the Creative Commons Attribution 4.0 License.
the Creative Commons Attribution 4.0 License.
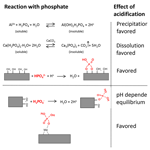
Isotopic exchangeability reveals that soil phosphate is mobilised by carboxylate anions, whereas acidification had the reverse effect
Siobhan Staunton
Chiara Pistocchi
Mineral P is an increasingly scarce resource, and therefore the mobilisation of legacy soil P must be optimised to maintain soil fertility. We have used isotopic exchangeability to probe the lability of native soil P in four contrasting soils following acidification and the addition of carboxylate anions (citrate and oxalate) in soil suspension. Acidification tended to cause immobilisation of soil P, but this was attributed to a salt effect. Addition of both citrate and oxalate led to marked increases in mobilisation of soil P. This would result from both competition between carboxylate and phosphate ions at adsorption sites and chelation of charge-compensating cations. The carboxylate effects were similar at each level of acidification, indicating that effects were largely additive. This is not true for the most calcareous soil where calcium oxalate may have been precipitated at the highest oxalate addition. Promoting carboxylate anions in soil by soil amendment or the use of crops that exude large amounts of such organic anions is a promising approach to improve soil-P availability.
- Article
(563 KB) - Full-text XML
-
Supplement
(341 KB) - BibTeX
- EndNote
Phosphorus (P) is an essential nutrient. The bioavailability of soil P is severely restricted because only orthophosphate in solution, accounting for less than 1 % of soil P, is directly available for uptake. Inorganic P is largely adsorbed on soil clay minerals and metal (oxy)hydroxides or precipitated. Highly specific adsorption takes place on anion exchange sites of mineral surfaces, in particular clay edge sites, and metal oxides, both as discrete particles and surface coatings, and is characterised by marked hysteresis (Arai and Sparks, 2007; Crisler et al., 2020; García et al., 2021; Gérard, 2016; Geng et al., 2022). Mineral P is a limited resource. The use of P fertiliser must be rationed and more sustainable approaches to P nutrition found. The research focus has thus shifted from fertilisation to the optimisation of legacy soil-P availability, i.e. P stored in soils with a long fertilisation history. The challenge for the 21st century is to find strategies to mobilise soil legacy P with plant breeding and the judicious choice of soil amendments. Agronomic strategies include reasoned choice of crop varieties, promotion of microbial activity, addition of immobilised enzymes, pH adjustment, compost amendment, and soil enrichment with carboxylate anions, including citrus residues (Alam et al., 2023; Jalali and Jalali, 2022; Paredes et al., 2022; Sindhu et al., 2022). Inspiration for effective soil amendments depends on an understanding of the forms and exchangeability of soil P combined with observation of the strategies of plants and soil biota to cope with P deficiency (Soumya et al., 2022)
Among the most widely considered modifications thought to promote soil-P availability are the modification of pH and the enrichment in carboxylate anions. The mechanisms of solubilisation of P by acidification and organic anions are quite well understood and have been reviewed (Geng et al., 2022; Barrow, 2017; Duputel et al., 2013; Khademi et al., 2009). The forms of orthophosphate in soil and the charge on P-adsorption sites are pH-sensitive, leading to pH sensitivity of adsorption–desorption reactions. It is now accepted that acidification and carboxylate release by plants and soil biota are separate processes. Carboxylates may exchange with P on soil mineral surfaces and chelate metal cations, thus solubilising P-carrier phases. The extent of the role of carboxylates on P nutrition in situ remains poorly quantified and lies in the difficulty in quantifying their release by plants. One of the reasons is that the carboxylates are strongly adsorbed by soil, so quantification depends on their desorption. They are also short-lived due to biological breakdown. To avoid this, studies are often carried out in solution or in an inert matrix such a quartz, but it is recognised that plant physiology may be very different in soil (Wang and Lambers, 2019).
