the Creative Commons Attribution 4.0 License.
the Creative Commons Attribution 4.0 License.
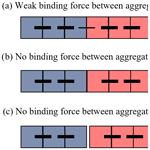
Perspectives on the misconception of levitating soil aggregates
John Koestel
Alice Johannes
Olivier Heller
Sebastian Doetterl
Thomas Keller
Soil aggregation is an important process in nearly all soils across the globe. Aggregates develop over time through a series of abiotic and biotic processes and interactions, including plant growth and decay, microbial activity, plant and microbial exudation, bioturbation, and physicochemical stabilization processes, and are greatly influenced by soil management practices. Together, and through feedback with organic matter and primary soil particles, these processes form dynamic soil aggregates and pore spaces, which jointly constitute a soil's structure and contribute to overall soil functioning. Nevertheless, the concept of soil aggregates is hotly debated, leading to confusion about their function or relevancy to soil processes. We argue here that the opposition to the concept of soil aggregation likely stems from the fact that the methods for the characterization of soil aggregates have largely been developed in the context of arable soils, where tillage promotes the formation of distinct soil aggregates that are easily visible in the topsoil. We propose that the widespread use of conceptual figures showing detached and isolated aggregates can be misleading and has contributed to the skepticism towards soil aggregates. However, the fact that we do not always see discrete aggregates within soils in situ does not mean that aggregates do not exist or are not relevant to the study of soil processes. Given that, by definition, soil aggregates consist of any group of soil particles that cohere more strongly to each other than neighboring particles, aggregates may, but do not necessarily need to be, bordered by pore space. Here, we illustrate how aggregates can form and dissipate within the context of undisturbed, intact soils, highlighting the point that aggregates do not necessarily need to have a discrete physical boundary and can exist seamlessly embedded in the soil. We hope that our contribution helps the debate on soil aggregates and supports the foundation of a shared understanding on the characterization and function of soil structure.
- Article
(2056 KB) - Full-text XML
- BibTeX
- EndNote
Soil structure is defined as “the spatial heterogeneity of the different components or properties of soil” (Dexter, 1988). In particular, the organization of these particles into solids (including organic material and stones), aggregates, and pore networks largely determines the capacity of soils to retain and transmit water, oxygen, and various other organic and inorganic substances through the soil profile (Bronick and Lal, 2005; Rabot et al., 2018). This structure not only provides habitat for soil organisms – and is in turn influenced by their activities – but the interaction between the physicochemical soil environment and its biological communities drives numerous environmental processes including root growth and plant development, nutrient cycling and carbon sequestration, water infiltration and purification, and protection against erosion (Lal, 1991; Sullivan et al., 2021). Together, these functions play a vital role in the provision of soil ecosystem services, thus further highlighting the importance of soils with respect to directly contributing to a multitude of sustainability goals (Lehmann et al., 2020; Lal et al., 2021).
Although the importance of soil structure as a foundation for sustaining key soil functions is increasingly recognized, no unified technique or single metric exists to characterize the structure of a soil. Rather, multiple approaches have been developed, each targeted towards a particular research question or aspect of soil structure (Rabot et al., 2018; Vogel et al., 2021; Yudina and Kuzyakov, 2023). For example, the establishment of noninvasive imaging methods as a means to directly quantify and visualize pore networks in undisturbed soil is progressing rapidly and promises to become a standard in future soil research (Rabot et al., 2018; Schlüter et al., 2020). In addition to the pore network, another important aspect of a soil's structure is its mechanical properties (Kay, 1990; Or et al., 2021). As this cannot be visually assessed, another frequently measured indicator of soil structure is aggregate stability. While this term has come to mean different things depending on the context and spatial scale of research (Amézketa, 1999), it generally refers to the degree to which a soil remains aggregated under various physical, chemical, biological, and environmental stresses (Tisdall and Oades, 1982; Kemper and Rosenau, 1986; Six et al., 2000a; Papadopoulos, 2011). Soil aggregates, in turn, are broadly defined as two or more primary soil particles that cohere more strongly to each other than to neighboring particles (Martin et al., 1955; Kemper and Chepil, 1965; SSSA, 1997).
