the Creative Commons Attribution 4.0 License.
the Creative Commons Attribution 4.0 License.
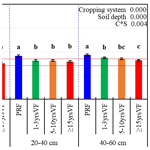
Significant soil degradation is associated with intensive vegetable cropping in a subtropical area: a case study in southwestern China
Ming Lu
David S. Powlson
Yi Liang
Dave R. Chadwick
Shengbi Long
Xinping Chen
Within the context of sustainable development, soil degradation driven by land use change is considered a serious global problem, but the conversion from growing cereals to vegetables is a change that has received limited attention, especially in subtropical regions. Here, we studied the effects of the conversion from paddy rice to an oilseed rape rotation to vegetable production in southwestern China on soil organic carbon (SOC), total nitrogen (TN), the ratio, pH, phosphorus (P), potassium (K), calcium (Ca), and magnesium (Mg) based on face-to-face farmer surveys and soil analysis. In the vegetable cropping system, fertilizer application often exceeds the crop demand or levels recommended by the local extension service several times over. Thus, the crop use efficiency of N, P, K, Ca, and Mg was only 26 %, 8 %, 56 %, 23 %, and 28 %, respectively. In the vegetable cropping system studied, SOC, C stock, TN, and N stock were decreased significantly due to low organic inputs from crop residues and high tillage frequency. Furthermore, the soil ratio decreased slightly; available P (AP) in the topsoil increased by 1.92 mg kg−1 for every 100 kg ha−1 of P surplus, and the critical levels of AP and CaCl2-soluble P in P leaching were 104 and 0.80 mg P kg−1. Besides, compared to the current paddy–rape rotation system, a clear trend of soil acidification was observed in the vegetable fields. However, increasing the contents of soil Ca and Mg significantly alleviated topsoil acidification, with the effect increasing over time. Given our findings, the potential benefits of conservation agricultural practices, integrated soil–crop system management strategies, and agricultural technology services for recovering the degraded soil and improving the vegetable productivity are discussed here.
- Article
(1635 KB) - Full-text XML
- BibTeX
- EndNote
Land use changes are an important anthropogenic perturbation that can cause soil degradation (Lal, 2004, 2015), but the impacts of land conversion from growing cereals to vegetables have received little attention. From 1997to 2017, total vegetable production increased by 98 % worldwide, and the area harvested increased by 59 % (FAO, 2017). These increases were driven by economic growth and an increased demand for vegetables associated with a raised awareness of their health benefits (Yan et al., 2012). The expansion of terrestrial vegetable production is particularly significant in the tropics and subtropics because these regions have a longer growing season compared to temperate regions (Fischer et al., 2012). On average, the sown area of non-grain crops has increased by > 12 % since 1980 in China, with a corresponding decrease in grain crop cultivation (Chen et al., 2016). In contrast to cereal crops, vegetable production requires more intensive management, including higher tillage frequencies and fertilization rates, and higher nutrient and water inputs due to the shallow root systems of most vegetable species (Wang et al., 2018a, b; Yan et al., 2013; Zhang et al., 2017). Thus, vegetable production is associated with a relatively higher risk of environmental pollution. For instance, Zhang et al. (2012) found that the per unit area of nutrient inputs in vegetable production are 0.81–1.20 times greater than those for the cereal production system in China and 1.5–12 times greater than those for vegetable cropping in the rest of the world. In particular, overuse or misuse of fertilizers in vegetable fields is a long-standing problem across the world (Conley et al., 2009).
Soil organic carbon (SOC) is widely regarded to be the most reliable indicator for monitoring soil degradation (Lal, 2015). Bünemann et al. (2018) reviewed soil quality assessment approaches and summarized the indicators used; after SOC, the most frequently used chemical indicators were soil pH, available phosphorus (P), available potassium (K), total nitrogen (TN), and bulk density. Numerous studies have reported on the severity of soil degradation in greenhouse vegetable fields converted from a maize–wheat rotation in the North China Plain due to high fertilizer inputs and the increased frequency of soil cultivation, resulting in a significant decrease in soil ratio and pH, enrichment of P and K, and salinization (Ju et al., 2007; Yan et al., 2012). However, the impacts of land conversion from cereal cropping to vegetable production may be more adverse in tropical and subtropical regions compared to temperate regions due to higher temperatures and rainfall combined, low organic inputs, and the high frequency of tillage practices (National Bureau of Statistics, 2017; Powlson et al., 2016; Sarker et al., 2018). Based on the background information, here we hypothesize that the conversion of cereals to vegetable production would result in significant degradation in agricultural soils in southwestern China.
For instance, in southern China, the paddy soil is normally in areas of long-term flooding, has a relatively higher input of carbon, and receives two deep tillage operations annually (Wang et al., 2014). In contrast, the vegetable fields will be tilled three to four times per year, resulting in severe disturbances in the surface soil layer (0–20 cm). Wang et al. (2014) also found that the SOC content and the ratio in open-field vegetable systems converted from paddy fields were decreased by 19.7 % and 27.8 %, respectively, which was mainly attributable to soil aggregate fragmentation. However, there is no information available on the effects of land use changes, from cereals to vegetable production, on the status of SOC and TN in southwestern China.
Additionally, high inputs of N fertilizers to vegetable crops and the crop removal of base cations, such as calcium (Ca) and magnesium (Mg), are expected to trigger soil acidification (Guo et al., 2010; Zhang et al., 2016). Nevertheless, according to our preliminary farmer survey in southwestern China, agricultural production commonly received large inputs of Ca and Mg from various fertilizers. Moreover, increasing research is being done to overcome crop yield gaps by ameliorating soil micronutrient status (White and Broadley, 2009; Cakmak and Yazici, 2010). To date, the combined impacts of these inputs and the removal of base cations on soil pH and other properties in vegetable production systems have not been studied.
Systematic surveys to elucidate the effects of different cropping systems on soil properties are needed for a clear understanding of the impacts of vegetable production and the proper development of sustainable, high-yield vegetable systems that do not cause environmental damage. The objectives of this study were to assess the impacts of the land use conversion from a paddy–rape rotation to vegetable production on soil pH, SOC, TN, the soil ratio, available P (AP), CaCl2-soluble P (CaCl2-P), and available base cations (K, Ca, and Mg). In the present study, paddy rice–oilseed rape rotation fields were used as a reference because all vegetable fields in this region were converted from paddy soil. This will allow the comparison between the conditions in areas that changed to open-field vegetable production with surrounding areas that have remained under paddy rice–oilseed rape rotations.
2.1 Site descriptions and cropping systems
The field study sites were located in the Jinping county in the eastern Guizhou province at 26∘23′–26∘46′ N and 108∘48′–109∘24′ E (Fig. 1a). In this area, vegetables are produced on 4814 ha, representing an increase of 24 % between 2009 and 2015, and the production increased by 132 % between 2011 and 2018 (84 886 t). The area covered by cereal crops (14 408 ha at the time of our study) decreased by 9 % from 2009 to 2018 (official statistics from http://www.jinping.gov.cn/xxgk/zdgk/tjxx/tjgb/; last access: 1 April 2020). The region is characterized by a typical subtropical humid monsoon climate with an annual average precipitation of 1160 mm for the 2007–2017 period, of which 85 % occurs between April and October, and a mean annual air temperature of 15.9 ∘C (Fig. 1b). The highest temperatures and rainfall occur in the same season. The main type of soil in this region is a typical Ultisol with a loamy clay texture (with an average content of sand 11.5 %, silt 43.5 %, and clay 45 %) and alluvial parent material based on the USDA (United States Department of Agriculture) soil classification system. The soil depth is generally > 90 cm. The main crops grown are open-field vegetables, paddy rice, oilseed rape, and fruit trees.