The relation between P solubility and pH is complex; acidification does not always promote solubility. For example, the mechanism of action of phosphate solubilising bacteria may not be acid release, as it is often presumed (Biswas et al., 2022; Barrow and Lambers, 2022; Menezes-Blackburn et al., 2016). The maximum of P availability is often assumed to occur at neutral pH (Penn and Camberato, 2019). This is attributed to the combination of pH dependences of P fixation by iron, aluminium, and calcium. However, both the explanation and the assumption are contested by Barrow (2017), who reported the minimum in the U-shaped relation between P desorption and pH at about pH 5. In calcareous soils, carbonates may be dissolved in preference to sparingly soluble phosphates upon acidification (Geng et al., 2022). The relative effects of pH and carboxylate anion are therefore not easily predicted, not least because some studies investigate carboxylate by adding the acid form.
Another important question is how to assess P availability reliably. Although the objective of all studies is to understand and predict biological P availability, uptake is species-dependent, so chemical extractions are often preferred. Common approaches assess the size of operationally defined “pools” of soil P. Water-extractable P is usually too low to be reliably measured, whereas harsh extractants (e.g. 1 M HCl) are unlikely to detect small changes in P availability. Furthermore, the size of soil-P pools is not related to their capacity to release P, and the rate of this release is soil-dependent (Helfenstein et al., 2020). Other common approaches to understanding and predicting soil-P dynamics include the study of adsorption isotherms. However, adsorption measures the affinity of soil to adsorb additional, often environmentally unrealistic, amounts of P. Adsorption isotherms give no information on the lability of either native or recently added P. Methods that probe the dynamics of immobilised soil P should be better suited to measure P availability. This is because biological uptake creates a depletion gradient, so availability is determined by the ease of replenishment of solution, namely the lability of immobilised P. One potentially suitable approach is resin-extractable P, which is reputed to be a good proxy for P that could be taken up by growing plants. Isotopic exchangeability provides another approach, with the advantage that it probes soil-P availability without displacing the sorption–desorption equilibria (McBeath et al., 2009, and references therein). Combinations of chemical extraction, spectroscopic techniques, and isotopic exchange suggest that they provide coherent information on P status in soil (Braun et al., 2020; Helfenstein et al., 2018; Randriamanantsoa et al., 2013).
The aim of this study was to elucidate the separate effects of pH and carboxylate anions on the solubility of phosphate. Four contrasting soils, with different pH, P availability, and carbonate content, were studied. We focussed on the effect of two carboxylates reported to be released by plants and associated microorganisms (Bhattacharyya et al., 2011), citrate, and oxalate. These anions have been found to have the largest effect on P solubility (Staunton and Leprince, 1996). We chose to use short-term (2 h) isotopic exchange of native soil P to assess its lability.
Some properties of the four contrasting soils selected for this study are given in Table 1. Soils were sampled in the Mediterranean region: one was acid, another neutral, and two calcareous. They also differed in their P status and pH-buffer capacity.
Soils were air-dried, sieved to 2 mm, and stored before use. All measurements were carried out in suspension (2 g soil / 4 mL). Preliminary experiments were carried out to measure soil pH buffer capacity by addition of HCl. Most soils showed a dramatic drop in pH when acidification was greater than 1 unit, so we decided to limit pH adjustment to this value. Preliminary tests showed that both carboxylate anions strongly decreased the adsorption of added phosphate. Water-extractable P (using the molybdate blue method) was found to be below the detection limit for three of the soils and about 3.6 µM for acid soil, Montana. pH was measured on the aqueous phase of suspension after settling of the suspension. The measurement of isotopically exchangeable P was carried out by adding carrier-free 32P to each suspension to obtain a final content of 50 MBq mL−1. Soils were suspended in solutions containing strong acid (HCl) to acidify the pH by about 0.5 or 1 pH unit and/or the sodium salt of oxalate or citrate (10−4, 10−3 or 10−2 N) or NaCl (10−2 N) as required. NaCl was added to test for a possible salt effect independent of the chelating action of carboxylate, i.e. change in ionic strength and therefore surface charge density and formation of cationic bridges. Since both citrate and oxalate are strongly adsorbed, no attempt was made to quantify the proportion of anion adsorbed or to follow the kinetics of microbial breakdown of the anion. After a contact period of 2 h between soil and solution, phases were separated by double centrifugation (10 min at 19 000 g, followed by 30 min at 19 000 g of 2 mL aliquots of the first supernatant solution). The proportion of 32P adsorbed was calculated from the difference of 32P content in the initial solution and after 2 h contact. All experiments were carried out in triplicate. After phase separation, 32P activity was quantified by liquid scintillation, after addition of 4.8 mL scintillation liquid (AquaSafe) to 0.5 mL aliquots of each sample (in triplicate). The distribution coefficient, defined as the ratio of adsorbed (x32P) to solution phase concentration of 32P, [32P], was calculated as follows:
where VL is the volume of solution in suspension, MS is the mass of soil, and the subscript 0 refers to time 0. This parameter is mathematically equivalent to the tangent of an adsorption/desorption isotherm and is inversely proportional to the lability of legacy P and to the effective diffusion coefficient of phosphate (Lambers and Plaxton, 2015).
