the Creative Commons Attribution 4.0 License.
the Creative Commons Attribution 4.0 License.
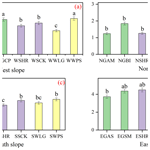
Response of soil nutrients and erodibility to slope aspect in the northern agro-pastoral ecotone, China
Yuxin Wu
Guodong Jia
Xinxiao Yu
Honghong Rao
Xiuwen Peng
Yusong Wang
Yushi Wang
Xu Wang
Soil erosion, considered a major environmental and social problem, leads to the loss of soil nutrients and the degradation of soil structure and impacts plant growth. However, data on the effects of land use changes caused by vegetation restoration on soil nutrients and erodibility for different slope aspects are limited. This study was conducted to detect the response of soil nutrients and erodibility to slope aspect in a typical watershed in the northern agro-pastoral ecotone in China. The following indexes were used to determine the improvement in soil nutrients and erodibility through a weighted summation method: the comprehensive soil nutrient index and the comprehensive soil erodibility index. The results showed that the vegetation types with the highest comprehensive soil quality index (CSQI) values on western, northern, southern, and eastern slopes were Pinus sylvestris and Astragalus melilotoides (1.45), Caragana korshinskii and Capillipedium parviflorum (2.35), Astragalus melilotoides (4.78), and Caragana korshinskii and Lespedeza bicolor (5.00), respectively. Slope aspect had a significant effect on understory vegetation characteristics, soil nutrients, and soil erodibility. Understory vegetation and soil characteristics explained 50.86 %–74.56 % of the total variance in soil nutrients and the erodibility. Mean weight diameter and total phosphorus were the main factors that affected the CSQI for different slope aspects. Our study suggests that the combinations of species, such as C. korshinskii and L. bicolor, were the optimal selection to improve soil nutrients and soil erodibility for any slope aspect.
- Article
(4025 KB) - Full-text XML
-
Supplement
(716 KB) - BibTeX
- EndNote
Soil erosion, considered a major environmental and social problem, leads to the loss of soil nutrients and the degradation of soil structure and influences the functional capacity of soils on a global scale (Singh and Panda, 2017; Wen et al., 2021). Vegetation restoration is an important method of ecological restoration that aims to control soil erosion and prevent soil degradation (Schmiedel et al., 2017; Zhang et al., 2021). Vegetation restoration can improve the soil structure and nutrients, which in turn promotes the restoration of soil quality and function (Guo et al., 2021; Li et al., 2017). Changes in land use due to vegetation restoration play important roles in improving the environment and ecosystem function as well as improving soil quality and soil nutrient cycling (Akiyama and Kawamura, 2007; Singh and Gupta, 2018).
Previous studies have shown that the plants selected for vegetation restoration projects drive land use change and alter soil properties, thus affecting soil erodibility (Wang et al., 2019b, a; Zhang et al., 2019). Many studies have also elucidated the influences of land use change on soil nutrients and have confirmed that revegetation is an effective way to enhance soil nutrients (Huang et al., 2020; Li et al., 2020; Yang et al., 2021; Zhu et al., 2020). Most studies have only focused on one aspect; thus, they lack a comprehensive consideration and evaluation of the impact of land use changes caused by vegetation restoration on soil nutrients and erodibility. However, it is not clear which of the plants selected for restoration are the most effective at enhancing soil nutrients and reducing soil erodibility. This lack of a comprehensive understanding prevents us from gaining the best ecological benefits from vegetation restoration. Therefore, studies must be conducted on the response of soil nutrients and erodibility to different vegetation restoration types.
Soil erodibility is the sensitivity of the soil surface to erosion processes (Batista et al., 2023; Bryan et al., 1989). It is a necessary parameter for establishing soil loss equations and erosion models. There is currently no soil erosion model that can accurately predict soil erosion, although there are many related models (de Vente et al., 2013, 2008). At present, the soil erodibility K-factor, as defined in the universal soil loss equation (USLE), is the most widely used measure (Wischmeier and Smith, 1978). In addition to K, other soil indexes have been adopted to quantify soil erodibility, including the saturated hydraulic conductivity (SHC), soil disintegration rate (SDR), mean weight diameter (MWD), soil structural stability index (SSSI), clay ratio (CR), and soil organic carbon cementing agent index (SCAI) (Dong et al., 2022a; Guo et al., 2021; Wang et al., 2018; Zhang et al., 2019). Soil organic carbon, nitrogen, and phosphorus as well as their stoichiometry are also essential for assessing soil quality as well as ecosystem productivity and functionality (Borchard et al., 2017; Li et al., 2020; Masciandaro and Ceccanti, 1999; Schloter et al., 2003). A single index cannot fully reflect all soil properties; therefore, it is necessary to develop a comprehensive soil index using several related indicators.
In addition to soil properties, topographic factors also significantly affect soil nutrients and erodibility (Bangroo et al., 2017; Nabiollahi et al., 2018; Qin et al., 2016; Zhang et al., 2018). The slope aspect can affect the growth of plants due to a combination of factors, such as light, temperature, wind speed, and precipitation, which can cause significant changes in the ecological relationship between plants and the environment (Li et al., 2018; Tamene et al., 2020; Zhang et al., 2020). This is especially true for harsh climates such as cold, dry alpine regions in the north, in which plants are more sensitive to environmental changes. However, the optimal vegetation restoration type has primarily been studied in relation to slope gradient and slope position (Dong et al., 2022a; Guo et al., 2021; Wen et al., 2021). Systematic evaluations of the effects of land use changes caused by vegetation restoration on soil nutrients and erodibility for different slope aspects are rare. Therefore, the classification of slope aspect needs to be further refined to elucidate the effect of slope aspect on the changes in soil nutrients and erodibility caused by revegetation.
The ecologically fragile northern agro-pastoral zone in China is located in an erosion zone affected by both wind and water; soil erosion in this zone is considered very serious (Guo et al., 2019). Recently, the Chinese government has planned and carried out a series of ecological restoration projects in this region, including the Beijing–Tianjin Wind and Sand Source Control Project, the Beijing–Hebei Water Protection Forest Project, and the Sebei Forest Plantation Afforestation Project. These ecological restoration projects have effectively reduced land erosion and desertification and have significantly delayed the onslaught of wind and sand (S. Wang et al., 2021; Zeng et al., 2014; Zhang et al., 2017). However, the method used for afforestation, which mainly consists of plantations, is affected by differences between the habitats in water, heat, wind, and sand, making it difficult to achieve vegetation restoration in some ecologically fragile areas, and the selection of suitable tree species is still equivocal.
Based on the abovementioned scientific gaps, we hypothesize that both the slope aspect and land use type can significantly affect the soil structure and properties, thus influencing soil nutrients and erodibility, under vegetation restoration. We further hypothesize that a western slope may have a lower comprehensive soil quality index compared to other slope aspects. Therefore, we selected four slope aspects (west, north, south, and east) that have four different land-use types (degraded land, grasslands, shrublands, and woodlands) in a typical watershed in the northern agro-pastoral ecotone for three specific purposes: (1) to determine the impacts of different vegetation types present on slopes with different aspects on soil nutrient improvement and soil erodibility enhancement; (2) to determine the key influencing factors affecting soil nutrients and erodibility for the four slope aspects; and (3) to provide optimal revegetation models for improving soil nutrients and reducing soil erodibility for different slope aspects.
2.1 Study area
This study was conducted in the Yangcaogou watershed (41∘4′–41∘8′ N, 114∘58′–115∘2′ E; Fig. 1), Chongli District, Zhangjiakou City, Heibei Province, China. The watershed is located in a typical ecological transition zone in the agro-pastoral ecotone in northern China (Wu et al., 2023). The study site spans an area of 10.6 km2 with an altitude ranging from 1084 to 1575 m. It has a typical temperate continental monsoon semi-arid climate with an annual average temperature of 3.5 ∘C. The average annual rainfall is 401.6 mm. The rainy season occurs from June to September (Chang et al., 2021; Guo et al., 2019). The main soil type is classified as chestnut soil in both the Chinese Soil Taxonomy and the World Reference Base for Soil Resources (Schad, 2017). Most of the study area consists of Proterozoic soil rock formations. Owing to irrational human reclamation and grazing, there is very serious soil and gully erosion. Over the past decade, due to the implementation of the Beijing–Tianjin Sandstorm Source Control Project, soil erosion and desertification has been effectively mitigated (S. Wang et al., 2020). However, native plant populations have been diminished; instead, the area is planted with trees, shrubs, and herbs.