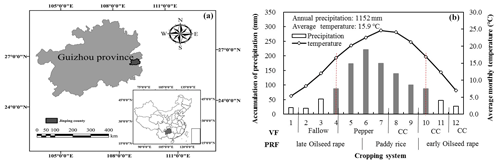
Figure 1The location (a), meteorological conditions (values averaged between 2007 and 2017), and typical cropping systems (b) of the study area. CC – non-heading Chinese cabbage; VF – vegetable production; PRF – paddy rice–oilseed rape rotation.
Open-field vegetable production accounts for 70 %–80 % of the total area of dryland agricultural production, and the most common planting pattern (71 % of the total vegetable fields) is a pepper–Chinese cabbage rotation system. In this cropping system, peppers are transplanted in April and harvested in mid-August. Then, non-heading Chinese cabbage is grown continually for three seasons, from August to January of the following year, followed by a fallow period until April (Fig. 1b). During the annual rainfed cropping period, tillage is performed four times (mainly tilled with a mini-sized fuel-rotary cultivator at the depth of ca. 20 cm in the soil layer), and fertilizers are applied 10 times (four times for pepper and twice for each Chinese cabbage crop). Conventional farming practices commonly used throughout the region were used for all crops. In the paddy rice–oilseed rape double-cropping system, paddy rice is grown from late May to September, and then oilseed rape is grown from mid-October to early May of the following year.
2.2 Farmer surveys and soil sampling
In this study, the focus was only on the typical vegetable production (pepper – Chinese cabbage – Chinese cabbage – Chinese cabbage – fallow rotation) and the paddy rice–oilseed rape rotation. A survey of management practices was conducted through face-to-face interviews with farmers in Jinping county in Guizhou province. Based on the farmers' survey methodology (Jia et al., 2013), two of the most important vegetable production townships in Jinping county, Xinhua and Dunzhai, were selected in this area. In each township, four villages were randomly selected, and 15–20 farmers from each village who had managed paddy–rape rotation before switching to the vegetable cropping system were randomly surveyed. Survey questions were related to crop varieties and the management of the two cropping systems, including sowing rate, fertilizer (chemical or organic) application rate, tillage frequency, crop residue management, crop growth cycle, cropping duration, and yield. A total of 24 surveys were related to paddy–rape rotation management and 133 surveys for vegetable production. The field sizes of the paddy–rape rotation and vegetable cropping were 0.03–0.13 and 0.01–0.08 ha, respectively.
To investigate the effect of conventional fertilization on nutrient surplus, enrichment and/or depletion, and leaching them into the soil profile of vegetable field, a multistage sampling technique was used to select representative fields for sampling soils from each cropping system. First, we randomly collected 36 soil samples from a total of 133 commercial vegetable fields that were converted from paddy–rape rotation 1–3 years (n=12), 5–10 years (n=12), and ≥15 years (n=12) ago. Second, 12 paddy–rape rotation fields and the adjacent (<100 m) vegetable fields were also randomly sampled for comparison. The soil type of the selected sites and the local/regional climatic conditions and agricultural production practices were similar (Ju et al., 2017; Yan et al., 2012). Soil samples were collected from six spots at 20 cm depth intervals to a depth of 60 cm within each plot, and they were pooled by depth. Samples were collected at all selected vegetable and paddy fields from 25 to 31 September 2018, after the crops (i.e., paddy rice and Chinese cabbage) were harvested (Fig. 1b). In addition, three other soil cores (diameter 50.46 mm; length 200 mm) were collected randomly from each field type for bulk density. The bulk density was measured using the cutting ring method. Each single soil sample in this study incorporates 20 cm soil depth.
2.3 Soil analysis
In this study, the moist composite field samples were air dried and passed through a 1 mm sieve for the analysis of soil pH, CaCl2-P, available P (AP), water-soluble K, Ca, and Mg and exchangeable K, Ca, and Mg. The samples were then passed through a 0.15 mm sieve for SOC and TN analysis (Yan et al., 2012; Wang et al., 2014; Gómez et al., 2020). Soil analysis methods for specific parameters and their corresponding soil size preparation standards (described previously) were used in this study (Lu, 2000). Soil pH in water with a 1 : 2.5 soil-to-water ratio was measured using a potentiometer. SOC was measured using ferric sulfate titration after dichromate digestion. Soil TN was measured by the semi-automatic Kjeldahl method after sulfuric acid digestion. Soil AP (Bray-P) content was measured by the molybdovanado phosphate method following soil extraction with 0.03 mol L−1 NH4F and 0.025 mol L−1 HCl and using a 1 : 10 ratio () of soil to solution. CaCl2 at 0.01 mol L−1 and a 1 : 5 soil to solution ratio were used to determine the soil CaCl2-P content using a UV-visible spectrophotometer. Soil-water-soluble K, Ca, and Mg were determined after extraction with water (soil-to-water ratio of 1 : 5), and exchangeable K, Ca, and Mg were extracted with 1 mol L−1 neutral NH4OAc (, 1 : 12.5) and measured using an ICP-OES (inductively coupled plasma optical emission spectroscopy).
2.4 Calculation of nutrient inputs and balance
Detailed records were made for the farm management practices employed in each field from 2017 to 2018, and we confirmed that the management history of each field was similar to the management practices employed during the survey year. The main mineral fertilizers used in this region were urea, potassium sulfate, compound or mixed fertilizers, calcium superphosphate and fused calcium–magnesium phosphate. Organic fertilizers included crop residues and commercial organic fertilizer, animal manure (from chickens, cows, and pigs and rapeseed meal). In the paddy–rape rotation, rice straw was directly returned after the grains were harvested, whereas oilseed rape straw was burned in the fields. Most above- and belowground residues in the vegetable production were removed during harvest. Besides, nutrient removal due to grain harvest or the removal of aboveground crop parts was calculated using yields reported by farmers and the published nutrient contents typical of the region. Nutrient surplus was calculated by subtracting nutrient removal through grain and vegetable harvest from total fertilizer inputs (Ju et al., 2007).
2.5 Calculation of SOC and TN stock, accumulation of P surplus, and changes in soil AP
The SOC and TN stocks in the paddy–rape rotation and vegetable fields were calculated by multiplying SOC and TN contents with bulk density and depth. Accumulation of P surplus was calculated as the annual P surplus multiplied by years since conversion to vegetable production, and changes in soil AP in vegetable field were calculated as soil AP in paddy–rape field subtracted from that in the vegetable field.
2.6 Statistical analysis
SPSS software (IBM SPSS Statistics for Windows, version 21.0; IBM Corporation, Armonk, NY, USA) was used for statistical analysis. A two-way repeated measures analysis of variance (ANOVA) was performed to assess the effects of cropping systems, soil depth, and their interactions on soil properties. Differences in soil nutrient contents and stock among cropping systems were assessed using one-way ANOVA, and mean values were compared using the least significant difference test with a significance level of 5 %. Correlations were analyzed using a two-tailed Pearson test with a significance level of 5 %. The linear-plateau model in SAS (version 9.2 for Windows; SAS Institute, Cary, NC, USA) was used to analyze the relationship between SOC and tillage frequency in the 0–20 cm surface layer in vegetable soil, and the two-segment linear model in SigmaPlot (version 12.5 for Windows; Systat Software, Inc, San Jose, CA, USA) was used to analyze the relationship between soil AP and CaCl2-P.
