Publishers, Boca Raton, 163–182, 2001.
Von Lutzow, M., Kogel-Knabner, I., Ekschmitt, K., Matzner, E., Guggenberger, G., Marschner, B., Guggenberger, C. G., Marschner, D. B., and Flessa, E. H.: Stabilization of organic matter in temperate soils: mechanisms and their relevance under different soil conditions: a review, Eur. J. Soil Sci., 57, 426–445, https://doi.org/10.1111/j.1365-2389.2006.00809.x, 2006.
Walkley, A. and Black, I. A.: Determination of organic matter in soil, Soil Sci., 37, 549–556, 1934.
Wang, X., Li, Y., Gong, X., Niu, Y., Chen, Y., Shi, X., Li, W., and Liu, J.: Changes of soil organic carbon stocks from the 1980s to 2018 in northern China's agro-pastoral ecotone, Catena, 194, 104722, https://doi.org/10.1016/j.catena.2020.104722, 2020.
Yoh, M.: Soil ratio as affected by climate: an ecological factor of forest NO leaching, Water Air Soil Pollut., 130, 661–666, https://doi.org/10.1023/A:1013860830153, 2001.
Yüksek, T.: The restoration effects of black locust (Robinia pseudoacacia L) plantation on surface soil properties and carbon sequestration on lower hillslopes in the semi-humid region of Coruh Drainage Basin in Turkey, Catena, 90, 18–25, https://doi.org/10.1016/j.catena.2011.10.001, 2012.
Zhang, D., Hui, D., Luo, Y., and Zhou, G.: Rates of litter decomposition in terrestrial ecosystems: global patterns and controlling factors, J. Plant Ecol., 1, 85–93, https://doi.org/10.1093/jpe/rtn002, 2008.
Zhang, X., Guo, J., Vogt, R. D., Mulder, J., Wang, Y., Qian, C., Wang, J., and Zhang, X.: Soil acidification as an additional driver to organic carbon accumulation in major Chinese croplands, Geoderma, 366, 114234, https://doi.org/10.1016/j.geoderma.2020.114234, 2020.
Zinn, Y. L., Marrenjoa, G. J., and Silva, C. A.: Soil C : N ratios are unresponsive to land use change in Brazil: A comparative analysis, Agr. Ecosyst. Environ., 255, 62–72, https://doi.org/10.1016/j.agee.2017.12.019, 2018.