the Creative Commons Attribution 4.0 License.
the Creative Commons Attribution 4.0 License.
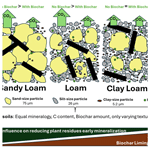
Biochar reduces early-stage mineralization rates of plant residues more in coarse-textured soils than in fine-textured soils – an artificial-soil approach
Thiago M. Inagaki
Simon Weldon
Franziska B. Bucka
Eva Farkas
Daniel P. Rasse
Quantifying the impact of biochar on carbon persistence across soil textures is complex, owing to the variability in soil conditions. Using artificial soils with precise textural and mineral compositions, we can disentangle the effects of biochar from the effects of soil particle size. We can show that biochar application significantly reduces the early-stage carbon mineralization rates of plant residues in various soil textures (from 5 % to 41 % clay) but more significantly in sandy soils. Clay and silt particles alone also reduce C mineralization, but the magnitude of the changes is negligible compared to the impact of biochar. This finding suggests that biochar can compensate for the lack of clay in promoting C persistence in soil systems. This short report contributes substantially to understanding soil texture and biochar application interactions.
- Article
(1357 KB) - Full-text XML
-
Supplement
(436 KB) - BibTeX
- EndNote
Biochar application in agriculture has been recognized to enhance carbon sequestration and to improve soil quality (Lehmann, 2007). In this regard, soil texture may play a fundamental role in the overall effectiveness of biochar in promoting carbon persistence, mainly due to influences on soil structural properties (Wang et al., 2017). However, the mechanisms behind the interaction between biochar and soil texture in promoting C storage still need to be fully understood due to the high heterogeneity in the soil properties and climate conditions of natural soils. As a result, the findings in the literature on this topic can vary depending on the specific experimental conditions used. For example, Gross et al. (2021) observed higher increases in soil organic carbon (SOC) stocks in more clayey soils than in sandy ones when evaluating field experiments in a meta-analysis. Contrastingly, the authors observed the opposite trend when considering non-field experiments. The reasons behind this were attributed to initial soil C contents as low-C soils have a higher potential for promoting increases in SOC stocks. However, the lack of research examining the effects of biochar on soil organic carbon (SOC) storage under diverse soil types and conditions undermines our understanding of these interactions. Likewise, biochar is recognized for promoting a liming effect and ameliorating acidic soils (Bolan et al., 2023). In contrasting comparisons, such liming effects are observed more intensely in clay soils than in sandy ones (Ajayi and Rainer, 2017), suggesting a potential synergistic effect of clay and biochar in promoting increases in soil pH. Nonetheless, there is a lack of studies systematically investigating the relationship between biochar-induced pH changes across a gradient of soil textures. High heterogeneity of soil properties across textural gradients usually challenges interpretations about the specific influence of soil particle size in these interactions.
In this sense, using artificial soil with known particle sizes and mineral compositions provides an excellent base for understanding the mechanisms behind soil processes (Pronk et al., 2012). These artificial soils can be mixed to mimic the composition of the typical arable soils of temperate regions, while their individual properties, like soil texture, can be freely adjusted (Bucka et al., 2021a). Artificial soils have already been used in research with mechanistic goals, such as studying the effects of microbial activity and mineral interactions on aggregation (Pronk et al., 2012; Vogel et al., 2014; Bucka et al., 2019), and in more applied uses, such as evaluating early soil development in post-mining soils (Bucka et al., 2021b). Therefore, this experimental setup provides a unique opportunity to investigate the intricate relationships between biochar application and soil particle size distribution, an area that remains largely unexplored.
This short communication explored the interactions between soil texture and biochar application in the early-stage soil organic matter mineralization using artificial soils with precise mineral and textural compositions in a controlled microcosm. We hypothesized that biochar could reduce organic soil mineralization, especially in coarser-textured soils.
2.1 Artificial-soil preparation: texture range
To produce the different textures of artificial soils, we have used quartz grains of varying particle sizes (EUROQUARZ, Laußnitz, Germany, and Quarzwerke, Frechen, Germany). We added goethite (<6 µm), illite (<7 µm), and bentonite (<63 µm) (ASPANGER, Aspang, Austria) to create soil-like reactive surfaces in the fine-silt and clay size fractions. The C content of the artificial soils was considered to be negligible due to the insignificant C concentration of the ingredients. The soil mixtures were prepared according to the proportions presented in Table 1. The texture classes were defined as (1) loamy sand, (2) sandy loam, (3) loam, (4) clay loam, and (5) silty clay.