3.1 Yields, fertilizer application, and nutrient balance
Total annual inputs of N, P, Ca, and Mg in the vegetable cropping were several times higher than those in the paddy–rape rotation (2.38, 2.97, 4.40 and 7.14 times, respectively), but the total annual K inputs were similar (Table 1). K, Ca, and Mg inputs were mainly from organic sources in the paddy–rape rotation, i.e., a combination of organic fertilizers and crop residues, representing 65 %, 80 %, and 65 % of the total inputs, respectively, while chemical fertilizer supplied 61 % and 72 % of total N and P inputs. In contrast, in the vegetable production, these nutrients were mainly supplied by chemical fertilizers, which comprised 75 %, 86 %, 71 %, 75 %, and 79 % of the total inputs for N, P, K, Ca, and Mg, respectively. Fertilizer application rates did not differ significantly in the vegetable system according to the time since conversion from paddy–rape rotation, and its yields also did not differ significantly (Table 1).
Table 1Average annual nutrient inputs, outputs, and surplus in paddy rice–oilseed rape rotation, and vegetable production converted from paddy–rape rotation 1–3, 5–10, or ≥ 15 years ago in southwestern China. Values are means ± standard deviation.
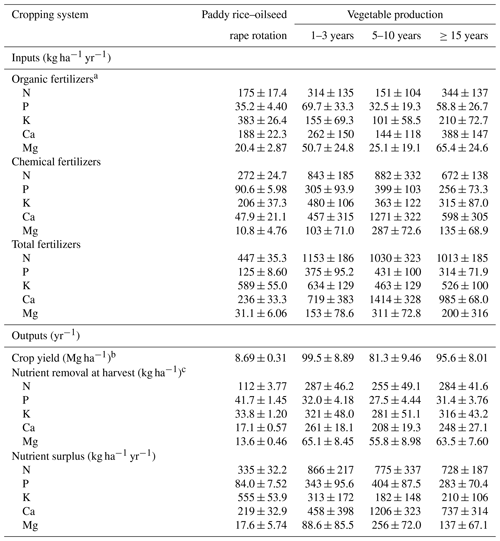
a Organic fertilizers included crop residues, commercial organic fertilizers, animal manure (from chickens, cows, and pigs), and rapeseed meal. In the paddy–rape rotation, rice straw was directly returned after the grains were harvested, whereas oilseed rape straw was burned in the fields. Most above- and belowground residues in the vegetable production were removed during harvest. b Vegetable yields are expressed as the annual sum of fresh weights per season, and yields in the paddy–rape rotation are expressed as the annual sum of air-dried weights per season. c Only grains were harvested in the paddy–rape rotation and most of the above- and belowground residues were returned to the fields. All vegetable parts were removed from the fields during harvest.
In the paddy–rape rotation crop, straw was returned to the field after grain harvest, while all plant biomass was completely removed during harvest in the vegetable cropping. Consequently, 2.46, 9.05, 14.0, and 4.52 times more N, K, Ca, and Mg were removed, respectively, from the vegetable field than that of the paddy–rape, but the P removal rate was slightly lower. Large surpluses of N (790 kg N ha−1 yr−1), P (343 kg P ha−1 yr−1), K (235 kg K ha−1 yr−1), Ca (800 kg Ca ha−1 yr−1), and Mg (161 kg Mg ha−1 yr−1) were observed in the vegetable field (Table 1).
3.2 Changes in SOC and soil TN
The contents (or value) of SOC, TN, the ratio, and C and N stocks were compared between the two cropping systems (Table 2). Conversion from paddy–rape rotation to open-field vegetable led to a significant decrease in both TN content and stock in the topsoil (0–20 cm), with mean decreases of 15.8 % and 17.6 % (P<0.05), respectively, while no significant changes in TN in the two deeper soil layers (20–60 cm) were observed in the vegetable field. However, intensive vegetable production led to a significant decrease in topsoil SOC content and stock, even after only 1–3 years. The C stock in the 0–20 cm soil layer decreased by 10.3 % after 1–3 years and increased to 24.3 % after 5–10 years. Similar trends were observed in the 20–40 and 40–60 cm soil layers. Because proportional decreases in SOC were slightly greater than those for TN, the soil ratio became slightly smaller following the conversion of paddy–rape field to vegetable field, with the trend most apparent in the 40–60 cm soil layer. The ratio, however, decreased by 24.3 % after 5–10 years.
Table 2Soil organic carbon (SOC) and total nitrogen (TN) contents, soil ratio, C and N stocks, and soil bulk density in the 0–20, 20–40, and 40–60 cm soil layers in paddy rice–oilseed rape rotation and vegetable production converted from paddy–rape rotation 1–3, 5–10, or ≥15 years ago in southwestern China.
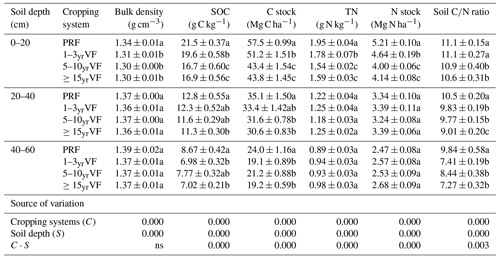
Values (± standard deviation) were averaged over 12 replicates. The least significant difference test was performed to compared values in each soil layer, and different lowercase letters indicate significant differences at P<0.05 among cropping systems. ANOVA results are shown at the bottom. Note: ns – no significant difference; PRF – paddy rice–oilseed rape rotation; VF – vegetable production.
The average bulk density in the topsoil tended to decrease upon the land use conversion but did not change significantly following years of vegetable production. On average, C stock in the 0–60 cm soil layer over all vegetable fields was 16.4 % (P<0.05) less than that in the paddy–rape rotation, with values ranging between 97.8 and 117 Mg C ha−1 yr−1.
One major reason for the SOC decline after the conversion to a vegetable field was that the organic C input in the paddy–rape rotation was 1.35-fold higher than that of the vegetable cropping (Fig. 2a). Tillage operations were performed more frequently in the vegetable fields, i.e., four times per year instead of two in the paddy–rape rotation, and it clearly affected topsoil SOC (0–20 cm) but not in the deeper layers (Fig. 2b). Moreover, soil N stock in the vegetable fields generally decreased in line with SOC stock, with a slight variation in this trend observed in the 40–60 cm layer (Fig. 2c).
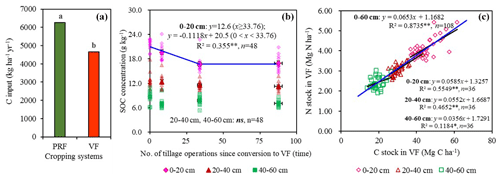
Figure 2Organic C inputs in paddy rice–oilseed rape rotation and vegetable production (a), the relationship between SOC content and the accumulative number of tillage operations since conversion to the vegetable cropping system (b), and the relationship between soil N stock and soil C stock in the 0–20, 20–40, and 40–60 cm soil layers in the vegetable production (c). Error bars represent the standard deviation. Significance levels are * P<0.05, P<0.01, and P<0.001. Note: ns – non-significant difference; VF – vegetable production; PRF – paddy rice–oilseed rape rotation.