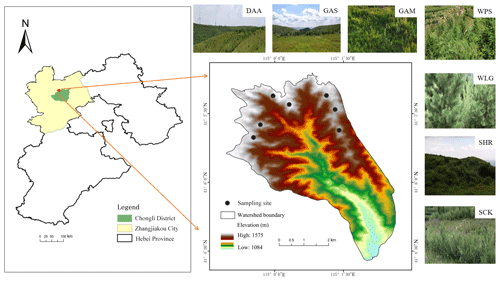
Figure 1Location map of the sampling points in the study area. The first letter of each sampling point – D, G, S, or W – represents degraded land, grassland, shrubland, or woodland, respectively. The sampling sites were as follows (from west to east): DAA, degraded land; GAS, Artemisia sacrorum; GAM, Astragalus melilotoides; WPS, Pinus sylvestris; WLG, Larix gmelinii; SHR, Hippophae rhamnoides; SCK, Caragana korshinskii.
2.2 Selection of sites and determination of the slope aspect
The study was conducted during the 2021 growing season. A comprehensive field survey of the dominant plant species and soil properties of each of the following land use types was conducted: degraded land, grasslands, shrublands, and woodlands in the Yangcaogou watershed. The grasslands, shrublands, and woodlands have been restored from degraded land over the past 12 years. The degraded land (which had suffered a loss of soil material due to wind and water erosion and a degradation of physical, chemical, and biological properties of the soil) was previously degraded cropland. All land use types were vegetated and restored using engineering measures such as fish scale pits (Z.-J. Wang et al., 2014) and parallel ditches (Barua and Alam, 2013).
In addition to the degraded land, the other three land use types were all sampled along four slope aspects on the E, W, N, and S slopes. This included 28 sample sites (20 m × 20 m) on degraded land, two grasslands, two shrublands, and two woodlands for each slope aspect. Three sampling quadrats (1 m × 1 m) were set up in each sample site to investigate and record the species, height, richness, coverage, aboveground biomass, belowground biomass, and litter biomass of herbs. Height was measured as the average height of herbs in the sample. Biomass coverage was determined following the visual method (Proulx and Mazumder, 1998). Richness was calculated by measuring the number of individuals of each herb in the quadrat and converting this into a percentage of the total number of individuals of all herbs in the quadrat (Dou et al., 2023). Belowground biomass and soil samples were collected with a 9 cm diameter soil drill. The measured land use types, major plant species, and understory vegetation characteristics at each selected field site are listed in Table S1 in the Supplement.
Following the methods described by Yimer et al. (2006), study sites were selected on slopes with different aspects (east, west, north, or south), with each slope including the four land use types. Eastern, western, northern, and southern slopes are also known as semi-sunny, semi-shady, shady, and sunny slopes (Che et al., 2022; Z. Chen et al., 2021). In this region, four unrestored degraded lands were selected as representatives from a western slope. The slope gradients and positions were similar for all selected sample sites (Fig. 1).
2.3 Soil sampling and analysis
Three quadrats were selected at each site to investigate the vegetation and collect soil samples. For each sampling point, a steel cutting ring (100 cm3) was used to obtain 75 soil samples (25 sites × 3 sampling points). The saturated hydraulic conductivity of the soil was evaluated using the constant head permeability test (Chandler and Chappell, 2008). The mean weight diameter was measured by using screens with different pore sizes (0.25, 0.50, 1.00, 2.50, and 5.00 mm) (Campo et al., 2008). After air-drying via dry screening, 50 g of the soil samples were placed on the sieve of a soil aggregate analyzer (TTF-100 model, China) and then completely immersed in water and shaken up and down 30 times for 1 min (B. Wang et al., 2014). After the shaking, the samples were removed from the settling cylinder, and the remaining aggregates on each sieve were put into an aluminum box for drying. Finally, the samples were weighed and the dried aggregates were recorded.
Soil characteristics of different vegetation types on slopes with different aspects are listed in Table S2. Topsoil samples were collected from 0–10 cm depth using a cutting ring. Samples were brought back to the lab, where they were oven dried at 105 ∘C for 24 h. Then, the soil bulk density (SBD) (Lardy et al., 2022; Moreira et al., 2020) and soil capillary porosity (SCP) (Singh and
Pollard, 1958) were measured. In addition, 225 mixed soil samples (25 sites × 3 quadrats/site × 3 samples/quadrat) were collected as soil samples. Among them, the particle size distributions of the clay content (Cl), silt content (Si), and sand content (Sa) were determined by a Microtrac S3500 laser particle sizer (Malvern 3000, UK). Total nitrogen (TN) and total phosphorus (TP) were determined by the dichromate oxidation (Bremner, 1996) and HClO4-H2SO4 methods (Kisand, 2005), respectively. Soil pH (Cornfield, 1954) was determined using a pH meter at a soil:water ratio of 2.5:1.
2.4 Calculation of soil indexes
The saturated hydraulic conductivity of the soil (KS) (Campo et al., 2008), mean weight diameter (MWD) (Ortas and Lal, 2012), soil disintegration rate (SDR) (Guo et al., 2021), soil structure stability index (SSSI) (Nichols and Toro, 2011), soil organic carbon cementing agent index (SCAI) (Dong et al., 2022a), and K factor (Jiang et al., 2020; Li et al., 2012) were used to express the soil erodibility. These indexes were calculated using Eqs. (1)–(5) show below.
where Q is the outflow volume (mL), A is the soil column section (mm2), t is the time (min), h is the head difference (mm), and L is the height of the soil column (mm).
where wi is the mass of the ith level of aggregates or other soil material (g), mt is the sample mass, and di is the mean diameter of the ith level of aggregates or other soil material (mm).
where M1 and M2 are the weight of the soil before (t1) and after (t2) disintegration, respectively.
where SOMC is the content of soil organic matter (Kar et al., 2023), C= 0.583 × SOMC; Cl and Si represent the clay and silt contents (%), respectively; SN1 = ; K represents the soil loss rate per unit area under rainfall erosivity conditions for a specified soil on a standard plot (Jiang et al., 2020; Renard et al., 1997). A previous study indicates the rationality and validity of estimating K in the Zhangjiakou region using this model (H. Wang et al., 2020).
In order to further evaluate the soil nutrients and erodibility, comprehensive soil nutrient and erodibility indexes were calculated using Eqs. (6) and (7), respectively:
where Kni and Cni are the weight and soil nutrient index score respectively; Kei and Cei are the weight and soil erodibility index score, respectively; and n is the number of indexes.
The weight of each soil nutrient index and soil erodibility index was determined using a principal component analysis (PCA) (Pandey et al., 2021; Wang et al., 2018). The SHC, MWD, SSSI, SOC, TN, and TP scores were calculated using a “reverse S” function, which was calculated using Eq. (8).
The SDR and K factor scores were calculated using an “S” function, as shown in Eq. (9).
The comprehensive soil quality index (CSQI) is used to express the soil quality, which takes into account both soil nutrients and erodibility (De Laurentiis et al., 2019; Dong et al., 2022b). The CSQI was calculated as follows (Eq. 10):
where CSQI (> 0), CSNI (0−1), and CSEI (0−1) are the comprehensive soil quality, nutrient, and erodibility indexes, respectively.
2.5 Statistical analysis
SPSS v. 20 software was used for data processing and statistical analysis, and ArcGIS 10.4.1 and Origin 2021 were used for graphing. A one-way analysis of variance (ANOVA) was used to compare soil nutrient and erodibility indexes for different slope aspects and different land-use types. The effects of land use type, slope aspect, and their interaction on the soil nutrient and erodibility indexes were tested using a two-way ANOVA. Pearson's correlation coefficient was used to determine the correlation between soil nutrients, erodibility, and quality indexes and their influencing factors. The contributions of the understory vegetation and soil characteristics to the total variance in the soil nutrient and erodibility indicators were determined using a redundancy analysis (RDA) (Capblancq et al., 2018; Peres-Neto et al., 2006). A random forest algorithm based on R software was used to analyze the importance of impact factors for different slope aspects (Schonlau and Zou, 2020; Vincenzi et al., 2011). The importance index was determined as the average accuracy reduction. When the importance index is higher, it means that the corresponding factor holds more weight (Y. Chen et al., 2021; Hao et al., 2015).