Soils differed in their pH buffer capacities as shown in Table 1. The presence of oxalate or citrate led to small alkalinisation (about 0.1 unit, not shown). Preliminary experiments with adsorption isotherms showed that addition of 10 mM citrate or oxalate caused marked decreases in P adsorption, as reported in a similar study (Staunton and Leprince, 1996), but we decided not to pursue the investigation of P-adsorption isotherms because of the limited relevance of large amounts of added P to the dynamics of legacy P.
Figure 1 shows the effect of anion addition on the distribution of 32P on each of the soils. Soils are presented in order of increasing initial pH, pH0, from top to bottom, and the values of pH0 and pH buffer capacity, β, are also indicated. The addition of 10−2 N sodium chloride (20 mmol kg−1 soil) is also shown (closed symbols) to distinguish between the true effect of carboxylate and the simple salt effect from the addition of chloride from HCl. Data are also given in the Supplement. Addition of salt led to decreased mobilisation of legacy P, except in the near-neutral soil, Cazevieille. In contrast, addition of carboxylate anions led to marked decreases in the distribution coefficient (P]), namely mobilisation of legacy P. The specific effect of carboxylate should be assessed by comparison with the soil amended with simple salt. Comparison with unamended soil would underestimate the effect. The threshold for observation of a significant effect is about 1 mN for oxalate and somewhat greater for citrate. It should be remembered that solutions had the same normality, so concentrations of oxalate were larger than those of citrate. Two mechanisms would account for this effect. Firstly, ligand exchange between carboxylate anions and adsorbed phosphate should liberate P. Secondly, the chelating effect of the oxyanions solubilises Fe and Al oxides, which are important carrier phases for P. Similar additions of carboxylate anions have been reported to increase P lability (Staunton and Leprince, 1996; Menezes-Blackburn et al., 2016; Barrow et al., 2018), although, unlike the present study, in some studies weak acid was added. Therefore, effects of anion and acidification cannot be separated.
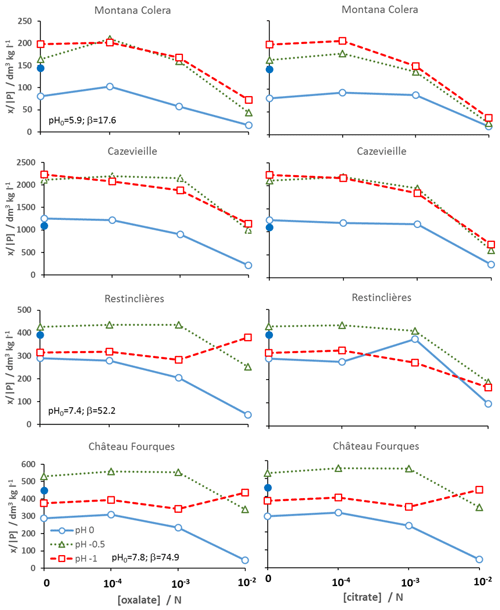
Figure 1Distribution of 32P on each of the soils, x32P/[32P], as a function of added citrate or oxalate sodium salt with or without acidification. Also shown is the distribution when chloride salt is added.