3.3 Change in soil-available P
Soil AP and CaCl2-P content in the 0–60 cm soil layer were significantly higher in the vegetable field than in the paddy–rape soil, with levels increasing with time since the conversion to vegetable production (Fig. 3). For example, compared to the paddy–rape rotation, soil AP and CaCl2-P were 22- and 9-fold higher, respectively, in the vegetable production system, where vegetables were farmed continuously over 15 years. These increases in deeper soil layers are indicative of downward movement of P. In term of the surface layer (0–20 cm) in the vegetable field, the accumulation of P surplus (P inputs minus crop offtake) was significantly and positively correlated with differences in soil AP between vegetable cropping system and paddy–rape rotation at the end of the growing season (Fig. 4a). For every 100 kg ha−1 of P surplus in the vegetable field, the AP content in the 0–20 cm soil layer was increased by 1.92 mg kg−1. The phenomenon of a change point has previously been observed in the relationship between soil AP and CaCl2-P (Hesketh and Brookes, 2000) and is used as an indicator of the risk of P loss. In the vegetable field, AP and CaCl2-P values of 0–20 cm soil at the change point, as calculated using a two-segment linear model, were 104 and 0.80 mg P kg−1, respectively (Fig. 4b). Above the change point, soil CaCl2-P increased rapidly with increasing AP.
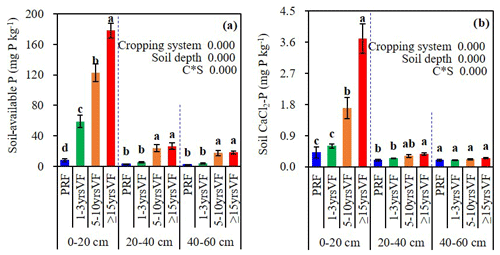
Figure 3Changes in soil-available phosphorus (P) (a) and CaCl2-P (b) in the 0–20, 20–40, and 40–60 cm soil layers in the paddy rice–oilseed rape rotation and vegetable production converted from the paddy–rape rotation in southwestern China 1–3, 5–10, or ≥15 years ago. For each soil layer, different lowercase letters indicate significant differences at P<0.05. Error bars represent standard deviation. ANOVA results are shown at the top right corner. Note: VF – vegetable production; PRF – paddy rice–oilseed rape rotation.
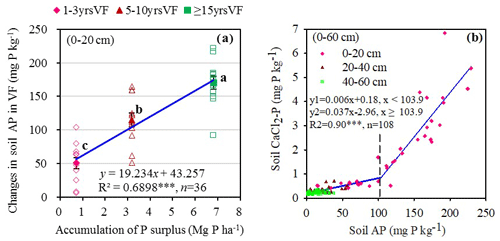
Figure 4The relationship between the change in soil-available phosphorus (AP) in vegetable production after conversion from paddy rice–oilseed rape rotation and the accumulation of P surplus in the 0–20 cm soil layer (a) and the change point (dashed line) in the relationship between soil CaCl2-P and AP in the 0–20 cm soil layer in the vegetable production (b). Different lowercase letters indicate significant differences at P<0.05 among cropping systems (conversion to vegetable fields 1–3, 5–10, or ≥15 years ago). Error bars represent standard deviation. Note: P<0.001; VF – vegetable production; PRF – paddy rice–oilseed rape rotation.
3.4 Changes in soil-available K, Ca, and Mg
The K nutrient removed via harvested crops in the paddy–rape rotation (33.8 kg K ha−1 yr−1) was only 11 % of that removed from the vegetable production (average – 306 kg K ha−1 yr−1; Table 1). Thus, the K surplus in the paddy–rape was, on average, 2.36 times that of the vegetable field. However, soil-available K levels (water-soluble and exchangeable fractions) were considerably higher in the vegetable field for all three soil layers (Fig. 5a and d). The depth distribution of soil-available K indicated that there was substantial downward movement of K. The accumulation of available K, but not exchangeable K, in each soil layer increased with the number of planting years since the conversion to vegetable production. Water-soluble K contents in the 0–20, 20–40, and 40–60 cm soil layers in the vegetable system which had continuous vegetable farming over 15 years were 26 %, 40 %, and 275 % higher, respectively, than those of new vegetable fields (cultivated for 1–3 years) but were also 193 %, 180 %, and 650 % higher than those in paddy–rape rotation, respectively.
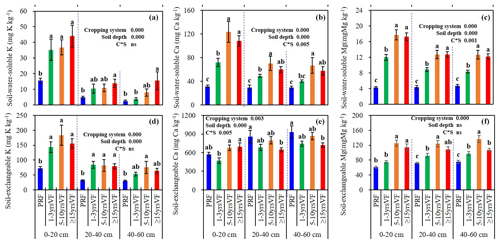
Figure 5Soil-water-soluble potassium (K) (a), water-soluble calcium (Ca) (b), water-soluble magnesium(Mg) (c), soil-exchangeable (including the water-soluble fraction) K (d), exchangeable Ca (e), and exchangeable Mg (f) in the 0–20, 20–40, and 40–60 cm soil layers in the paddy rice–oilseed rape rotation and vegetable production converted from the paddy–rape rotation in southwestern China 1–3, 5–10, or ≥15 years ago. For each soil layer, different lowercase letters indicate significant differences at P<0.05 among the cropping systems. Error bars represent standard deviation. The ANOVA result is shown in the blank space of the plot. Note: ns – no significant difference; VF – vegetable production; PRF – paddy rice–oilseed rape rotation.
Water-soluble Ca was significantly higher in the vegetable field (0–60 cm), and its level increased in the first 10 years after conversion from paddy–rape rotation but not thereafter (Fig. 5b). Similarly, the exchangeable Ca in the topsoil (0–20 cm) also increased with farming duration (Fig. 5e). The soil-available Mg (water-soluble and exchangeable fractions) content was also increased after conversion from paddy–rape rotation conversion to vegetable production and followed the same general trend as that of Ca (Fig. 5c and f).
3.5 Change in soil pH and its effect factors
Soil in the paddy–rape rotation system was slightly acidic, especially in the topsoil (0–20 cm), with an average pH of 5.34 across the fields sampled. It initially decreased to approximately pH 4.7 after conversion to the vegetable field but recovered eventually (Fig. 6). The initial pH was higher in the deeper soils (ca. pH 6.5) than in the 0–20 cm layer in the paddy–rape rotation, but it was significantly lower in the vegetable field. The soil-exchangeable Ca and soil pH were significantly and positively correlated in both the vegetable field and paddy–rape rotation system, particularly in the vegetable cropping system (Table 3), while soil-exchangeable Mg exhibited the same trend in the vegetable field. In addition, excessive N and K surplus were significantly correlated with decreased soil pH in the 0–20 cm soil layer. Conversely, the enrichment of soil P had a negative effect on soil pH.
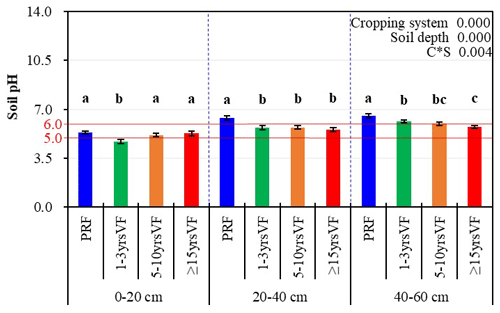
Figure 6Soil pH in 0–20, 20–40, and 40–60 cm soil layers in the paddy rice–oilseed rape rotation and vegetable production converted from the paddy–rape rotation in southwestern China 1–3, 5–10, or ≥ 15 years ago. For each soil layer, different lowercase letters indicate significant differences at P<0.05 among cropping systems. Error bars represent the standard deviation. The ANOVA result is shown in the top right corner. Note: VF – vegetable production; PRF – paddy rice–oilseed rape rotation.
Table 3Pearson correlation analysis of the relationships between soil properties and soil pH in the 0–20, 20–40, and 40–60 cm soil layers in vegetable fields and paddy rice–oilseed rape rotation fields.