3.1 Changes in the characteristics of understory vegetation with slope aspect
Slope aspect significantly influenced some of the characteristics of the understory vegetation such as the aboveground biomass (AGB) and belowground biomass (BGB). All measured characteristics of the understory vegetation on the western slope were lower than those on the slopes with other aspects. AGB and BGB was significantly lower for the western slope than the eastern slope (Fig. 2). AGB and BGB on the eastern slope were significantly (63.40 % and 78.40 %, respectively) higher than those on the western slope (Fig. 2d, e). The measured plant characteristics from the eastern and western slopes were not significantly different from those on the northern and southern slopes. There were significant differences among the four land-use types for all characteristics measured on the western slope (Table S1). The BH, R, and AGB of the understory vegetation were significantly higher for the woodland than for the other three land-use types (Fig. 2). Overall, shrubland had the highest litter biomass for each slope aspect, while degraded land on the western slope had the lowest.
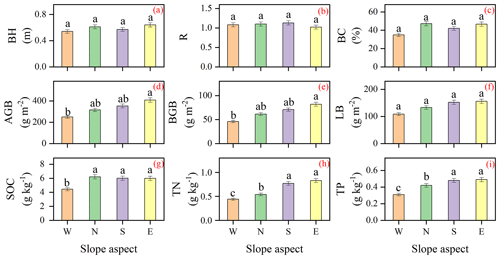
Figure 2Variations in understory vegetation characteristics and soil nutrients with slope aspect. BH, biomass height; R, richness; BC, biomass coverage; AGB, aboveground biomass; BGB, belowground biomass; LB, litter biomass; SOC, soil organic carbon; TN, total nitrogen; TP, total phosphorus; W, west; N, north; S, south; E, east. Different letters indicate significant differences among the different slope aspects at the p<0.05 level.
3.2 Changes in soil nutrients for different slope aspects
Slope aspect significantly affected soil nutrients. Soil organic carbon (SOC), total nitrogen (TN), and total phosphorus (TP) were significantly lower in soil collected from the western slope than in soil collected from the eastern slope (Fig. 2). The SOC of the eastern slope was 0.96–1.38 times greater than the SOCs of the other slopes (Fig. 2g). TN was highest on the eastern slope and was 0.39 and 0.28 g kg−1 greater on that slope than on the western and northern slopes, respectively (Fig. 2h). Similarly, the TP was significantly greater on the eastern slope than on the southern and eastern slopes by 59.60 % and 17.37 %, respectively (Fig. 2i). When all slope aspects were considered, the comprehensive soil nutrient index (CSNI) was significantly lower on the western slope than for the other three slope aspects. The highest CSNI was found for both the southern slope (0.81) and the eastern slope (0.86) (Fig. 3). For a given slope aspect, land use type also significantly influenced soil nutrients (Fig. S1). For example, on the western slope, the SOC of forested land was significantly higher than those of other restored land uses by 11.81 %–150.84 %, depending on the comparison. SOC, TN, and TP of degraded land were significantly lower than those of other land use types. CSNI was influenced by land use type, slope aspect, and their interactions (Table 1). Compared to degraded land, CSNI was significantly higher for all three land uses, with the greatest increase in CSNI seen for shrubland (0.75), followed by woodland and grassland (Fig. 4).
Table 1The two-way ANOVA results for soil nutrients and erodibility. SOC: soil organic carbon; TN: total nitrogen; TP: total phosphorus; CSNI: comprehensive soil nutrient index; SHC: saturated hydraulic conductivity; SDR: soil disintegration rate; MWD: mean weight diameter; K: soil erodibility factor; SSSI: soil structure stability index; SCAI: SOC cementing agent index; CSEI: comprehensive soil erodibility index; CSQI: comprehensive soil quality index.
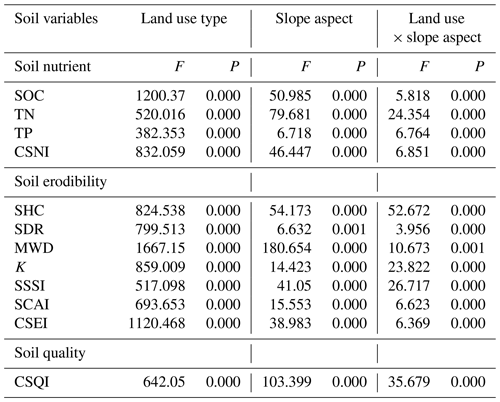
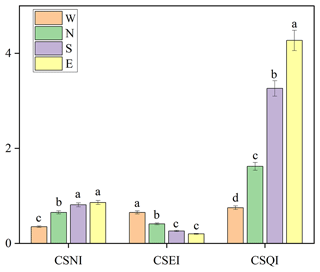
Figure 3Variation in the comprehensive soil nutrient, erodibility, and quality indexes with slope aspect. CSNI, comprehensive soil nutrient index; CSEI, comprehensive soil erodibility index; CSQI, comprehensive soil quality index. For each index, bars with different letters above them have significantly different values at the p<0.05 level.
3.3 Changes in soil erodibility under vegetation restoration
The effect of slope aspect on soil erodibility indicators was significant (Tables 1 and 2). Among the four slope aspects, the SHC of the soil collected from the eastern slope was the greatest; it was significantly greater than those of the western and northern slopes by 311.16 % and 187.10 %, respectively. MWD was highest on the eastern slope (3.65 mm), followed by the southern and northern slopes. MWD was significantly different for the four slopes. The SSSI of the western slope was the lowest (0.41 g kg−1), and it was significantly lower than the SSSIs for the other three slope aspects. In contrast, the highest SCAI was found on the western slope, and it was significantly higher than those for the other slope aspects by 46.10 %–59.70 %. When all slope aspects were considered, the southern (0.26) and eastern (0.20) slopes had the highest comprehensive soil erodibility index (CSEI) reduction capacities (Fig. 3). For any given slope aspect, land use type also greatly influenced soil erodibility indicators (Table 2). On the western slope, MWD was significantly increased by 0.67–1.59 mm. On the northern slope, the SHC of woodland was significantly higher than those of shrubland (by 117.67 %) and grassland (by 94.24 %). On the southern slope, the K of the grassland land-use type was significantly lower than those of woodland and shrubland. On the eastern slope, the soil disintegration rates of the three restored land uses were significantly different, with the highest SDR occurring in the woodlands. CSEI was influenced by land use type, slope aspect, and their interactions (Table 1). The CSEIs of all three restored land uses were significantly lower (by 63.01 %–64.70 %) compared to that of the degraded land (Fig. 4).
3.4 Changes in comprehensive soil quality index under vegetation restoration
When all slope aspects were considered, there were found to be significant differences in comprehensive soil quality index (CSQI), with the eastern slope (2.46) having the greatest capacity to increase the CSQI (Fig. 3). Compared to degraded land, the CSQIs of grassland, shrubland, and woodland were increased significantly by 2.51, 2.65, and 2.44, respectively (Fig. 4). CSQI was influenced by land use type, slope aspect, and their interactions (Table 1).
The differences in CSQI between vegetation types were compared to determine the optimal vegetation restoration types for different slope aspects. On the western slope, the grassland with Capillipedium parviflorum (WGCP) and the woodland with Pinus sylvestris and Astragalus melilotoides (WGCP) had relatively high CSQIs. They were significantly higher than the CSQIs of other vegetation types (Fig. 6a). Therefore, these two plant communities may be selected for restoration practices on the western slope. On the northern slope, the CSQI of the shrubland NSCK was significantly higher than those of the other vegetation types, with the second highest CSQI observed for the grassland NGBI. Thus, the combination of Caragana korshinskii and Capillipedium parviflorum (NSCK) could also be selected as taxa for use as restoration vegetation (Fig. 5b). On the southern slope, the CSQI of the grassland SGAM was significantly higher than those of the other vegetation types (Fig. 5c). SGAM was dominated by the herb Astragalus melilotoides, which had the highest CSQI. A. melilotoides could be selected to improve soil quality on the southern slope. On the eastern slope, the CSQI of the shrubland ESCK was higher than those for the other sites (Fig. 5d). ESCK was dominated by Caragana korshinskii and Lespedeza bicolor, which had the highest CSQI. Therefore, these species should be selected to improve soil quality on the eastern slope.