The artificial soils were prepared by mixing the ingredients in a dry state, ranging from coarser- to finer-scale particles. As each component was added, 10 manual turns were executed to combine them. After all the ingredients were mixed, the containers were added to a horizontal shaker and shaken overnight at 140 rpm. After the overnight shaking period, each soil mix container was manually turned 30 times.
2.2 Incubation experiment and treatments
The experimental setup was a 5×2 factorial design testing five different textures of artificial soils with and without biochar application with three replicates. For the incubation experiment, we used 20 g of artificial soil per experimental unit in 120 mL glass flasks. We added ball-milled air-dried clover biomass (Trifolium sp.) (C : N ratio of 18) as an organic matter source to all samples at a rate of 27 mg C g−1 soil to mimic the natural background organic carbon (OC) content of arable topsoils. Ball-milled organic matter was chosen to enhance the development of these soils since the inorganic components added were C-free. We have used dissolved organic matter extracted from a local crop field as an inoculum of soil microorganisms for the artificial soils, applied at a rate of 0.06 mL g−1 soil, in accordance with Pronk et al. (2012). The artificial soils were incubated under 60 % of the maximum water-holding capacity to ensure microbial activity (Fig. S1 in the Supplement). The water used to add the inoculum was accounted for in the amount added. Biochar was produced from Norwegian spruce at 700 °C, with 7 min holding time, and was added to the soil at a rate of 50 mg biochar g−1 soil. Biochar had a C content of 95.6 %. The added biochar had a size distribution between 0.063 and 2 mm, controlled by sieving before addition to the soil. The basic biochar properties are described in Table S1 in the Supplement.
2.3 CO2 respiration measurements
We measured CO2 production over 115 h using an automated incubation system, described in detail by Molstad et al. (2007), with modifications as described in Molstad et al. (2016). The system consists of an autosampler (CTC PAL) connected to an Agilent gas chromatograph (Model 7890A, Agilent, Santa Clara, CA, USA). The system allows for high-resolution analysis of headspace gas concentrations in airtight 120 mL serum bottles. Corrections were applied to adjust for sampling dilution, leakage, and CO2 equilibrium state as a function of the material pH and soil solution volume (Appelo and Postma, 2005). We monitored the weight of the samples during the incubation period to check whether adjusting the water content was necessary. Since this was a relatively short incubation (5 d) conducted in a closed environment, the water loss was minimal, and we did not need to refill the flasks to maintain the initial moisture content.
2.4 Soil pH analysis
Soil pH was measured in a 0.01 M CaCl2 solution according to the ISO 10390:2021.
2.5 Statistical analysis
We performed the Shapiro–Wilk test to confirm the data's normal distribution. Once the data normality was confirmed, we conducted a two-way analysis of variance (ANOVA) and a post-hoc test using Fisher's least significant difference (LSD) test to compare biochar and control treatments within each soil textural group. We used linear regressions correlating the increase in clay and silt content with the variables of interest to compare the different textural classes.
3.1 Early plant mineralization affected by particle size and biochar applications
We observed significant effects of both biochar application and texture on C mineralization and a significant interaction between these variables in the analysis of variance (ANOVA) at p<0.01. Biochar application significantly reduced C mineralization rates compared to the control in the coarser-textured soils, namely (1) loamy sand, (2) sandy loam, and (3) loam (Fig. 1), according to the LSD test at p<0.05. However, no differences were observed between biochar and control treatments for the finer-textured soils, namely (4) clay loam and (5) silty clay (Fig. 1).
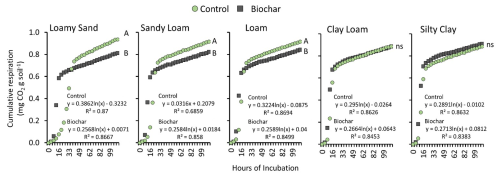
Figure 1Biochar effect on the CO2 cumulative respiration in artificial soils with different textures in a 5 d incubation experiment. For a given soil, different uppercase letters indicate a significant difference (p<0.05) in the cumulative respiration between the control and biochar-amended soils according to Fisher's least significant difference (LSD) test. Each point is the average of three replicates. Variations between replicates are not visible in the graph scale due to the uniformity of the artificial-soil composition.