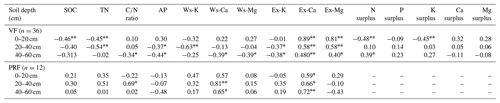
SOC – soil organic carbon; TN – total nitrogen; AP – soil-available phosphorus; Ws-K (Ca, Mg) – soil water-soluble potassium (calcium, magnesium); Ex-K (Ca, Mg) – soil exchangeable K (Ca, Mg; includes the water-soluble fraction); PRF – paddy rice–oilseed rape rotation; VF – vegetable production. Significance levels are * P<0.05 and P<0.01.
4.1 Nutrient balance
Through the farmer surveys, we obtained a considerable amount of data on the fertilizer application rates in the paddy–rape rotation and vegetable production systems. These two cropping systems are mainly used in southwestern China. The annual application rates of fertilizer N, P, and K in the vegetable production (Table 1) were much higher than those recommended (N – 600–765 kg ha−1; P – 79–144 kg ha−1; K – 398–498 kg ha−1) based on crop requirements and soil properties (i.e., the agriculture extension agencies; Zhang et al., 2009). In the paddy–rape rotation, fertilizer N and K inputs were also much higher than the recommended levels (N – 255–330 kg ha−1; K – 12–174 kg ha−1), but the annual fertilizer P rate was close to the local recommended rate (P – 33–79 kg ha−1; Zhang et al., 2009). Consequently, nutrients surplus developed under both cropping systems but were especially large in the vegetable production. Thus, on average, N, P, K, Ca, and Mg use efficiencies (nutrients removed in the harvested crop expressed as a percentage of nutrient input) were 26 %, 8 %, 56 %, 23 %, and 28 %, respectively. Vegetable crops at the national scale have an estimated N use efficiency of 26±13 % in China, which is consistent with our finding (Ti et al., 2015). The low P use efficiency of vegetable crops found in the present study is even lower than the values reported previously (15 %–20 %; Conley et al., 2009; Yan et al., 2013; Zhang et al., 2008).
Vegetable yields and fertilizer use efficiency in southwestern China could be due to relatively little support from local extension services (Ju et al., 2007), including a lack of fertilization recommendations and farmer education materials, remoteness of advisers from the production fields, and a lack of training for most farmers. It also could be ascribed to the small farm size, with the average farm size in this region being 0.07 to 0.1 ha (Zhang, 2017). Field size is often used as a proxy for the level of professionalism exhibited by farmers and awareness of efficient nutrient management practices (Ju et al., 2016; Wu et al., 2018), while smallholder farmers in China generally have a low awareness of fertilizer-saving practices, and farm size significantly influences the usage intensity and efficiency of agricultural chemicals.
Previous work in our group has shown that there is considerable scope for increasing yields and decreasing fertilizer use in vegetable production. For instance, Wang et al. (2018b) investigated the status quo of pepper production in the same subtropical region of China and found that both nutriment management (in particular, decreasing the application of N and P fertilizer) and crop management (mainly planting density) account for the yield difference among four groups of pepper considerably, and nutrient discharge remains the major contributor of environmental disturbances (e.g., global warming potential, eutrophication potential, and acidification potential). Furthermore, integrated soil–crop system management approaches (ISSM) based on advanced crop and nutrient management have been successfully developed for cereal cropping in China (Chen et al., 2014). The grain yields of maize, rice, and wheat can be increased by 35 % (n=5406), 18 % (n=6592), and 24 % (n=6940), respectively, with no increase in fertilizer inputs and, often, achieve a decrease in fertilizer use instead when using the ISSM approach (Chen et al., 2014). An integrated management system has been developed for greenhouse pepper production in the Yangtze River basin (Wang et al., 2020). After implementing ISSM for 2 years, pepper yield was increased by 16 %, and the N application rate was decreased by 54 %, which is a cumulative outcome of optimized soil management, plant population, and nutrient management (Wang et al., 2020). Thus, local extension services could provide support and resources for applying integrated soil–crop system management methods to increase vegetable production, minimize nutrients surplus, and mitigate soil degradation of vegetable fields in the study region.
4.2 SOC and N stocks
Our study provides unequivocal evidence for a sharp decline in SOC and TN content and stocks after converting the long-established paddy–rape rotation to open-field vegetable production. The trend was clearest in the topsoil, where initial levels of SOC and TN were the highest, but it was also apparent in the 20–40 and 40–60 cm soil layers (Table 2). SOC was depleted further during the vegetable farming, particularly in the first 10 years (Table 2), but the decrease observed thereafter was generally small. The low input of organic C from organic fertilizers and crop residues (Fig. 2a) and high tillage frequency (Fig. 2b) were two factors responsible for causing this change. Our results agreed with the findings of Wang et al. (2014), who reported that SOC content decreased by 25 % following the conversion from paddy soil to a vegetable field in southern China. We estimated that the average annual C input in the vegetable cropping was 25 % less than that of the paddy–rape rotation (Fig. 2a). A higher frequency of tillage operation generally results in the breakup of soil macro-aggregates, soil structure damage (Chivenge et al., 2007; Pires et al., 2017; Six et al., 2000; Gómez et al., 2020), and an increase in soil aeration, which promotes the microbial decomposition of SOC (Sarker et al., 2018). Accelerated SOC decomposition by high air temperatures and soil moisture levels in subtropical regions has been reported (Zhang et al., 2013).
The present study also indicated that soil TN content declined proportionally less than the SOC content after conversion to vegetable cropping. This was accompanied by marked changes in the soil ratio (Table 2). For instance, compared to the paddy–rape rotation, the soil ratio in the 20–40 cm layer depth decreased from 10.5 to 9.01 in the vegetable field, which had continuous farming over 15 years, and a larger change was observed in the 40–60 cm depth soil layer. Though the changes were significant, the absolute quantities of C and N in the deeper soil layers were small (Table 2); thus, experimental errors from sampling and analysis could easily be magnified when calculating ratios. However, the ratio did seem to exhibit a decreasing trend, which has been noted in other studies of land use conversion from cereal to vegetable cropping (Ju et al., 2007; Yan et al., 2012). As this is effected by a large N surplus (Table 1), it is perhaps conceivable that a fraction of the inorganic N in the soil profile was immobilized into soil organic matter under the conditions prevailing in the vegetable production, thus counteracting the loss of N that accompanied the observed SOC loss. The slightly lower pH in subsoil in the vegetable field compared to that in the paddy–rape rotation might have slowed nitrification such that part of the inorganic N remained in the ammonium form sufficiently long enough for some immobilization to occur (Fig. 6; Marschner, 2012). However, Nieder et al. (2011) indicated that the ammonium fixing capacity of the Ultisol, the type of soil used in this study, is extremely low. This may increase the risk of ammonium losses in this region, which is a potential environmental issue that warrants more attention in the future.
We did not directly measure N losses at the study sites, but the large N surplus strongly implies that the losses would have been substantial. Zhang et al. (2017) found that 36 % of the N applied (at a rate of 139 kg N ha−1) during the growing season of bitter gourd was lost by leaching in the same subtropical region. In other intensive vegetable cropping systems, Wang et al. (2018a, 2019) measured a loss of 3.91 kg N2O-N ha−1 per season and a runoff of 16.5 kg N ha−1 per year. Therefore, losses of this order are to be expected in our study region. Hence, to minimize N loss, which leads to undesirable environmental impacts, such as water pollution, eutrophication, and greenhouse gas emissions, rational N fertilizer management for intensive vegetable production in high-rainfall, subtropical environments is urgently needed.