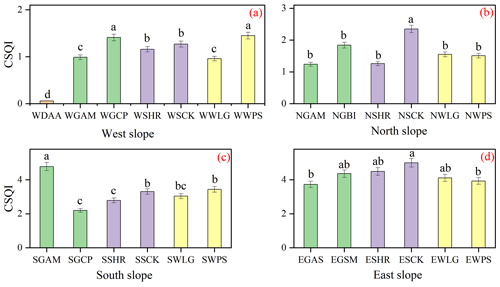
Figure 5Variation in the comprehensive soil quality index with vegetation type for different slope aspects. WDAA, Artemisia annua; WGAM, NGAM, and SGAM, Astragalus melilotoides; NGBI, Bothriochloa ischaemum; EGSM, Artemisia sacrorum, Astragalus melilotoides; WGCP, NGCP, and SGCP, Capillipedium parviflorum; WSHR, NSHR, SSHR, and ESHR, Hippophae rhamnoides; WSCK, NSCK, SSCK, and ESCK, Caragana korshinskii; WWLG, NSWG, SSWG, and ESWG, Larix gmelinii; WWPS, NWPS, SWPS, and EWPS, Pinus sylvestris. Different letters indicate significant differences among different seasons at p<0.05 level.
3.5 Key factors and their contributions for different slope aspects
The RDA followed by Monte Carlo permutation tests revealed that the variations in the nine measured soil quality indicators were significantly influenced by understory vegetation and soil characteristics for the four slope aspects (p<0.01, Fig. 6). On the western slope, 62.7 % of the total variance can be explained by understory vegetation and soil characteristics (Fig. 6a), with understory vegetation and soil characteristics explaining 43.11 % and 19.59 % of the total variance, respectively. For the northern slope, the understory vegetation and soil characteristics contributed 50.86 % of the total variance of soil quality (Fig. 6b), of which understory vegetation and soil characteristics accounted for 33.28 % and 17.58 % of the total variance, respectively. On the southern slope, 54.23 % of the total variance of soil quality could be explained by understory vegetation and soil characteristics, of which the combination of soil and roots contributed 44.56 % and 9.67 % of the total variance, respectively (Fig. 6c). However, on the eastern slope, the understory vegetation and soil characteristics contributed 74.56 % of the total variance of soil quality (Fig. 6d), of which understory vegetation and soil characteristics accounted for 56.81 % and 17.59 % of the total variance, respectively.
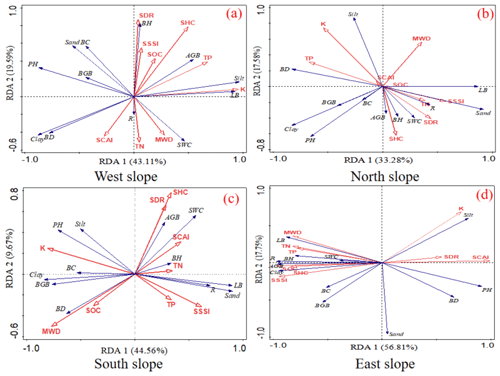
Figure 6Results of a redundancy analysis (RDA) of soil quality parameters and the characteristics of the vegetation and soil for the four slope aspects. BH: biome height; R: richness; BC: biome coverage; AGB: aboveground biomass; BGB: belowground biomass; LB: litter biomass; Sand: sand content; Silt: silt content; Clay: clay content; SWC: soil water content; SBD: soil bulk density; SOC: soil organic carbon; TN: total nitrogen; TP: total phosphorus; SHC, saturated hydraulic conductivity; SDR, soil disintegration rate; MWD, mean weight diameter; K, soil erodibility factor; SSSI, soil structure stability index; SCAI, SOC cementing agent index.
The random forest analysis highlighted the importance of 21 modeling factors when determining the restoration characteristics of understory vegetation and the physical and chemical characteristics of topsoil for different slope aspects. MWD, TP, saturated hydraulic conductivity (SHC), and soil disintegration rate (SDR) were the main factors that influenced understory vegetation and soil properties for different slope aspects. The mean accuracy reduction was calculated using the random forest method. Using this calculation, we obtained an MWD of 13.40, a TP of 13.30, an SHC of 12.60, and an SDR of 8.20 (Fig. S2).
4.1 Effects of slope aspect on understory vegetation characteristics
Although slope aspect, one of the most important topographic factors, may impact vegetation characteristics due to differences in sunlight, moisture, temperature, and soil, our results showed that most of the characteristics of understory vegetation showed no significant differences for different slope aspects. This may be due to the fact that the understory plants were shaded by the taller trees and shrubs (Niinemets, 2010). Aboveground biomass was greater on the eastern and southern slopes than on the northern and western slopes. Vegetation density was lowest on the western slope. These findings indicated that aboveground biomass is closely related to the number of sunshine hours, which affects the balance of heat and water (Z. Chen et al., 2021; Shi et al., 2021). This contributed to the low aboveground biomass of the western slope. Similarly, belowground biomass declined in the following order: eastern slope, southern slope, northern slope, and western slope. This may be due to the differences in aboveground biomass between the four slope aspects. Aboveground biomass impacts belowground biomass (Sun et al., 2022), and the belowground biomass was significantly lower on the western slope than on the eastern slope.
4.2 Effects of slope aspect on soil nutrients
Our results show that the conditions related to slope aspect have significant effects on single soil nutrient indicators and the comprehensive soil nutrient index (Figs. 2, 5). In the same area, soil nutrients can vary depending on the slope aspect (Li et al., 2021; Sharma et al., 2010). TN, TP, and the comprehensive soil nutrient index of surface soil were highest on the eastern and southern slopes, while the soil organic carbon content was highest on the northern slope. Plants need to absorb a large amount of fast-acting nitrogen and phosphorus during vegetative growth, and the nutrients required for plant growth are converted from organic matter in the soil. The presence of the lowest SOC, TN, TP, and comprehensive soil nutrient index on the western slope is due to the fact that it was located in the wind–water erosion zone of the northern agro-pastoral ecotone, and the topsoil on this slope has been lost due to long-term wind erosion.
The effect of slope aspect on soil pH was limited. This is because plant root systems and sediments were not abundant in the case of vegetation restoration 12 years (Bai et al., 2020). The organic acid content was low when combined with organic matter during decomposition and vegetation restoration; therefore, it was insufficient to lower the pH of the surface soil (Seddaiu et al., 2013).
4.3 Effects of slope aspect on soil erodibility
Our results show that slope aspect has a significant effect on single soil erodibility indexes as well as the comprehensive soil erodibility index. In general, soil erodibility decreases from the western slope to the eastern slope (Table 2), a pattern that may be related to the geographical location, altitude, temperature, and semi-arid climate of the region. Due to their special locations, the western and northern slopes are susceptible to year-round gales from the northwestern interior and Siberia, resulting in varying environmental conditions. However, the soil water content of the northern slope (shaded slope) is higher than that of the western slope, which may favor vegetation restoration on the northern slope (Liu et al., 2020); the western slope may be more vulnerable to erosion. Wind speed and soil moisture are key factors controlling the process of vegetation restoration (Hupet and Vanclooster, 2002; Meng et al., 2018), and these factors further influence soil erodibility (Sun et al., 2016).
Table 2Soil erodibility indicators for different land use types on slopes with different aspects (mean ± SD). SHC, saturated hydraulic conductivity; SDR, soil disintegration rate; MWD, mean weight diameter; K, soil erodibility factor; SSSI, soil structure stability index; SCAI, SOC cementing agent index. When comparing slope aspects, values with different capital letters are significantly different (p<0.05); when comparing land use types, values with different lowercase letters are significantly different (p<0.05).
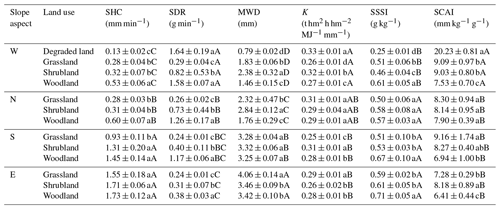
4.4 Relationship between soil nutrients and soil erodibility
The comprehensive soil nutrient index was significantly positively correlated with saturated hydraulic conductivity, mean weight diameter, and soil structure stability index (Fig. 7), while the comprehensive soil nutrient index was highly significantly negatively correlated with the comprehensive soil erodibility index. Many previous studies have reported similar results (Dong et al., 2022a; Zhu et al., 2018). In this study, higher saturated hydraulic conductivity, mean weight diameter, and soil structure stability index values and lower soil disintegration rate, K, and SOC cementing agent index values indicate a better soil structure and lower soil erodibility. These characteristics can significantly reduce runoff and sediment loss, which can result in soil nutrient accumulation (Pan and Shangguan, 2006; Sun et al., 2015; Zheng et al., 2021). Therefore, revegetation increases soil nutrients and reduces soil erodibility, which further change the vegetation and soil characteristics. In addition, these factors could reduce soil nutrient loss and further promote soil nutrient accumulation by reducing soil erodibility.