The reduction in C mineralization promoted by biochar in the loamy sand (soil 1) was over 6-fold higher than in the silty-clay soil (soil 5). The influence of clay and silt content on C mineralization also differed depending on whether or not biochar was applied (Fig. 2). However, the impact of soil texture was minimal in magnitude compared to the changes promoted by biochar addition. The control samples' clay and silt contents generally decreased C mineralization (Fig. 2). Every milligram of silt- and clay-sized particles in the artificial soils reduced clover residue mineralization by 0.00007 mg g−1 soil (Fig. 2) under a constant moisture level (60 % of the water-holding capacity), given the slope of the equation. After a 5 d incubation in the silty-clay soil (soil 5), the clover mineralization was 0.06 mg CO2 g−1 soil lower than in the loamy-sand soil (soil 1).
3.2 Soil pH affected by particle size and biochar application
Soil pH (CaCl2) was significantly influenced by increased soil texture and biochar application (Fig. 3). Overall, increases in pH due to biochar addition were higher with increasing clay and silt contents, i.e., by 0.68 in the silty clay and 0.24 in the loamy sand (Fig. 3a). The increase in silt and clay particles alone also promoted increases in soil pH (p<0.05) (Fig. 3b). Given the slope of the linear regression equation, every milligram of the clay and silt fraction promoted an increase of 0.0003 in soil pH (CaCl2). This increase was 3 times higher (0.009) when biochar was applied (p<0.01) (Fig. 2b).
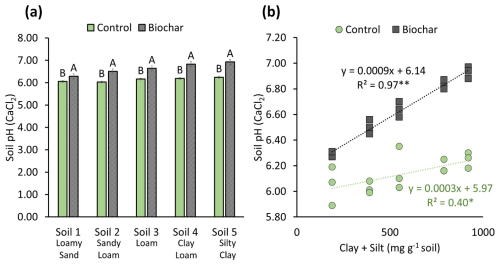
Figure 3Soil pH as affected by the biochar application and soil texture in artificial soils incubated for 5 d. (a) Influence of biochar application to soil pH (CaCl2) under different textures. For a given soil, different letters indicate significant differences according the LSD test at p<0.05. (b) Influence of soil texture (clay and silt proportion) on soil pH (CaCl2) in soils with and without biochar. Significant at p<0.01. * Significant at p<0.05.
4.1 Interactions of clay and silt content and biochar application in reducing early mineralization of plant residues
The significant interaction between biochar application and soil texture suggests that the extent of biochar's impact on the mineralization of plant residues depends on soil texture, as observed by different studies (Butnan et al., 2017; Gross et al., 2021; Wang et al., 2017). On the other hand, soil texture alone had minor effects on C mineralization. The differences in the magnitude of early plant mineralization depending on soil texture suggest that biochar had a higher impact on reducing early C mineralization from clover residues in sandier textures than in clay-rich soils, which has also been observed under natural soil conditions (Butnan et al., 2017). Biochar has known benefits in terms of enhancing soil properties associated with promoting C protection, such as aggregation (Wang et al., 2017; Juriga et al., 2021), sorption capacity (Siedt et al., 2021), water retention, and porosity (Obia et al., 2016) in natural soils. Such effects may have potentially influenced the decrease in the early mineralization of the added plant residues since the improvement of the soil structure in artificial soils, such as through aggregate formation, is already observed within the first days of their development (Pronk et al., 2012).