Both acidification and salt addition led to marked decreases in P lability. Acidification by addition of HCl implies the addition of chloride, and therefore any observed effect could be due to both salt concentration and acidification. Salt effects are associated with variable charge surfaces and the formation of cation bridges. The observation of a salt effect suggests, therefore, the implication of sorption on variable charge surfaces, e.g. kaolinite and metal oxides, in P immobilisation (Barrow, 2015). Only in the case of the neutral Cazevieille soil could the decrease in P lability not be explained by a salt effect. A true pH effect is more complex. The formation of phosphate bridging complexes at clay edge sites and the hydroxyl groups of Al–OH and Fe–OH consume protons, so equilibrium is displaced by pH changes. In the present study, the pH effect was not continuous, with an initial increase of x32P/[32P], followed by a decrease to close to the initial value, for the two calcareous soils: Château de Fourques and Restinclières. This could be interpreted as a U-shaped pH dependence of P lability, resulting from two different processes with opposite pH effects. Acidification of calcareous soils dissolves carbonates releasing calcium, explaining the large buffer index for these soils and to a lesser extent phosphate from precipitated calcium phosphate. Acidification by 0.5 pH units might not be sufficient to solubilise Ca phosphates, so the apparent acid effect was instead dominated by a salt effect. Further acidification could cause the release of P from Ca phosphates or co-precipitated with carbonates and displace the pH-dependent equilibria of adsorbed P. The acid soil, Montana Colera, was the only soil where the increasing acidification led to a further (small) increase in x32P/[32P], confirming a trend in addition to a salt effect. For this acid soil, acidification could not have solubilised carbonates.
It must be recalled that the pH changes had little effect on the acid–base equilibria of the carboxylate anions. Oxalate (pKa = 1.23 and 4.28) would be in divalent form at all pH levels. The equilibrium between the divalent and trivalent forms of citrate (pKa = 3.13, 4.76, 6.39) would change over the pH range, with roughly equal amounts of both around pH 6.4, more trivalent at more alkaline pH, and more divalent at more acid pH. Modelling of the citrate species present as a function of pH (Barrow, 2018) shows that important changes in Ca citrate complexes only occur in a pH range relevant to the acid soil in this study (4.5–5.5). The curves of x32P/[32P] with increasing carboxylate concentration followed the same trends at each acid addition. This implies that the anion effects, via ligand exchange and chelation, dominate over pH. The magnitude of the carboxylate effect was slightly greater for acidified soils, as seen by the convergence of curves. The only exception is observed for the two calcareous soils where there was an inversion of the trend with the highest oxalate concentration in more acid suspension. This may be attributed to precipitation of calcium oxalate (solubility product, KSP= 10−9 M), thus removing oxalate from solution and reducing its interaction with soil P.
In conclusion, carboxylate anion addition led to marked increases in P lability assessed by isotopic exchangeability, beyond a threshold of about 0.2 mol charge per kg soil, with greater increases observed for oxalate than citrate. Similar trends of carboxylate were observed when soils were simultaneously acidified. However, acidification tended to decrease P lability in all soils. In most cases the effect could be attributed to a salt effect (increase in ionic strength). When calcareous soils were acidified by about 1 pH unit, the effect of oxalate was small, probably due to precipitation of calcium oxalate. We found no evidence that P lability is greatest around neutral pH, although this is often assumed to be the case.
Data are provided in the Supplement, and any queries will be answered.
The supplement related to this article is available online at https://doi.org/10.5194/soil-11-389-2025-supplement.
SS contributed to conceptualisation, data curation, formal analysis, investigation, methodology, validation, and writing the original draft and revisions. CP contributed to formal analysis, methodology, validation, and writing the original draft and revisions.
The contact author has declared that neither of the authors has any competing interests.
Publisher’s note: Copernicus Publications remains neutral with regard to jurisdictional claims made in the text, published maps, institutional affiliations, or any other geographical representation in this paper. While Copernicus Publications makes every effort to include appropriate place names, the final responsibility lies with the authors.
This paper was edited by Luisella Celi Celi and reviewed by two anonymous referees.
Alam, K., Barman, M., Datta, S. P., Annapurna, K., and Shukla, L.: Modification of Inorganic Fractions of Phosphorus by Phosphate-Solubilising Microorganisms in Conjunction with Phosphorus Fertilisation in a Tropical Inceptisol, J. Soil Sci. Plant Nut., 23, 2488–2497, https://doi.org/10.1007/s42729-023-01206-6, 2023.