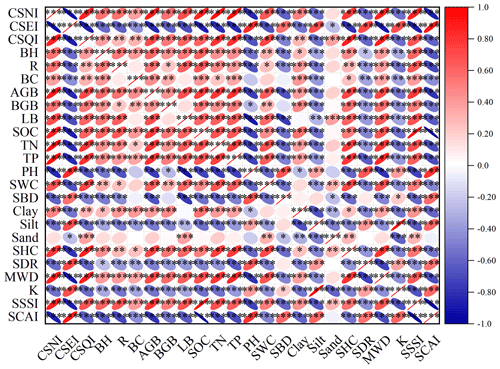
Figure 7Correlation analysis of CSNI, CSEI, and CSQI with vegetation and soil characteristics. Red indicates a positive correlation, blue indicates a negative correlation, and the color depth indicates the Pearson coefficient. * p<0.05, , p<0.01, and p<0.001, n= 84. CSNI, comprehensive soil nutrient index; CSEI, comprehensive soil erodibility index; CSQI, comprehensive soil quality index.
The comprehensive soil erodibility index was highly significantly negatively correlated with SOC, TN, and TP (Fig. 7). Previous studies have shown that soil organic matter and SOC are closely related to soil erodibility (Wang et al., 2019b). SOC acts as a cement for soil aggregation, which improves soil structural stability through the formation of aggregates, thus reducing soil erodibility. Soil nitrogen indirectly affects soil erodibility by promoting plant growth and development, increasing the accumulation of SOC in plants. In addition, nitrogen enrichment increased soil macroparticles and mean weight diameter, which directly affected soil erodibility. Similar to nitrogen, phosphorus is one of the essential elements for plant growth and development, and the phosphorus content of soil determines the development of soil microorganisms and root systems, which will further influence the input of soil organic carbon and the formation of soil aggregates.
4.5 Effects of slope aspect on the key factors impacting soil and vegetation
The results derived from the random forest method showed that mean weight diameter and TP were the main influencing factors. The main adhesion agents for the formation of aggregates included clay content, SOC, and cementation. The mean weight diameter was significantly and positively correlated with soil organic carbon and clay content. The magnitude of the mean weight diameter affects soil structural stability and root establishment, which varies with slope aspect due to environmental factors. Soil phosphorus is an important element necessary for plant growth and development, and rapid growth requires more soil phosphorus, so there were some differences between land use types on slopes with different aspects. The difference in TP between slope aspects affected the amount of inorganic phosphorus available for uptake by plants, and the lower phosphorus content limited plant growth. Upon analyzing the main factors influencing the surface soil quality for different slope aspects, we can conclude that the timely application of phosphorus fertilizer in vegetation restoration projects could help accelerate the process of afforestation.
4.6 Optimal land use type and plant species based on slope aspect
Our study has shown that vegetation restoration can be an effective measure to improve soil nutrients and reduce soil erodibility. Moreover, the optimal restored land-use types and plant species to use to improve soil quality differed significantly depending on the slope aspect. Therefore, the selection of the land use and its corresponding vegetation types should be carefully considered according to the differences in water, heat, wind, and sand for different slope aspects. Our findings both agree with and differ from previous studies (Colgan et al., 2010; Dong et al., 2022a; H. Wang et al., 2021). Studies that found contrasting results are likely due to the different environmental conditions (in terms of, e.g., climate, rainfall, topographic conditions, seed bank, soil texture) of the different slope aspects. It is noteworthy that herbaceous vegetation on the western slope is prone to severe shallow nutrient loss and soil erosion because of strong wind conditions and sandy soil (Guo et al., 2020). Therefore, the use of herbaceous vegetation as the primary restoration vegetation species should be carefully considered. Fortunately, our proposal (to use Caragana korshinskii and Lespedeza bicolor) satisfies this requirement. In addition, wind also contributes to soil erosion in this region; however, limited research has been conducted on wind erosion and combined erosion by wind and water. Future studies should be conducted on combined erosion by wind and water to better characterize soil erosion.
We found that some understory vegetation characteristics and soil properties varied significantly with slope aspect. Soil nutrients and erodibility, reflected by the soil organic carbon, total nitrogen, total phosphorus, saturated hydraulic conductivity, soil disintegration rate, mean weight diameter, soil structure stability index, soil erodibility factor, and soil organic carbon cementing agent index, were also influenced by slope aspect and land use. Furthermore, the comprehensive soil nutrient, erodibility, and quality indexes also varied significantly with slope aspect, land use, and the predominant plant species. Slope aspect strongly modified the relationship between the comprehensive soil nutrient, erodibility, and quality indexes as well as understory vegetation characteristics and soil properties. Our study found that Caragana korshinskii and Lespedeza bicolor were the best taxa to include on slopes of any aspect to improve soil nutrients and prevent soil erosion. This study provides insight into the rational planning of vegetation restoration measures for slopes with particular aspects in the northern agro-pastoral ecotone in semi-arid areas. Future work will focus on land degradation associated with soil erosion from water and storms in the region.
Data will be made available on request.
The supplement related to this article is available online at: https://doi.org/10.5194/soil-10-61-2024-supplement.
YWu: writing – original draft. GJ: project administration, funding acquisition, writing – review and editing. XY: project administration, funding acquisition, writing – review and editing. HR: methodology and formal analysis. XP: investigation. YusoW, YushW, and XW: investigation.
The contact author has declared that none of the authors has any competing interests.
Publisher’s note: Copernicus Publications remains neutral with regard to jurisdictional claims made in the text, published maps, institutional affiliations, or any other geographical representation in this paper. While Copernicus Publications makes every effort to include appropriate place names, the final responsibility lies with the authors.
This research has been supported by the National Key Research and Development Program of China (grant no. 2022YFF1302502-03) (China) and the National Natural Science Foundation of China (grant no. 42230714).
This paper was edited by Hu Zhou and reviewed by two anonymous referees.
Akiyama, T. and Kawamura, K.: Grassland degradation in China: Methods of monitoring, management and restoration, Grassl. Sci., 53, 1–17, https://doi.org/10.1111/j.1744-697X.2007.00073.x, 2007.
Bai, Y., Zha, X., and Chen, S.: Effects of the vegetation restoration years on soil microbial community composition and biomass in degraded lands in Changting County, China, J. Forestry Res., 31, 1295–1308, https://doi.org/10.1007/s11676-019-00879-z, 2020.
Bangroo, S. A., Najar, G. R., and Rasool, A.: Effect of altitude and aspect on soil organic carbon and nitrogen stocks in the Himalayan Mawer Forest Range, Catena, 158, 63–68, https://doi.org/10.1016/j.catena.2017.06.017, 2017.
Barua, G. and Alam, W.: An analytical solution for predicting transient seepage into ditch drains from a ponded field, Adv. Water Resour., 52, 78–92, https://doi.org/10.1016/j.advwatres.2012.09.002, 2013.
Batista, P. V. G., Evans, D. L., Cândido, B. M., and Fiener, P.: Does soil thinning change soil erodibility? An exploration of long-term erosion feedback systems, SOIL, 9, 71–88, https://doi.org/10.5194/soil-9-71-2023, 2023.
Borchard, N., Adolphs, T., Beulshausen, F., Ladd, B., Gießelmann, U. C., Hegenberg, D., Möseler, B. M., and Amelung, W.: Carbon accrual rates, vegetation and nutrient dynamics in a regularly burned coppice woodland in Germany, GCB Bioenergy, 9, 1140–1150, https://doi.org/10.1111/gcbb.12408, 2017.
Bryan, R. B., Govers, G., and Poesen, J.: The concept of soil erodibility and some problems of assessment and application, Catena, 16, 393–412, https://doi.org/10.1016/0341-8162(89)90023-4, 1989.