Improvements in soil structure and C stability upon biochar application are known to happen at a higher intensity in sandy soils compared to in more clayey ones (Buchkina et al., 2017; Butnan et al., 2017), which may justify biochar's better performance in reducing early plant mineralization in sandy soils in our study. Our observations corroborate general trends observed in non-field experiments (Gross et al., 2021), with higher biochar performance in sandy textures compared to in clay. On the other hand, other experiments in incubation setups, such as that of Wang et al. (2017) and the field experiment observations made by Gross et al. (2021), suggest an opposite trend. The reasons for these discrepancies seem to depend mainly on the initial condition of the evaluated soils. For example, in the incubation setup, comparing contrasting textures in Wang et al. (2017), the unamended samples showed no difference in terms of water-stable aggregates despite their contrasting textures, and the C content was higher in the sandy soil. This experiment resulted in higher impacts of biochar application to clay soil structure and C storage. On the other hand, in our experiment, the unamended samples with higher clay content had a significantly higher water-holding capacity (Fig. S1), indicating an overall better structure than the sandy soils, and the organic matter added to them was the same for all soils, which resulted in a better performance of biochar in more sandy textures. Therefore, the impacts of biochar on reducing C mineralization in our experiment suggest that the amendment can compensate for the lack of soil structural quality in promoting early C persistence in soils.
Likewise, Gross et al. (2021) pointed out the low initial soil C content of sandier textures as a reason for observing higher SOC sequestration potential in non-field experiments. The contrast with field experiments can also be due to factors that happen only in field trial setups, such as biochar leaching in sandy soils; these are factors which are not observed under pot or incubation setups. Therefore, our interpretations are more focused on the intrinsic relationships between soil texture and biochar and their impacts on C mineralization in a controlled environment. However, climatic factors and long-term effects need to be confirmed through long-term field trials. Nonetheless, since most of the works evaluating the performance of biochar in different soil textures vary with regard to other factors such as C content, mineral composition, and structural properties, our work offers important insights into understanding these interactions of biochar with soil particle size in C mineralization.
Also, the increase in fine particles in soils is assumed to decrease organic matter decomposability due to the increased opportunity for physicochemical protection (Hassink, 1992; Kravchenko and Guber, 2017), which justifies the minor reductions observed in the mineralization rates caused by increased silt and clay fractions (Fig. 2). However, despite the significant correlations, the magnitude of changes in mineralization caused by soil texture was negligible in this experiment, given the low slope values (Fig. 2). Therefore, our results suggest that soil particle size (i.e., texture) has played a milder overall role than the application of biochar in our short-term study.
In particular, in sand-rich soil, where the available mineral surface area (as well as the permanently charged surfaces of clay minerals) is low, biochar can deliver additional surface area for adsorption processes. The biochar effect is probably reduced by increasing clay content as the higher clay mineral surface area and the smaller pores may overrule the biochar effect on the physicochemical protection of organic matter (OM). Since the biochar added in our experiment was a standard size, between 0.063 and 2 mm, we can argue that the biochar composition was foremostly responsible for the observed effects on plant mineralization rates rather than a change in the proportion of particle sizes in each soil texture. Nevertheless, the results with regard to texture controlling soil organic matter mineralization are contrasting in the literature, with results being dependent on soil moisture and other experimental conditions (Li et al., 2020, 2022). Our experiment using artificial soils with precise compositions and an early formation stage helped shed light on the effects of textures and particle size on organic matter decomposition.
4.2 Interactions between clay and biochar in enhancing soil pH and the consequences of early mineralization of plant residues
The pronounced biochar effect on pH is likely due to its high acid-buffering capacity. Biochar consists of both alkaline functional groups and mineral ash containing both base cations and secondary carbonates (Fidel et al., 2017), explaining why the biochar and clay and silt content have increased soil pH. The higher pH of the soil solution promotes the dissolution of CO2 gas, thereby reducing the amount of gas released from soils. This can result in a measurement artifact of lower-than-actual mineralization rates in pH-enhanced soils (Ma et al., 2013). However, this effect was accounted for in our study as mineralization rates were corrected for amounts of CO2 dissolved in solution as a function of solution volume and pH (Appelo and Postma, 2005).
The liming effect of biochar is recognized in a variety of soils (Bolan et al., 2023), with an overall higher effect in clay than in sandy soils (Ajayi and Rainer, 2017), which agrees with the findings of our experiment (Fig. 3a). Nonetheless, soil comparisons under natural conditions also vary in terms of mineral composition, making the isolated textural effect less clear (Ajayi and Rainer, 2017). In this sense, our artificial-soil setup helps to clarify this effect by demonstrating a significant role of clay and silt fractions in increasing the soil pH (Fig. 3b) in soils with the same mineral composition and organic matter content. Our results also showcase that this influence of clay and silt particles on soil pH is significantly boosted in biochar-amended soils as the slope of the linear correlation was 3 times higher in the biochar treatment than in the control (Fig. 3b).