4.3 P enrichment
P enrichment in farmland soils in China has been a serious problem for many years, as the movement of phosphate to surface waters causes environmental pollution (Bai et al., 2013; Cao et al., 2012; Li et al., 2011; Yan et al., 2013). On average, the annual P input (373 kg P ha−1; Table 1) in the present study was 2.6–4.7 times higher than those recommended for vegetable production agriculture (Zhang et al., 2009). Soil AP varied widely and ranged from 14.9 to 229 mg P kg−1 (mean, 120 mg P kg−1) in the 0–20 cm soil layer, with a large variation which is several times higher than the critical AP based on yield for fruit vegetables (58 mg P kg−1) and leafy vegetables (46 mg P kg−1; Yan et al., 2013). It has been noted that when the soil pH reaches values less than 5.3, as occurred with the conversion to vegetable production, the presence of aluminum and iron in clay minerals of Ultisols can precipitate P fixation. Therefore, available P content in the 0–20 cm soil has increased by 1.92 mg kg−1 for every 100 kg ha−1 of P surplus in the vegetable field. Compared to other cropping systems in different type of soils, this increasing coefficient ranged from 1.44 to 5.74 mg kg−1 (Cao et al., 2012). A high level of P enrichment in the soil is detrimental to plant growth because it inhibits the rhizosphere manipulation processes employed by plants to efficiently acquire P, including the colonization of roots by arbuscular mycorrhiza fungi and the exudation of organic acids or phosphatase enzymes (Dong et al., 2004; Ryan and Graham, 2002; Zhang et al., 2019). Besides, exudates in combination with organic residues better protect soils against structural destabilization by increasing particle cementation and decreasing rapid soil wetting and porosity (Oleghe et al., 2019).
CaCl2-P serves as an indicator of the risk of P loss from leaching (Hesketh and Brookes, 2000). Previous studies have reported a change point in the graph of soil-available P against CaCl2-P, above which the risk of P leaching increases greatly (Bai et al., 2013; Shen et al., 2018; Yan et al., 2013). Here, in terms of the 0–20 cm soil layer for a vegetable field, though the critical value of soil AP exceeds the observation value, in a few other vegetable experimental reports, due to the difference in soil type, excessive P fertilizer application should be reduced and the P surplus should be zero to avoid P movement to waterbodies.
4.4 Soil pH responded to land use change
At the national scale, long-term intensive farming has been shown to cause soil acidification, with an average decline of 0.50 units in pH value in the topsoil of major Chinese croplands from 1980 to 2000 (Guo et al., 2010). Soil acidification is caused by the excessive application of N fertilizers and the loss of base cations via leaching (Guo et al., 2010; Zhang et al., 2016), which leads to decreased crop production and quality (Cakmak and Yazici, 2010). After the conversion from paddy–rape rotation to vegetable cropping, soil pH at all depths decreased, especially 1–3 years after land conversion, by 0.64, 0.68, and 0.40 pH units in 0–20, 20–40, 40–60 cm, respectively (Fig. 6). With increasing farming duration, the pH in the 0–20 cm soil layer increased slightly and either stayed as it is or decreased very slightly in the deeper soil layers. Meanwhile, exchangeable Mg and, to a lesser extent, Ca, accumulated due to the types of fertilizers used in the vegetable production. This apparently sets the trend towards acidification, as shown by the correlation between Mg and Ca accumulation and soil pH (Table 3). In this sense, the current fertilization practice does confer benefits in terms of improving soil quality. However, the greater accumulation rate of Mg compared to Ca is likely to cause physiological damage to crops due to the antagonism between the two elements. An imbalance of soil mineral nutrients in vegetable field has been observed in other fields in southwestern China.
In this study, mean content of soil-exchangeable Mg in the new vegetable field which was farmed for 1–3 years is considered deficient (73.2 mg kg−1), as it is much lower than the critical threshold (Bai et al., 2004). Cationic antagonism is also an important influencing factor of mineral nutrient bioavailability (Tisdale et al., 1993; Marschner, 2012). With increasing farming duration (1–10 years), the soil-exchangeable K, Ca, and Mg content gradually increased (Fig. 5). The ratio of ex-K ex-Mg decreased from 1.92 to 1.37 and can be observed as the accumulation ratio of each nutrient was not consistent (K > Mg > Ca), while the ratio of ex-Ca ex-Mg remained at 6.14–6.29 with little change. However, it has been reported that plant Mg absorption would be inhibited by soil K and Ca when the value of soil ex-K ex-Mg and ex-Ca ex-Mg greater than 0.6 and 7.0, respectively (Tisdale et al., 1993; Morton et al., 2008). Thus, the current vegetable cropping practices can easily result in Mg deficiency (Yan et al., 2016). Nowadays, more attention has been paid to Mg in the soil–plant–human continuum and, with intensive vegetable rotation in southwestern China, an optimal Mg fertilization strategy is urgently needed.
Under conventional agricultural practices, intensive open-field vegetable production in southwestern China can result in significant soil degradation and potential environmental pollution after conversion from paddy rice to an oilseed rape rotation. Application of fertilizers in the current vegetable production is often far higher than the crop requirement or even the recommendation by the local extension service. Excessive fertilization has led to substantial soil enrichment of P, K, Ca, and Mg. In this study, a trend of soil acidification at 0–60 cm soil layer depths was observed, although it was partially alleviated by the accumulation of Mg and Ca. Additionally, SOC decreased rapidly after conversion to vegetable cropping, and its levels continued to decrease over time. This was attributed to decreased C inputs from organic fertilizer and crop residues and increased operation frequency of deep tillage compared to the paddy–rape rotation, as well as the high temperatures and moisture levels prevalent under subtropical climatic conditions. The reduction in SOC inevitably causes a decline in soil physical and biological properties, with potential long-term impacts on the sustainability of the vegetable production agriculture. The ratio was found to decrease in deeper soil layers in the vegetable field. Management practices likely to slow or reverse soil degradation and prevent environmental pollution in the vegetable system include increasing organic inputs (as either manure, organic fertilizers, or crop residues, possibly through a change in the crop rotation to include some cereals with the possibility of incorporating straw), optimizing fertilizer application to better match crop requirements, decreasing tillage frequency, and changing the types of chemical fertilizers used to avoid an imbalance between Mg and Ca. These may be achieved by applying ISSM concepts through the development of effective agriculture extension strategies to deliver information to farmers.
The data that support the findings of this study are available on request from the corresponding author (Dunyi Liu).
DL and XC designed the experimental setup. ML and YL did soil sampling and led the lab analysis procedure and the farmer survey, with the input of SL. ML, DSP, and DL also did the statistics, prepared the paper, with valuable contributions of DRC and XC, and undertook the revisions during the reviewing process.
The authors declare that they have no conflict of interest.
Publisher's note: Copernicus Publications remains neutral with regard to jurisdictional claims in published maps and institutional affiliations.
This research has been supported by funding from the National Natural Science Foundation of China (grant no. 31902117), the National Key Research and Development Program of China (grant no. 2018YFD0800600), the China Postdoctoral Science Foundation (grant no. 2018M643393), the State Cultivation Base of Eco-Agriculture for Southwest Mountainous Land (Southwest University), and UK Research and Innovation (UKRI) through the UK Biotechnology and Biological Sciences Research Council (BBSRC).
This paper was edited by Fuensanta García-Orenes and reviewed by Lukas Kohl, Paul Hallett, and one anonymous referee.
Bai, Y., Jin, J., and Yang, L.F: Study on the content and distribution of soil available magnesium and foreground of magnesium fertilizer in China, Soil Fert. Sci. China, 2, 3–5, 2004 (in Chinese).