Campo, J., Gimeno-García, E., Andreu, V., González-Pelayo, O., and Rubio, J. L.: Aggregation of under canopy and bare soils in a Mediterranean environment affected by different fire intensities, Catena, 74, 212–218, https://doi.org/10.1016/j.catena.2008.05.002, 2008.
Capblancq, T., Luu, K., Blum, M. G. B., and Bazin, E.: Evaluation of redundancy analysis to identify signatures of local adaptation, Mol. Ecol. Resour., 18, 1223–1233, https://doi.org/10.1111/1755-0998.12906, 2018.
Chandler, K. R. and Chappell, N. A.: Influence of individual oak (Quercus robur) trees on saturated hydraulic conductivity, Forest Ecol. Manag., 256, 1222–1229, https://doi.org/10.1016/j.foreco.2008.06.033, 2008.
Chang, X., Sun, L., Yu, X., Liu, Z., Jia, G., Wang, Y., and Zhu, X.: Windbreak efficiency in controlling wind erosion and particulate matter concentrations from farmlands, Agr. Ecosyst. Environ., 308, 107269, https://doi.org/10.1016/j.agee.2020.107269, 2021.
Che, C., Xiao, S., Ding, A., Peng, X., and Su, J.: Growth response of plantations Hippophae rhamnoides Linn. on different slope aspects and natural Caragana opulens Kom. to climate and implications for plantations management, Ecol. Indic., 138, 108833, https://doi.org/10.1016/j.ecolind.2022.108833, 2022.
Chen, Y., Zheng, W., Li, W., and Huang, Y.: Large group activity security risk assessment and risk early warning based on random forest algorithm, Pattern Recogn. Lett., 144, 1–5, https://doi.org/10.1016/j.patrec.2021.01.008, 2021.
Chen, Z., Wang, G., Pan, Y., Yang, X., and Shen, Y.: Water use patterns differed notably with season and slope aspect for Caragana korshinskii on the Loess Plateau of China, Catena, 198, 105028, https://doi.org/10.1016/j.catena.2020.105028, 2021.
Colgan, R., Atkinson, C. J., Paul, M., Hassan, S., Drake, P. M. W., Sexton, A. L., Santa-Cruz, S., James, D., Hamp, K., Gutteridge, C., and Ma, J. K.-C.: Optimisation of contained Nicotiana tabacum cultivation for the production of recombinant protein pharmaceuticals, Transgenic Res., 19, 241–256, https://doi.org/10.1007/s11248-009-9303-y, 2010.
Cornfield, A. H.: The phosphate status of garden soils in relation to soil pH, Plant Soil, 5, 243–245, https://doi.org/10.1007/BF01395899, 1954.
De Laurentiis, V., Secchi, M., Bos, U., Horn, R., Laurent, A., and Sala, S.: Soil quality index: Exploring options for a comprehensive assessment of land use impacts in LCA, J. Clean. Prod., 215, 63–74, https://doi.org/10.1016/j.jclepro.2018.12.238, 2019.
de Vente, J., Poesen, J., Verstraeten, G., Van Rompaey, A., and Govers, G.: Spatially distributed modelling of soil erosion and sediment yield at regional scales in Spain, Global Planet. Change, 60, 393–415, https://doi.org/10.1016/j.gloplacha.2007.05.002, 2008.
de Vente, J., Poesen, J., Verstraeten, G., Govers, G., Vanmaercke, M., Van Rompaey, A., Arabkhedri, M., and Boix-Fayos, C.: Predicting soil erosion and sediment yield at regional scales: Where do we stand?, Earth-Sci. Rev., 127, 16–29, https://doi.org/10.1016/j.earscirev.2013.08.014, 2013.
Dong, L., Li, J., Zhang, Y., Bing, M., Liu, Y., Wu, J., Hai, X., Li, A., Wang, K., Wu, P., Shangguan, Z., and Deng, L.: Effects of vegetation restoration types on soil nutrients and soil erodibility regulated by slope positions on the Loess Plateau, J. Environ. Manage., 302, 113985, https://doi.org/10.1016/j.jenvman.2021.113985, 2022a.
Dong, L., Li, J., Zhang, Y., Bing, M., Liu, Y., Wu, J., Hai, X., Li, A., Wang, K., Wu, P., Shangguan, Z., and Deng, L.: Effects of vegetation restoration types on soil nutrients and soil erodibility regulated by slope positions on the Loess Plateau, J. Environ. Manage., 302, 113985, https://doi.org/10.1016/j.jenvman.2021.113985, 2022b.
Dou, P., Miao, Z., Wang, J., Huang, J., Gao, Q., Wang, K., and Wang, K.: The key to temperate savanna restoration is to increase plant species richness reasonably, Front. Environ. Sci., 11, 1112779, https://doi.org/10.3389/fenvs.2023.1112779, 2023.
Guo, M., Chen, Z., Wang, W., Wang, T., Wang, W., and Cui, Z.: Revegetation induced change in soil erodibility as influenced by slope situation on the Loess Plateau, Sci. Total Environ., 772, 145540, https://doi.org/10.1016/j.scitotenv.2021.145540, 2021.
Guo, Q., Cheng, C., Jiang, H., Liu, B., and Wang, Y.: Comparative rates of wind and water erosion on typical farmland at the northern end of the Loess Plateau, China, Geoderma, 352, 104–115, https://doi.org/10.1016/j.geoderma.2019.05.011, 2019.
Guo, W.-Z., Chen, Z.-X., Wang, W.-L., Gao, W.-W., Guo, M.-M., Kang, H.-L., Li, P.-F., Wang, W.-X., and Zhao, M.: Telling a different story: The promote role of vegetation in the initiation of shallow landslides during rainfall on the Chinese Loess Plateau, Geomorphology, 350, 106879, https://doi.org/10.1016/j.geomorph.2019.106879, 2020.
Hao, P., Zhan, Y., Wang, L., Niu, Z., and Shakir, M.: Feature selection of time series MODIS data for early crop classification using random forest: A case study in Kansas, USA, Remote Sens., 7, 5347–5369, https://doi.org/10.3390/rs70505347, 2015.
Huang, C., Zeng, Y., Wang, L., and Wang, S.: Responses of soil nutrients to vegetation restoration in China, Reg. Environ. Change, 20, 82, https://doi.org/10.1007/s10113-020-01679-6, 2020.
Hupet, F. and Vanclooster, M.: Intraseasonal dynamics of soil moisture variability within a small agricultural maize cropped field, J. Hydrol., 261, 86–101, https://doi.org/10.1016/S0022-1694(02)00016-1, 2002.
Jiang, Q., Zhou, P., Liao, C., Liu, Y., and Liu, F.: Spatial pattern of soil erodibility factor (K) as affected by ecological restoration in a typical degraded watershed of central China, Sci. Total Environ., 749, 141609, https://doi.org/10.1016/j.scitotenv.2020.141609, 2020.
Kar, S. K., Singh, R. M., Patra, S., Sankar, M., Kumar, S., and Singh, A.: Implication of land use shifting on land degradation and restoration potential of conservation agriculture in India's North-West Himalayan region, Geoderma Regional, 32, e00616, https://doi.org/10.1016/j.geodrs.2023.e00616, 2023.
Kisand, A.: Distribution of sediment phosphorus fractions in hypertrophic strongly stratified Lake Verevi, in: Lake Verevi, Estonia – A Highly Stratified Hypertrophic Lake, Vol. 182, edited by: Ott, I. and Kõiv, T., Springer-Verlag, Berlin/Heidelberg, 33–39, https://doi.org/10.1007/1-4020-4363-5_3, 2005.
Lardy, J. M., DeSutter, T. M., Daigh, A. L. M., Meehan, M. A., and Staricka, J. A.: Effects of soil bulk density and water content on penetration resistance, Agr. Environ. Lett., 7, 2, https://doi.org/10.1002/ael2.20096, 2022.
Li, H., Zhu, H., Qiu, L., Wei, X., Liu, B., and Shao, M.: Response of soil OC, N and P to land-use change and erosion in the black soil region of the Northeast China, Agr. Environ. Lett., 302, 107081, https://doi.org/10.1016/j.agee.2020.107081, 2020.
Li, R., Zhang, W., Yang, S., Zhu, M., Kan, S., Chen, J., Ai, X., and Ai, Y.: Topographic aspect affects the vegetation restoration and artificial soil quality of rock-cut slopes restored by external-soil spray seeding, Sci. Rep., 8, 12109, https://doi.org/10.1038/s41598-018-30651-y, 2018.