Direct sorption of CO2 into biochar has also been reported alongside N2O adsorption. It can also be considered to be a mechanism through which biochar can reduce the presence of these gases in the atmosphere (Cornelissen et al., 2013). This effect was probably counterbalanced by a higher clay and silt content in the soils, which may have protected the biochar surfaces and thus prevented CO2 sorption, justifying the relatively greater increases in C mineralization in relation to the function of clay and silt in biochar-amended soils (Fig. 2). A higher soil pH has been shown to increase the mineralization of organic residues in soils (Li et al., 2007; Khalil et al., 2005). This effect has been attributed to a higher microbial biomass and a more bacteria-dominated microbial community in high-pH soils (Li et al., 2007; Laura 1976). In addition, a high pH causes stress for the microbial community, resulting in lower carbon use efficiency, translating into lower assimilation for organic C and a higher CO2 release (Li et al., 2007). Some authors propose that the higher mineralization of organic matter may foster the supply of nutrients like nitrogen and sulfur, further stimulating microbial activity (Barrow and Hartemink, 2023). Due to mineral surface availability, a high clay and silt content may have leveled out the biochar effect. Sorption of cationic nutrients on those mineral surfaces may have led to limited availability, reducing the CO2 release in the mixtures with biochar and a high clay and silt content (Barrow and Hartemink, 2023). Therefore, we encourage further studies exploring the specific roles of microorganisms in artificial-soil setups to understand how they can influence these microcosms and how these results compare to natural conditions.
In conclusion, our study suggests that biochar has a greater capacity to promote reductions in the early mineralization of clover residues in sandy soils. This potential diminishes as the clay and silt content increases in the soil. Here, we show that the artificial-soil setup using a precise composition is a suitable tool for investigating the effects of factors that are difficult to control in natural soils due to their heterogeneity. Our mineralization rates are comparable to other studies using artificial soils (Vogel et al., 2014; Bucka et al., 2021a, b) but can differ from experiments done under natural conditions (Gross et al., 2021). Therefore, interpretations made here are mainly applied to understand the intrinsic relationships between soil particle size and the biochar application to soil C persistence but should not be accounted for for purposes of quantifying C emissions from determined soil types or land uses.
We report the results of a screening study using an experimental setup for high-frequency short-term measurements. We have observed significant effects of biochar content in reducing the early mineralization of clover residues in artificial soils. Soil particle size influences the decreasing C mineralization, but the magnitude of these changes is negligible compared to biochar effects. Biochar has demonstrated the potential to reduce the early mineralization of plant residues, especially in sandy soils. This effect is diminished with an increased clay and silt content in the soil, suggesting that biochar may compensate for the lack of clay in sandy soils by promoting lower mineralization of organic matter. Results regarding soil organic matter persistence and carbon sequestration must be confirmed using longer-term experiments. However, this first set of results demonstrates the power of using standardized multi-texture artificial soils to study biochar and organic matter interactions in soils. Here, we suggest that this artificial-soil setup is a valuable platform for understanding mechanisms associated with biochar in soils.
The code used for data analysis is available from the corresponding authors upon reasonable request.
The dataset supporting the findings of this study is available at https://doi.org/10.5281/zenodo.14780288 (Inagaki et al., 2025).
The supplement related to this article is available online at: https://doi.org/10.5194/soil-11-141-2025-supplement.
TMI designed the experiment, carried out the analyses, and wrote the paper with the support of SW, FBB, EF, and DPR at every step.
The contact author has declared that none of the authors has any competing interests.
Publisher’s note: Copernicus Publications remains neutral with regard to jurisdictional claims made in the text, published maps, institutional affiliations, or any other geographical representation in this paper. While Copernicus Publications makes every effort to include appropriate place names, the final responsibility lies with the authors.
The authors thank Mag. Engelbert Pürrer and ASPANGER (Aspang, Austria) for providing the bentonite and illite used to prepare the artificial soils.