Soil aggregation occurs at multiple spatial scales and is driven by a variety of complex and dynamic biotic and abiotic interactions. The scale at which soil aggregation occurs, coupled with the specific mechanism(s) binding soil particles together, directly impacts the strength of these soil bonds, and thus overall aggregate stability (Yudina and Kuzyakov, 2023). A vital driver of soil aggregation is related to the proportion and types of iron and aluminum oxides and clay minerals in a given soil as well as to organic matter either applied externally or derived from plants and soil organisms, which forms organo-mineral complexes with clay particles and is crucial for soil carbon sequestration (Tisdall and Oades, 1982; Hemingway et al., 2019; Totsche et al., 2018). The degree to which aggregation occurs is simultaneously driven by multiple abiotic processes, including flocculation and cementation of clay particles as well as shrinking–swelling processes induced by changes in soil moisture and temperature (Bronick and Lal, 2005; Totsche et al., 2018; Pihlap et al., 2021). Together with bioturbation by macrofauna (Wilkinson et al., 2009; Piron et al., 2017) and activity of microorganisms and growing plant roots (Rillig and Mummey, 2006; Lehmann et al., 2017), these dynamic processes create soil aggregates and soil pores, both of which are important aspects of soil structure and regulators of soil functioning.
However, despite the long-standing acknowledgement of both soil pore spaces (Rabot et al., 2018; Vogel et al., 2021) and soil aggregates (Emerson, 1959; Edwards and Bremner, 1967; Chenu et al., 1998), some researchers have questioned the relevance of aggregates for soil processes. This doubt was first introduced over 3 decades ago (Letey, 1991) and has continued until the present, with recent debates on the function of aggregates (Kravchenko et al., 2019; Wang et al., 2019; Yudina and Kuzyakov, 2019). One of the main critiques of using aggregates to characterize soil structure and assess soil functioning is the inherent destruction of the soil required for such assessments (Young et al., 2001), the fact that aggregate properties depend on the method used to isolate them (Letey, 1991), and the unrealistic boundary conditions of isolated aggregates (Kravchenko et al., 2019; Vogel et al., 2021) that are completely separated from surrounding soil particles. Furthermore, it has been claimed that it is not possible to identify soil aggregates in X-ray images of consolidated undisturbed soil, or at least not with the same size and proportion as the soil aggregates measured with destructive measurement techniques (Baveye, 2020), although only very few attempts have been made to corroborate or falsify this claim (e.g., Koestel et al., 2021). Here, we argue that such in situ identification is not necessary and, in many cases, is not realistic for verifying the existence of soil aggregates.
This strong opposition between viewpoints on soil structure has led to a rift in the soil science community, essentially dividing researchers into two groups. Despite the well-accepted definition of soil structure that integrates both the solids and pore spaces (Dexter, 1988), in practice what we see today is one group focusing primarily on aggregates (the “solid-phase” or “aggregate” perspective) and the other on the pore network morphology (the “pore space” or “architecture” perspective), with very little overlap (Rabot et al., 2018; Vogel et al., 2021; Yudina and Kuzyakov, 2023). We believe that this scientific divide is not only unnecessary but is also in fact hindering the progression of research in the field of soil science. In an effort to reduce the confusion surrounding these apparently contradictory aspects of soil structure and to bring a foundation of shared understanding in the soil science community, here we discuss and illustrate how aggregates do not necessarily need to have a distinct physical boundary to exist in the soil profile. We do not attempt to choose a side in this debate, as we believe there is in fact no contradiction between these concepts for describing soil structure. Rather, we aim to demonstrate that there is no incongruity between the existence of aggregates and the fact that we often cannot see them in undisturbed soil, which we hope helps resolve some of the conflicting views and ultimately advances our understanding of soil functioning.