Bai, Z., Li, H., Yang, X., Zhou, B., Shi, X., Wang, B., Li, D., Shen, J., Chen, Q., Qin, W., Oenema, O., and Zhang, F.: The critical soil P levels for crop yield, soil fertility and environmental safety in different soil types, Plant Soil, 372, 27–37, https://doi.org/10.1007/s11104-013-1696-y, 2013.
Bünemann, E. K., Bongiorno, G., Bai, Z., Creamer, R. E., Deyn, G. D., Goede, R., Fleskens, L., Geissen V., Kuyper, T. W., Mäder, P., Pulleman, M., Sukkel, W., Groenigen J. W., and Brussaard, L.: Soil quality – A critical review, Soil Biol. Biochem., 120, 105–125, https://doi.org/10.1016/j.soilbio.2018.01.030, 2018.
Cakmak, I. and Yazici, A. M.: Magnesium: a forgotten element in crop production, Better Crops Plant Food, 94, 23–25, 2010.
Cao, N., Chen, X., Cui, Z., and Zhang, F.: Change in soil available phosphorus in relation to the phosphorus budget in China, Nutr. Cycl. Agroecosys., 94, 161–170, https://doi.org/10.1007/s10705-012-9530-0, 2012.
Chen, X., Cui, Z., Fan, M., Vitousek, P., Zhao, M., Ma, W., Wang, Z., Zhang, W., Yan, X., Yang, J., Deng, X., Gao, Q., Zhang, Q., Guo, S., Ren, J., Li, S., Ye, Y., Wang, Z., Huang, J., Tang, Q., Sun, Y., Peng, X., Zhang, J., He, M., Zhu, Y., Xue, J., Wang, G., Wu, L., An, N., Wu, L., Ma, L., Zhang, W., and Zhang, F.: Producing more grain with lower environmental costs, Nature, 514, 486–492, https://doi.org/10.1038/nature13609, 2014.
Chen, Y., Yi, X., Fang, L., and Yang, R.: Analysis of cultivated land and grain production potential in China, Sci. Agric. Sinica., 49, 1117–1131, https://doi.org/10.3864/j.issn.0578-1752.2016.06.008, 2016 (in Chinese).
Chivenge, P. P., Murwira, H. K., Giller, K. E., Mapfumo, P., and Six, J.: Long-term impact of reduced tillage and residue management on soil carbon stabilization: Implications for conservation agriculture on contrasting soils, Soil Till. Res., 94, 328–337, https://doi.org/10.1016/j.still.2006.08.006, 2007.
Conley, D. J., Paerl, H. W., Howarth, R. W., Boesch, D. F., Seitzinger, S. P., Havens, K. E., Lancelot C., and Likens, G. E.: Controlling eutrophication: nitrogen and phosphorus, Science, 323, 1014–1015, https://doi.org/10.1126/science.1167755, 2009.
Dong, D., Peng, X., and Yan, X.: Organic acid exudation induced by phosphorus deficiency and/or aluminium toxicity in two contrasting soybean genotypes, Physiol. Plant., 122, 190–199, https://doi.org/10.1111/j.1399-3054.2004.00373.x, 2004.
FAO: FAOSTAT Database-Resources. Food and Agriculture Organization of the United Nations, FAO Statistical Yearbook 2017: World Food and Agriculture, Rome, 2017.
Fischer, G., Nachtergaele, F. O., Prieler, S., Teixeira, E., Toth, G., Velthuizen, H. V., Verelst, L., and Wiberg, D.: Global Agro-Ecological Zones (GAEZ v 3.0) – Model Documentation, Laxenburg, IIASA and Rome, FAO, 2012.
Gómez, J. A., Guzmán, G., Toloza, A., Resch, C., García-Ruíz, R., and Mabit, L.: Variation of soil organic carbon, stable isotopes, and soil quality indicators across an erosion–deposition catena in a historical Spanish olive orchard, SOIL, 6, 179–194, https://doi.org/10.5194/soil-6-179-2020, 2020.
Guo, J., Liu, X., Zhang, Y., Shen, J., Han, W., Zhang, W., Christie, P., Goulding, K. W. T., Vitousek, P. M., and Zhang, F.: Significant acidification in major Chinese croplands, Science, 327, 1008–1010, https://doi.org/10.1126/science.1182570, 2010.
Hesketh, N. and Brookes, P. C.: Development of an indicator for risk of phosphorus leaching, J. Environ. Qual., 29, 105–110, https://doi.org/10.2134/jeq2000.00472425002900010013x, 2000.
Jia, X., Huang, J., Xiang, C., Hou, L., Zhang, F., Chen, X., Cui, Z., and Bergmann, H.: Farmer's adoption of improved nitrogen management strategies in maize production in China: an experimental knowledge training, J. Integr. Agr., 12, 364–373, https://doi.org/10.1016/S2095-3119(13)60237-3, 2013.
Ju, X., Kou, C., Christie, P., Dou, Z., and Zhang, F.: Changes in the soil environment from excessive application of fertilizers and manures to two contrasting intensive cropping systems on the North China Plain, Environ. Pollut., 145, 497–506, https://doi.org/10.1016/j.envpol.2006.04.017, 2007.
Ju, X., Gu, B., Wu, Y., and Galloway, J.: Reducing China's fertilizer use by increasing farm size, Global Environ. Chang., 41, 26–32, https://doi.org/10.1016/j.gloenvcha.2016.08.005, 2016.
Lal, R.: Soil carbon sequestration impacts on global climate change and food security, Science, 304, 1623–1627, https://doi.org/10.1126/science.1097396, 2004.
Lal, R.: Restoring soil quality to mitigate soil degradation, Sustain., 7, 5875–5895, https://doi.org/10.3390/su7055875, 2015.
Li, H., Huang, G., Meng, Q., Ma, L., Yuan, L., Wang, F., Cui, Z., Shen, J., Chen, X., Jiang, R., and Zhang, F.: Integrated soil and plant phosphorus management for crop and environment in China: A review, Plant Soil, 349, 157–167, https://doi.org/10.1007/s11104-011-0909-5, 2011.
Lu, R. (Ed.): Analysis Method of Agricultural Chemistry in Soil, 1st Edn., Agriculture and Science Press, Beijing, China, ISBN: 7-80119-925-1, 2000.
Marschner, P. (Ed.): Marschner's Mineral Nutrition of Higher Plants, 3rd Edn., Academic Press, USA, ISBN: 978-0-12-384905-2, 2012.
Morton, A. R., Trolove, S. N., and Kerckhoffs, L. H. J.: Magnesium deficiency in citrus grown in the Gisborne district of New Zealand, New Zealand J. Crop Hortic. Sci., 36, 199–213, https://doi.org/10.1080/01140670809510236, 2008.
National Bureau of Statistics: National Agricultural Product Cost-benefit Compilation (NAPCC), China, available at: http://data.stats.gov.cn/index.htm, last access: 8 October 2017.
Nieder, R., Benbi, D. K., and Scherer, H. W.: Fixation and defixation of ammonium in soils: a review, Biol. Fert. Soils, 47, 1–14, https://doi.org/10.1007/s00374-010-0506-4, 2011.
Oleghe, E., Naveed, M., Baggs, E. M., and Hallett, P. D.: Residues with varying decomposability interact differently with seed or root exudate compounds to affect the biophysical behaviour of soil, Geoderma, 343, 50–59, https://doi.org/10.1016/j.geoderma.2019.02.023, 2019.
Pires, L. F., Borges, J. A. R., Rosa, J. A., Cooper, M., Heck, R. J., Passoni, S., and Roque, W. L.: Soil structure changes induced by tillage systems, Soil Till. Res., 165, 66–79, https://doi.org/10.1016/j.still.2016.07.010, 2017.