This open-access publication was funded by Goethe University Frankfurt.
This paper was edited by Cornelia Rumpel and reviewed by Marie-Liesse Aubertin and one anonymous referee.
Ajayi, A. E. and Rainer, H.: Biochar-induced changes in soil resilience: effects of soil texture and biochar dosage, Pedosphere, 27, 236–247, 2017.
Appelo, C. and Postma, D.: Geochemistry, Groundwater and Pollution, 2nd ed., CRC Press, 683 pp., https://doi.org/10.1201/9781439833544, 2005.
Barrow, N. J. and Hartemink, A. E.: The effects of pH on nutrient availability depend on both soils and plants, Plant Soil, 487, 21–37, https://doi.org/10.1007/s11104-023-05960-5, 2023.
Bolan, N., Sarmah, A. K., Bordoloi, S., Bolan, S., Padhye, L. P., Van Zwieten, L., Sooriyakumar, P., Khan, B. A., Ahmad, M., and Solaiman, Z. M.: Soil acidification and the liming potential of biochar, Environ. Pollut., 317, 120632, https://doi.org/10.1016/j.envpol.2022.120632, 2023.
Buchkina, N., Balashov, E., Šimanský, V., Igaz, D., and Horák, J.: Changes in biological and physical parameters of soils with different texture after biochar application, Selskokhozyaistvennaya Biologiya (Agricultural Biology), 52, 471–477, 2017.
Bucka, F. B., Kölbl, A., Uteau, D., Peth, S., and Kögel-Knabner, I.: Organic matter input determines structure development and aggregate formation in artificial soils, Geoderma, 354, 113881, https://doi.org/10.1016/j.geoderma.2019.113881, 2019.
Bucka, F. B., Felde, V. J. M. N. L., Peth, S., and Kögel-Knabner, I.: Disentangling the effects of OM quality and soil texture on microbially mediated structure formation in artificial model soils, Geoderma, 403, 115213, https://doi.org/10.1016/j.geoderma.2021.115213, 2021a.
Bucka, F. B., Pihlap, E., Kaiser, J., Baumgartl, T., and Kögel-Knabner, I.: A small-scale test for rapid assessment of the soil development potential in post-mining soils, Soil Till. Res., 211, 105016, https://doi.org/10.1016/j.still.2021.105016, 2021b.
Butnan, S., Deenik, J. L., Toomsan, B., and Vityakon, P.: Biochar properties affecting carbon stability in soils contrasting in texture and mineralogy, Agriculture and Natural Resources, 51, 492–498, 2017.
Cornelissen, G., Rutherford, D. W., Arp, H. P., Dorsch, P., Kelly, C. N., and Rostad, C. E.: Sorption of pure N2O to biochars and other organic and inorganic materials under anhydrous conditions, Environ. Sci. Technol., 47, 7704–7712, https://doi.org/10.1021/es400676q, 2013.
Fidel, R. B., Laird, D. A., Thompson, M. L., and Lawrinenko, M.: Characterization and quantification of biochar alkalinity, Chemosphere, 167, 367–373, https://doi.org/10.1016/j.chemosphere.2016.09.151, 2017.
Gross, A., Bromm, T., and Glaser, B.: Soil Organic Carbon Sequestration after Biochar Application: A Global Meta-Analysis, Agronomy-Basel, 11, 2474, https://doi.org/10.3390/agronomy11122474, 2021.
Hassink, J.: Effects of Soil Texture and Structure on Carbon and Nitrogen Mineralization in Grassland Soils, Biol. Fert. Soils, 14, 126–134, https://doi.org/10.1007/Bf00336262, 1992.
Inagaki, T. M., Weldon, S., Bucka, F., Farkas, E., and Rasse, D.: Dataset of: Biochar reduces early-stage mineralization rates of plant residues more in coarse than fine-texture soils – an artificial soil approach, in Soil, Zenodo [data set], https://doi.org/10.5281/zenodo.14780288, 2025.
Juriga, M., Aydin, E., Horák, J., Chlpík, J., Rizhiya, E. Y., Buchkina, N. P., Balashov, E. V., and Simansky, V.: The importance of initial application and reapplication of biochar in the context of soil structure improvement, J. Hydrol. Hydromech., 69, 87–97, https://doi.org/10.2478/johh-2020-0044, 2021.