Li, T., Zeng, J., He, B., and Chen, Z.: Changes in Soil C, N, and P Concentrations and Stoichiometry in Karst Trough Valley Area under Ecological Restoration: The Role of Slope Aspect, Land Use, and Soil Depth, Forests, 12, 144, https://doi.org/10.3390/f12020144, 2021.
Li, W., Yan, M., Qingfeng, Z., and Zhikaun, J.: Effects of Vegetation Restoration on Soil Physical Properties in the Wind-Water Erosion Region of the Northern Loess Plateau of China, Clean Soil Air Water, 40, 7–15, https://doi.org/10.1002/clen.201100367, 2012.
Li, Z., Liu, C., Dong, Y., Chang, X., Nie, X., Liu, L., Xiao, H., Lu, Y., and Zeng, G.: Response of soil organic carbon and nitrogen stocks to soil erosion and land use types in the Loess hilly–gully region of China, Soil and Tillage Research, 166, 1–9, https://doi.org/10.1016/j.still.2016.10.004, 2017.
Liu, L., Gudmundsson, L., Hauser, M., Qin, D., Li, S., and Seneviratne, S. I.: Soil moisture dominates dryness stress on ecosystem production globally, Nat. Commun., 11, 4892, https://doi.org/10.1038/s41467-020-18631-1, 2020.
Masciandaro, G. and Ceccanti, B.: Assessing soil quality in different agro-ecosystems through biochemical and chemico-structural properties of humic substances, Soil and Tillage Research, 51, 129–137, https://doi.org/10.1016/S0167-1987(99)00056-2, 1999.
Meng, Z., Dang, X., Gao, Y., Ren, X., Ding, Y., and Wang, M.: Interactive effects of wind speed, vegetation coverage and soil moisture in controlling wind erosion in a temperate desert steppe, Inner Mongolia of China, J. Arid Land, 10, 534–547, https://doi.org/10.1007/s40333-018-0059-1, 2018.
Moreira, W. H., Tormena, C. A., de Lima, R. P., Anghinoni, G., and Imhoff, S.: The influence of sowing furrow opening and wetting and drying cycles on soil physical quality under no-tillage in Southern Brazil, Soil and Tillage Research, 204, 104711, https://doi.org/10.1016/j.still.2020.104711, 2020.
Nabiollahi, K., Golmohamadi, F., Taghizadeh-Mehrjardi, R., Kerry, R., and Davari, M.: Assessing the effects of slope gradient and land use change on soil quality degradation through digital mapping of soil quality indices and soil loss rate, Geoderma, 318, 16–28, https://doi.org/10.1016/j.geoderma.2017.12.024, 2018.
Nichols, K. A. and Toro, M.: A whole soil stability index (WSSI) for evaluating soil aggregation, Soil and Tillage Research, 111, 99–104, https://doi.org/10.1016/j.still.2010.08.014, 2011.
Niinemets, Ü.: A review of light interception in plant stands from leaf to canopy in different plant functional types and in species with varying shade tolerance, Ecol. Res., 25, 693–714, https://doi.org/10.1007/s11284-010-0712-4, 2010.
Ortas, I. and Lal, R.: Long-Term Phosphorus Application Impacts on Aggregate-Associated Carbon and Nitrogen Sequestration in a Vertisol in the Mediterranean Turkey, Soil Sci., 177, 241–250, https://doi.org/10.1097/SS.0b013e318245d11c, 2012.
Pan, C. and Shangguan, Z.: Runoff hydraulic characteristics and sediment generation in sloped grassplots under simulated rainfall conditions, J. Hydrol., 331, 178–185, https://doi.org/10.1016/j.jhydrol.2006.05.011, 2006.
Pandey, S., Kumar, P., Zlatic, M., Nautiyal, R., and Panwar, V. P.: Recent advances in assessment of soil erosion vulnerability in a watershed, International Soil and Water Conservation Research, 9, 305–318, https://doi.org/10.1016/j.iswcr.2021.03.001, 2021.
Peres-Neto, P. R., Legendre, P., Dray, S., and Borcard, D.: Variation partitioning of species data matrices: estimation and comparison of fractions, Ecology, 87, 2614–2625, https://doi.org/10.1890/0012-9658(2006)87[2614:VPOSDM]2.0.CO;2, 2006.
Proulx, M. and Mazumder, A.: Reversal of grazing impact on plant species richness in nutrient-poor vs. nutrient-rich ecosystems, Ecology, 79, 2581–2592, https://doi.org/10.1890/0012-9658(1998)079[2581:ROGIOP]2.0.CO;2, 1998.
Qin, Y., Feng, Q., Holden, N. M., and Cao, J.: Variation in soil organic carbon by slope aspect in the middle of the Qilian Mountains in the upper Heihe River Basin, China, Catena, 147, 308–314, https://doi.org/10.1016/j.catena.2016.07.025, 2016.
Renard, K. G., Foster, G. R., Weesies, G. A., McCool, D. K., and Yoder, D. C. (Eds.): Predicting soil erosion by water: a guide to conservation planning with the revised universal soil loss equation (RUSLE), Washington, DC, 384 pp., 1997.
Richard, H. L. and Donald, L. S.: Methods of soil analysis. Part 3, Chemical Methods, https://doi.org/10.2136/sssabookser5.3, 1996.
Schad, P.: World Reference Base for Soil Resources, in: Reference Module in Earth Systems and Environmental Sciences, Elsevier, https://doi.org/10.1016/B978-0-12-409548-9.10496-8, 2017.
Schloter, M., Dilly, O., and Munch, J. C.: Indicators for evaluating soil quality, Agr. Ecosyst. Environ., 98, 255–262, https://doi.org/10.1016/S0167-8809(03)00085-9, 2003.
Schmiedel, U., Kruspe, M., Kayser, L., and Oettlé, N.: The ecological and financial impact of soil erosion and its control – a case study from the semiarid northern cape province, south africa, Land Degrad. Dev., 28, 74–82, https://doi.org/10.1002/ldr.2513, 2017.
Schonlau, M. and Zou, R. Y.: The random forest algorithm for statistical learning, The Stata Journal, 20, 3–29, https://doi.org/10.1177/1536867X20909688, 2020.
Seddaiu, G., Porcu, G., Ledda, L., Roggero, P. P., Agnelli, A., and Corti, G.: Soil organic matter content and composition as influenced by soil management in a semi-arid Mediterranean agro-silvo-pastoral system, Agr. Ecosyst. Environ., 167, 1–11, https://doi.org/10.1016/j.agee.2013.01.002, 2013.
Sharma, C. M., Baduni, N. P., Gairola, S., Ghildiyal, S. K., and Suyal, S.: Effects of slope aspects on forest compositions, community structures and soil properties in natural temperate forests of Garhwal Himalaya, J. Forestry Res., 21, 331–337, https://doi.org/10.1007/s11676-010-0079-y, 2010.
Shi, X., Du, C., Guo, X., and Shi, W.: Heterogeneity of water-retention capacity of forest and its influencing factors based on meta-analysis in the Beijing-Tianjin-Hebei region, J. Geogr. Sci., 31, 69–90, https://doi.org/10.1007/s11442-021-1833-0, 2021.
Singh, G. and Panda, R. K.: Grid-cell based assessment of soil erosion potential for identification of critical erosion prone areas using USLE, GIS and remote sensing: A case study in the Kapgari watershed, India, International Soil and Water Conservation Research, 5, 202–211, https://doi.org/10.1016/j.iswcr.2017.05.006, 2017.
Singh, J. S. and Gupta, V. K.: Soil microbial biomass: A key soil driver in management of ecosystem functioning, Sci. Total Environ., 634, 497–500, https://doi.org/10.1016/j.scitotenv.2018.03.373, 2018.
Singh, K. and Pollard, A. G.: Relationship between soil structure, soil cultivation, nitrogen uptake and crop growth. III. – Effects of cultivation on the porosity of soil and its compactness and on crop development and yields, J. Sci. Food Agr., 9, 454–462, https://doi.org/10.1002/jsfa.2740090712, 1958.
Sun, J., YU, X., Fan, D., Liang, H., Chang, Y., and Li, H.: Impact of vegetation cover on surface runoff hydraulic characteristics with simulated rainfall, Acta Ecologica Sinica, 35, 8, https://doi.org/10.5846/stxb201310302620, 2015.