Arai, Y. and Sparks, D. L.: Phosphate Reaction Dynamics in Soils and Soil Components: A Multiscale Approach, Adv. Agron., 94, 135–179, https://doi.org/10.1016/s0065-2113(06)94003-6, 2007.
Barrow, N. J.: Soil phosphate chemistry and the P-sparing effect of previous phosphate applications, Plant Soil, 397, 401–409, https://doi.org/10.1007/s11104-015-2514-5, 2015.
Barrow, N. J.: The effects of pH on phosphate uptake from the soil, Plant Soil, 410, 401–410, https://doi.org/10.1007/s11104-016-3008-9, 2017.
Barrow, N. J., Debnath, A., and Sen, A.: Mechanisms by which citric acid increases phosphate availability. Plant and Soil, 423, 193–204, 2018.
Barrow, N. J. and Lambers, H.: Phosphate-solubilising microorganisms mainly increase plant phosphate uptake by effects of pH on root physiology, Plant Soil, 476, 397–402, https://doi.org/10.1007/s11104-021-05240-0, 2022.
Barrow, N. J., Debnath, A., and Sen, A.: Mechanisms by which citric acid increases phosphate availability, Plant Soil, 423, 193–204, https://doi.org/10.1007/s11104-017-3490-8, 2018.
Bhattacharyya, P., Datta, S. C., and Dureja, P.: Interrelationship of pH, Organic Acids, and Phosphorus Concentration in Soil Solution of Rhizosphere and Non-rhizosphere of Wheat and Rice Crops, Commun. Soil Sci. Plan., 34, 231–245, https://doi.org/10.1081/css-120017428, 2011.
Biswas, S. S., Biswas, D. R., Ghosh, A., Sarkar, A., Das, A., and Roy, T.: Phosphate solubilizing bacteria inoculated low-grade rock phosphate can supplement P fertilizer to grow wheat in sub-tropical inceptisol, Rhizosphere, 23, 100556, https://doi.org/10.1016/j.rhisph.2022.100556, 2022.
Braun, S., McLaren, T. I., Frossard, E., Tuyishime, J. R. M., Börjesson, G., and Gustafsson, J. P.: Phosphorus desorption and isotope exchange kinetics in agricultural soils, Soil Use Manage., 38, 515–527, https://doi.org/10.1111/sum.12674, 2020.
Crisler, G. B., Perera, V., Hernandez, C. G., Orr, A., Davis, R., Moore, J., Smith, J., Varco, J., Schauwecker, T., Brown, A., Mlsna, T., and Mlsna, D.: Phosphate in Soils: An Undergraduate Exploration of Soil Texture, Chemistry, and Amendment, J. Chem. Educ., 97, 1077–1082, https://doi.org/10.1021/acs.jchemed.9b00529, 2020.
Duputel, M., Devau, N., Brossard, M., Jaillard, B., Jones, D. L., Hinsinger, P., and Gérard, F.: Citrate adsorption can decrease soluble phosphate concentration in soils: Results of theoretical modeling, Appl. Geochem., 35, 120–131, https://doi.org/10.1016/j.apgeochem.2013.03.018, 2013.
García, K. I., Quezada, G. R., Arumí, J. L., Urrutia, R., and Toledo, P. G.: Adsorption of Phosphate Ions on the Basal and Edge Surfaces of Kaolinite in Low Salt Aqueous Solutions Using Molecular Dynamics Simulations, J. Phys. Chem. C, 125, 21179–21190, https://doi.org/10.1021/acs.jpcc.1c05995, 2021.
Geng, Y., Pan, S., Zhang, L., Qiu, J., He, K., Gao, H., Li, Z., and Tian, D.: Phosphorus biogeochemistry regulated by carbonates in soil, Environ. Res., 214, 113894, https://doi.org/10.1016/j.envres.2022.113894, 2022.
Gérard, F.: Clay minerals, iron/aluminum oxides, and their contribution to phosphate sorption in soils – A myth revisited, Geoderma, 262, 213–226, https://doi.org/10.1016/j.geoderma.2015.08.036, 2016.