Khalil, M. I., Hossain, M. B., and Schmidhalter, U.: Carbon and nitrogen mineralization in different upland soils of the subtropics treated with organic materials, Soil Biol. Biochem., 37, 1507–1518, https://doi.org/10.1016/j.soilbio.2005.01.014, 2005.
Kravchenko, A. N. and Guber, A. K.: Soil pores and their contributions to soil carbon processes, Geoderma, 287, 31–39, https://doi.org/10.1016/j.geoderma.2016.06.027, 2017.
Lehmann, J.: A handful of carbon, Nature, 447, 143–144, https://doi.org/10.1038/447143a, 2007.
Li, H. C., Van den Bulcke, J., Wang, X. L., Gebremikael, M. T., Hagan, J., De Neve, S., and Sleutel, S.: Soil texture strongly controls exogenous organic matter mineralization indirectly via moisture upon progressive drying – Evidence from incubation experiments, Soil Biol. Biochem., 151, 108051, https://doi.org/10.1016/j.soilbio.2020.108051, 2020.
Li, H. C., van den Bulcke, J., Kibleur, P., Mendoza, O., De Neve, S., and Sleutel, S.: Soil textural control on moisture distribution at the microscale and its effect on added particulate organic matter mineralization, Soil Biol. Biochem., 172, 108777, https://doi.org/10.1016/j.soilbio.2022.108777, 2022.
Li, X. G., Rengel, Z., and Mapfumo, E.: Increase in pH stimulates mineralization of “native” organic carbon and nitrogen in naturally salt-affected sandy soils, Plant Soil, 290, 269–282, https://doi.org/10.1007/s11104-006-9158-4, 2007.
Ma, J., Wang, Z. Y., Stevenson, B. A., Zheng, X. J., and Li, Y.: An inorganic CO2 diffusion and dissolution process explains negative CO2 fluxes in saline/alkaline soils, Sci. Rep.-UK, 3, 2025, https://doi.org/10.1038/srep02025, 2013.
Molstad, L., Dorsch, P., and Bakken, L. R.: Robotized incubation system for monitoring gases (O2, NO, N2O N2) in denitrifying cultures, J. Microbiol. Meth., 71, 202–211, https://doi.org/10.1016/j.mimet.2007.08.011, 2007.
Molstad, L., Dörsch, P., and Bakken, L.: Improved robotized incubation system for gas kinetics in batch cultures, Researchgate, 10, https://doi.org/10.13140/RG.2.2.30688.07680, 2016.
Obia, A., Mulder, J., Martinsen, V., Cornelissen, G., and Borresen, T.: In situ effects of biochar on aggregation, water retention and porosity in light-textured tropical soils, Soil Till. Res., 155, 35–44, https://doi.org/10.1016/j.still.2015.08.002, 2016.
Pronk, G. J., Heister, K., Ding, G. C., Smalla, K., and Kögel-Knabner, I.: Development of biogeochemical interfaces in an artificial soil incubation experiment; aggregation and formation of organo-mineral associations, Geoderma, 189, 585–594, https://doi.org/10.1016/j.geoderma.2012.05.020, 2012.
Siedt, M., Schaffer, A., Smith, K. E. C., Nabel, M., Ross-Nickoll, M., and van Dongen, J. T.: Comparing straw, compost, and biochar regarding their suitability as agricultural soil amendments to affect soil structure, nutrient leaching, microbial communities, and the fate of pesticides, Sci. Total Environ., 751, 141607, https://doi.org/10.1016/j.scitotenv.2020.141607, 2021.
Vogel, C., Babin, D., Pronk, G. J., Heister, K., Smalla, K., and Kögel-Knabner, I.: Establishment of macro-aggregates and organic matter turnover by microbial communities in long-term incubated artificial soils, Soil Biol. Biochem., 79, 57–67, 2014.
Wang, D. Y., Fonte, S. J., Parikh, S. J., Six, J., and Scow, K. M.: Biochar additions can enhance soil structure and the physical stabilization of C in aggregates, Geoderma, 303, 110–117, https://doi.org/10.1016/j.geoderma.2017.05.027, 2017.