Sun, L., Zhang, G., Luan, L., and Liu, F.: Temporal variation in soil resistance to flowing water erosion for soil incorporated with plant litters in the Loess Plateau of China, Catena, 145, 239–245, https://doi.org/10.1016/j.catena.2016.06.016, 2016.
Sun, Y., Wang, Y., Yan, Z., He, L., Ma, S., Feng, Y., Su, H., Chen, G., Feng, Y., Ji, C., Shen, H., and Fang, J.: Above- and belowground biomass allocation and its regulation by plant density in six common grassland species in China, J. Plant Res., 135, 41–53, https://doi.org/10.1007/s10265-021-01353-w, 2022.
Tamene, G. M., Adiss, H. K., and Alemu, M. Y.: Effect of Slope Aspect and Land Use Types on Selected Soil Physicochemical Properties in North Western Ethiopian Highlands, Applied and Environmental Soil Science, 2020, 1–8, https://doi.org/10.1155/2020/8463259, 2020.
Vincenzi, S., Zucchetta, M., Franzoi, P., Pellizzato, M., Pranovi, F., De Leo, G. A., and Torricelli, P.: Application of a Random Forest algorithm to predict spatial distribution of the potential yield of Ruditapes philippinarum in the Venice lagoon, Italy, Ecol. Model., 222, 1471–1478, https://doi.org/10.1016/j.ecolmodel.2011.02.007, 2011.
Wang, B., Zhang, G.-H., Shi, Y.-Y., and Zhang, X. C.: Soil detachment by overland flow under different vegetation restoration models in the Loess Plateau of China, Catena, 116, 51–59, https://doi.org/10.1016/j.catena.2013.12.010, 2014.
Wang, H., Zhang, G., Li, N., Zhang, B., and Yang, H.: Soil erodibility influenced by natural restoration time of abandoned farmland on the Loess Plateau of China, Geoderma, 325, 18–27, https://doi.org/10.1016/j.geoderma.2018.03.037, 2018.
Wang, H., Zhang, G., Li, N., Zhang, B., and Yang, H.: Soil erodibility as impacted by vegetation restoration strategies on the Loess Plateau of China: Effect of vegetation restoration on soil erodibility, Earth Surf. Proc. Land., 44, 796–807, https://doi.org/10.1002/esp.4531, 2019a.
Wang, H., Zhang, G., Li, N., Zhang, B., and Yang, H.: Variation in soil erodibility under five typical land uses in a small watershed on the Loess Plateau, China, Catena, 174, 24–35, https://doi.org/10.1016/j.catena.2018.11.003, 2019b.
Wang, H., Sun, B., Yu, X., Xin, Z., and Jia, G.: The driver-pattern-effect connection of vegetation dynamics in the transition area between semi-arid and semi-humid northern China, Catena, 194, 104713, https://doi.org/10.1016/j.catena.2020.104713, 2020.
Wang, H., Wang, J., and Zhang, G.: Impact of landscape positions on soil erodibility indices in typical vegetation-restored slope-gully systems on the Loess Plateau of China, Catena, 201, 105235, https://doi.org/10.1016/j.catena.2021.105235, 2021.
Wang, S., Zhang, B., Xie, G., Zhai, X., and Sun, H.: Vegetation cover changes and sand-fixing service responses in the Beijing–Tianjin sandstorm source control project area, Environmental Development, 34, 100455, https://doi.org/10.1016/j.envdev.2019.08.002, 2020.
Wang, S., Zhang, B., Wang, S., and Xie, G.: Dynamic changes in water conservation in the Beijing–Tianjin Sandstorm Source Control Project Area: A case study of Xilin Gol League in China, J. Clean. Prod., 293, 126054, https://doi.org/10.1016/j.jclepro.2021.126054, 2021.
Wang, Z.-J., Jiao, J.-Y., Su, Y., and Chen, Y.: The efficiency of large-scale afforestation with fish-scale pits for revegetation and soil erosion control in the steppe zone on the hill-gully Loess Plateau, Catena, 115, 159–167, https://doi.org/10.1016/j.catena.2013.11.012, 2014.
Wen, H., Ni, S., Wang, J., and Cai, C.: Changes of soil quality induced by different vegetation restoration in the collapsing gully erosion areas of southern China, International Soil and Water Conservation Research, 9, 195–206, https://doi.org/10.1016/j.iswcr.2020.09.006, 2021.
Wischmeier, W. H. and Smith, D. D.: Predicting rainfall erosion losses. A guide to conservation planning, in: Agriculture Handbook No. 537, USDA-SEA, US. Govt. Printing Office, Washington, DC, 58 pp., 1978.
Wu, Y., Yu, X., and Jia, G.: Seasonal Variation of Soil Erodibility Under Vegetation Restoration in the Agro-pastoral Ecotone of Northern China, J. Soil Sci. Plant Nut., 23, 2331–2343, https://doi.org/10.1007/s42729-023-01183-w, 2023.
Yang, X., Shao, M., Li, T., Zhang, Q., Gan, M., Chen, M., and Bai, X.: Distribution of soil nutrients under typical artificial vegetation in the desert–loess transition zone, Catena, 200, 105165, https://doi.org/10.1016/j.catena.2021.105165, 2021.
Yimer, F., Ledin, S., and Abdelkadir, A.: Soil organic carbon and total nitrogen stocks as affected by topographic aspect and vegetation in the Bale Mountains, Ethiopia, Geoderma, 135, 335–344, https://doi.org/10.1016/j.geoderma.2006.01.005, 2006.
Zeng, X., Zhang, W., Cao, J., Liu, X., Shen, H., and Zhao, X.: Changes in soil organic carbon, nitrogen, phosphorus, and bulk density after afforestation of the “Beijing–Tianjin Sandstorm Source Control” program in China, Catena, 118, 186–194, https://doi.org/10.1016/j.catena.2014.01.005, 2014.
Zhang, B., Zhang, G., Zhu, P., and Yang, H.: Temporal variations in soil erodibility indicators of vegetation-restored steep gully slopes on the Loess Plateau of China, Agr. Ecosyst. Environ., 286, 106661, https://doi.org/10.1016/j.agee.2019.106661, 2019.
Zhang, J., Chen, H., Fu, Z., and Wang, K.: Effects of vegetation restoration on soil properties along an elevation gradient in the karst region of southwest China, Agr. Ecosyst. Environ., 320, 107572, https://doi.org/10.1016/j.agee.2021.107572, 2021.
Zhang, L., Cao, W., and Fan, J.: Soil organic carbon dynamics in Xilingol grassland of northern China induced by the Beijing-Tianjin Sand Source Control Program, Front. Earth Sci., 11, 407–415, https://doi.org/10.1007/s11707-016-0589-9, 2017.
Zhang, X., Hu, M., Guo, X., Yang, H., Zhang, Z., and Zhang, K.: Effects of topographic factors on runoff and soil loss in Southwest China, Catena, 160, 394–402, https://doi.org/10.1016/j.catena.2017.10.013, 2018.
Zhang, X., Adamowski, J. F., Liu, C., Zhou, J., Zhu, G., Dong, X., Cao, J., and Feng, Q.: Which slope aspect and gradient provides the best afforestation-driven soil carbon sequestration on the China's Loess Plateau?, Ecol. Eng., 147, 105782, https://doi.org/10.1016/j.ecoleng.2020.105782, 2020.
Zheng, J. Y., Zhao, J. S., Shi, Z. H., and Wang, L.: Soil aggregates are key factors that regulate erosion-related carbon loss in citrus orchards of southern China: Bare land vs. grass-covered land, Agr. Ecosyst. Environ., 309, 107254, https://doi.org/10.1016/j.agee.2020.107254, 2021.
Zhu, G., Deng, L., and Shangguan, Z.: Effects of soil aggregate stability on soil N following land use changes under erodible environment, Agr. Ecosyst. Environ., 262, 18–28, https://doi.org/10.1016/j.agee.2018.04.012, 2018.
Zhu, M., Yang, S., Ai, S., Ai, X., Jiang, X., Chen, J., Li, R., and Ai, Y.: Artificial soil nutrient, aggregate stability and soil quality index of restored cut slopes along altitude gradient in southwest China, Chemosphere, 246, 125687, https://doi.org/10.1016/j.chemosphere.2019.125687, 2020.