Helfenstein, J., Tamburini, F., von Sperber, C., Massey, M. S., Pistocchi, C., Chadwick, O. A., Vitousek, P. M., Kretzschmar, R., and Frossard, E.: Combining spectroscopic and isotopic techniques gives a dynamic view of phosphorus cycling in soil, Nat. Commun., 9, 3226, https://doi.org/10.1038/s41467-018-05731-2, 2018.
Helfenstein, J., Pistocchi, C., Oberson, A., Tamburini, F., Goll, D. S., and Frossard, E.: Estimates of mean residence times of phosphorus in commonly considered inorganic soil phosphorus pools, Biogeosciences, 17, 441–454, https://doi.org/10.5194/bg-17-441-2020, 2020.
Jalali, M. and Jalali, M.: Effect of Low-Molecular-Weight Organic Acids on the Release of Phosphorus from Amended Calcareous Soils: Experimental and Modeling, J. Soil Sci. Plant Nut., 22, 4179–4193, https://doi.org/10.1007/s42729-022-01017-1, 2022.
Lambers, H., and Plaxton, W. C.: Phosphorus: Back to the Roots, Annual Plant Reviews, 48, 3–22, 2015.
Khademi, Z., Jones, D. L., Malakouti, M. J., and Asadi, F.: Organic acids differ in enhancing phosphorus uptake by Triticum aestivum L. – effects of rhizosphere concentration and counterion, Plant Soil, 334, 151–159, https://doi.org/10.1007/s11104-009-0215-7, 2009.
McBeath, T. M., Lombi, E., McLaughlin, M. J., and Bünemann, E. K.: Exchangeability of orthophosphate and pyrophosphate in soils: a double isotopic labelling study, Plant Soil, 314, 243–252, 2008.
Menezes-Blackburn, D., Paredes, C., Zhang, H., Giles, C. D., Darch, T., Stutter, M., George, T. S., Shand, C., Lumsdon, D., Cooper, P., Wendler, R., Brown, L., Blackwell, M., Wearing, C., and Haygarth, P. M.: Organic Acids Regulation of Chemical-Microbial Phosphorus Transformations in Soils, Environ. Sci. Technol., 50, 11521–11531, https://doi.org/10.1021/acs.est.6b03017, 2016.
Paredes, C., Staunton, S., Durán, P., Rodríguez, R., and Mora, M. L.: Assessment of the combined effects of beef cattle manure and lemon peel waste on soil-plant biochemical properties and phosphorus uptake by ryegrass, Appl. Soil Ecol., 169, 104217, https://doi.org/10.1016/j.apsoil.2021.104217, 2022.
Penn, C. and Camberato, J.: A Critical Review on Soil Chemical Processes that Control How Soil pH Affects Phosphorus Availability to Plants, Agriculture, 9, 120, https://doi.org/10.3390/agriculture9060120, 2019.
Randriamanantsoa, L., Morel, C., Rabeharisoa, L., Douzet, J.-M., Jansa, J., and Frossard, E.: Can the isotopic exchange kinetic method be used in soils with a very low water extractable phosphate content and a high sorbing capacity for phosphate ions?, Geoderma, 200–201, 120–129, https://doi.org/10.1016/j.geoderma.2013.01.019, 2013.
Sindhu, S. S., Sehrawat, A., and Glick, B. R.: The involvement of organic acids in soil fertility, plant health and environment sustainability, Arch. Microbiol., 204, 720, https://doi.org/10.1007/s00203-022-03321-x, 2022.
Soumya, P. R., Vengavasi, K., and Pandey, R.: Adaptive strategies of plants to conserve internal phosphorus under P deficient condition to improve P utilization efficiency, Physiol. Mol. Biol. Pla., 28, 1981–1993, 2022.
Staunton, S. and Leprince, F.: Effect of pH and some organic anions on the solubility of soil phosphate: implications for P bioavailability, Eur. J. Soil Sci., 47, 231–239, https://doi.org/10.1111/j.1365-2389.1996.tb01394.x, 1996.
Wang, Y. and Lambers, H.: Root-released organic anions in response to low phosphorus availability: recent progress, challenges and future perspectives, Plant Soil, 447, 135–156, https://doi.org/10.1007/s11104-019-03972-8, 2019.