Powlson, D. S., Stirling, C. M., Thierfelder, C., White, R. P., and Jat, M. L.: Does conservation agriculture deliver climate change mitigation through soil carbon sequestration in tropical agro-ecosystems?, Agr. Ecosyst. Environ., 220, 164–174, https://doi.org/10.1016/j.agee.2016.01.005, 2016.
Ryan, M. H. and Graham, J. H.: Is there a role for arbuscular mycorrhizal fungi in production agriculture?, Plant Soil, 244, 263–271, https://doi.org/10.1023/a:1020207631893, 2002.
Sarker, J. R., Singh, B. P., Dougherty, W. J., Fang, Y., Badgery, W., Hoyle, F. C., Dalal, R. C., and Cowie, A. L.: Impact of agricultural management practices on the nutrient supply potential of soil organic matter under long-term farming systems, Soil Till. Res., 175, 71–81, https://doi.org/10.1016/j.still.2017.08.005, 2018.
Shen, Y., Duan, Y., Huang, S., Guo, D., Zhang, S., and Xu, M.: Response of CaCl2-P to phosphorus fertilization and leaching risk in fluvo-aquic soils, J. Plant Nutr. Fert., 24, 1689–1696, https://doi.org/10.11674/zwyf.18167, 2018 (in Chinese).
Six, J., Elliott, E. T., and Paustian, K.: Soil macroaggregate turnover and microaggregate formation: a mechanism for C sequestration under no-tillage agriculture, Soil Biol. Biochem., 32, 2099–2103, https://doi.org/10.1016/s0038-0717(00)00179-6, 2000.
Ti, C., Luo, Y., and Yan, X.: Characteristics of nitrogen balance in open-air and greenhouse vegetable cropping systems of China, Environ. Sci. Pollut. Res., 22, 18508–18518, https://doi.org/10.1007/s11356-015-5277-x, 2015.
Tisdale, S. L., Nelson, W. L., and Beaton, J. D.: Soil Fertility and Fertilizers, 5th Edn., Macmillan Publishing Co., New York, 1993.
Wang, H., Guan, D., Zhang, R., Chen, Y., Hu, Y., and Xiao, L.: Soil aggregates and organic carbon affected by the land use change from rice paddy to vegetable field, Ecol. Eng., 70, 206–211, https://doi.org/10.1016/j.ecoleng.2014.05.027, 2014.
Wang, R., Min, J., Kronzucker, H. J., Li, Y., and Shi, W.: N and P runoff losses in China's vegetable production systems: Loss characteristics, impact, and management practices, Sci. Total Environ., 663, 971–979, https://doi.org/10.1016/j.scitotenv.2019.01.368, 2019.
Wang, X., Zou, C., Gao, X., Guan, X., Zhang, W., Zhang, Y., and Chen, X.: Nitrous oxide emissions in Chinese vegetable systems: a meta-analysis, Environ. Pollut., 239, 375–383, https://doi.org/10.1016/j.envpol.2018.03.090, 2018a.
Wang, X., Zou, C., Zhang, Y., Shi, X., Liu, J., Fan, S., Liu, Y., Du, Y., Zhao, Q., Tan, Y., Wu, C., and Chen, X.: Environmental impacts of pepper (Capsicum annuum L.) production affected by nutrient management: A case study in southwest China, J. Clean. Prod., 171, 934–943, https://doi.org/10.1016/j.jclepro.2017.09.258, 2018b.
Wang, X., Liu, B., Wu, G., Sun, Y., Guo, X., Jin, G., Jin, Z., Zou, C., Chadwick, D., and Chen, X.: Cutting carbon footprint of vegetable production with integrated soil – crop system management: A case study of greenhouse pepper production, J. Clean. Prod., 254, 120158, https://doi.org/10.1016/j.jclepro.2020.120158, 2020.
White, P. J. and Broadley, M. R.: Biofortification of crops with seven mineral elements often lacking in human diets – iron, zinc, copper, calcium, magnesium, selenium and iodine, New Phytol., 182, 49–84, https://doi.org/10.1111/j.1469-8137.2008.02738.x, 2009.
Wu, Y., Xi, X., Tang, X., Luo, D., Gu, B., Lam, S. K., Vitousek P. M., and Chen, D.: Policy distortions, farm size, and the overuse of agricultural chemicals in China, P. Natl. Acad. Sci. USA, 115, 7010–7015, https://doi.org/10.1073/pnas.1806645115, 2018.
Yan, B., Zhou, T., Wang, H., Chen, Z., Cao, J., Liu, S., and Zhou, J.: The relationships between magnesium deficiency of tomato and cation balances in solar greenhouse soil, Sci. Agr. Sin., 49, 3588–3596, https://doi.org/10.3864/j.issn.0578-1752.2016.18.013, 2016 (in Chinese).
Yan, Y., Tian, J., Fan, M., Zhang, F., Li, X., Christie, P., Chen, H., Lee, J., Kuzyakov, Y., and Six, J.: Soil organic carbon and total nitrogen in intensively managed arable soils, Agr. Ecosyst. Environ., 150, 102–110, https://doi.org/10.1016/j.agee.2012.01.024, 2012.
Yan, Z., Liu, P., Li, Y., Ma, L., Alva, A., Dou, Z., Chen, Q., and Zhang, F.: Phosphorus in China's intensive vegetable production systems: overfertilization, soil enrichment, and environmental implications, J. Environ. Qual., 42, 982–989, https://doi.org/10.2134/jeq2012.0463, 2013.
Zhang, B.: Theory, model and route on spatial recombination of arable land use system based on land fragmentation perspective, PhD dissertation, China Agricultural University, 2017 (in Chinese).
Zhang, B., Li, Q., Cao, J., Zhang, C., Song, Z., Zhang, F., and Chen, X.: Reducing nitrogen leaching in a subtropical vegetable system, Agr. Ecosyst. Environ., 241, 133–141, https://doi.org/10.1016/j.agee.2017.03.006, 2017.
Zhang, F., Chen, X., and Chen, Q.: Fertilization recommendations for major crops in China, China Agricultural University Press, Beijing, 2009 (in Chinese).
Zhang, W., Ma, W., Ji, Y., Fan, M., Oenema, O., and Zhang, F.: Efficiency, economics, and environmental implications of phosphorus resource use and the fertilizer industry in China, Nutr. Cycl. Agroecosys., 80, 131–144, https://doi.org/10.1007/s10705-007-9126-2, 2008.
Zhang, W., Zhang, F., Li, Y., Huang, G., and Wu, L.: China fertilizer development research report, China Agricultural University Press, Beijing, 2012 (in Chinese).
Zhang, X., Zhang, S., Li, Q., Lu, Y., and Liang, W.: Contributions of soil biota to C sequestration varied with aggregate fractions under different tillage systems, Soil Biol. Biochem., 62, 147–156, https://doi.org/10.1016/j.soilbio.2013.03.023, 2013.
Zhang, Y., He, X., Liang, H., Zhao, J., Zhang, Y., Xu, C., and Shi, X.: Long-term tobacco plantation induces soil acidification and soil base cation loss, Environ. Sci. Pollut. Res., 23, 5442–5450, https://doi.org/10.1007/s11356-015-5673-2, 2016.
Zhang, Y., Fan, Z., Chen, X., Yang, H., Wu, Y., and Zhang, W.: Effect of phosphorus supply on root morphology and rhizosphere traits in pepper, China Veg., 11, 49–55, 2019 (in Chinese).