We believe that part of the controversy and confusion surrounding soil aggregates is rooted in conceptual models that display detached, isolated aggregates that seem to levitate (Fig. 1), whereas aggregates are often not visible in undisturbed soils or in deeper soil layers in reality (Fig. 2). This likely stems from research on soil structure emerging from the study of tilled, arable soils (Dexter, 1988; Elliott and Coleman, 1988; Or et al., 2021), where soil aggregates are indeed distinct units that are easily visible in the topsoil layers (Fig. 2a). Here, we argue that soil aggregates do indeed exist, but they do not necessarily look like these classic images of soil aggregates seen in drawings and found in arable fields (Figs. 1, 2a). While this distinction may be obvious for many in the soil science fields, there is apparently some confusion, wherein the simplistic, conceptual images created to highlight the mechanistic process of aggregate formation and disintegration are taken as a realistic depiction of soil aggregates. Here, we address this and show that, while this may be true in certain topsoils, this is rarely the case in undisturbed and deeper soil layers. In fact, one of the oldest and most widely used definitions of soil aggregates describe them as “any group of soil particles that coheres more strongly to each other than neighboring particles” (Martin et al., 1955; SSSA, 1997). Given this understanding of soil aggregates, it is then logical that they may be, but do not necessarily need to be, bordered by pore space. Our viewpoint challenges the concept of intra- versus inter-aggregate pore space: as aggregates cannot be surrounded on all sides by pores, there is not necessarily a distinctive inter-aggregate pore space. Instead, as soil aggregates within intact soils do not levitate, they logically must be in physical contact at a minimum of one point; thus, we argue that aggregate boundaries are rather defined by planes or regions of weaker cohesion. As a result, aggregates formed in situ will inherently not look the same as destructively isolated aggregates; rather, they will appear seamlessly embedded in the heterogeneous organo-mineral soil matrix, punctuated at various points by pore spaces, as described by Vogel et al. (2021).
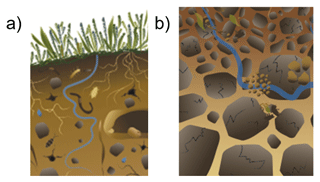
Figure 1Conceptualization of aggregates that are detached from each other and isolated within the soil yields confusion about aggregate boundaries and how they are embedded in soil. This illustrative example from FAO (2015) shows detached and isolated aggregates (dark brown) from (a) topsoils and (b) deeper soil horizons.
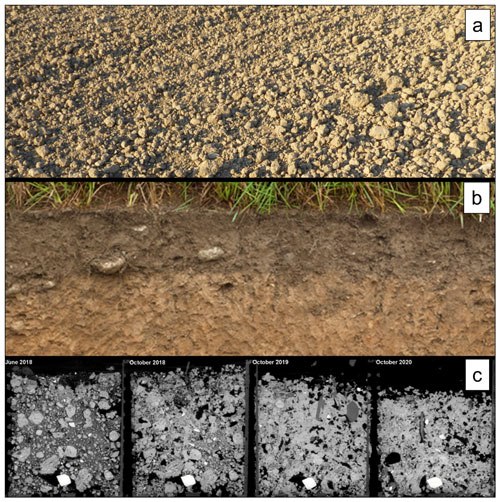
Figure 2Aggregates are clearly visible following tillage in topsoils but are indistinguishable at deeper soil horizons and over time. (a) Aggregates are clearly evident in a freshly tilled soil (photo by Dani Or, ETH Zurich, Switzerland) but are not visible in (b) deeper soil layers of undisturbed soils at the same spatial scale. (c) The temporal evolution of soil structure after tillage based on X-ray computed tomography images (visible pores >120 µm, based on voxel size of 60 µm): aggregates are clearly visible directly after tillage (left panel, June 2018) but coalesce and fuse with time (right panel, October 2020) (John Koestel, unpublished).
To illustrate this idea conceptually, we first show an example of two soil aggregates, each consisting of three soil particles (Fig. 3). For illustration purposes, we represent the different soil solids as simple single-unit-sized squares, with the outer edges of each square representing one or more of the various biotic and abiotic binding agents (i.e., microbial or plant-derived polysaccharides, electrostatic interactions between clay particles, mycorrhizal fungi, etc.). Here, we do not explicitly account for the nature of the binding agents and organic matter involved in the soil aggregation process, as the specific binding agents and mechanisms happen simultaneously and dynamically and differ depending on the local (micro-)climatic conditions, soil mineralogy and texture, biological components, and the scale at which aggregates are assessed (micrometer to meter). Furthermore, these processes have been described in detail and are not the focus of our discussion (Totsche et al., 2018; Yudina et al., 2018; Yudina and Kuzyakov, 2023). We instead highlight the relative strength of these binding agents between aggregate constituents, whereby the lines connecting two squares represent the bond strength between soil particles during a given period of time, with thicker lines indicating a higher bond strength (Fig. 3). In this example, we show that two separate soil aggregates can exist adjacent to each other without interaggregate pore space when there is either (a) a weaker inter-aggregate bond compared with the intra-aggregate bonds or (b) no binding force between adjacent aggregates, if we assume that these are pressed together (i.e., confined) by the surrounding soil structure (Figs. 2b and c and 3). Pore space between neighboring soil aggregates can, of course, also occur in cases when there is no binding force between adjacent aggregates (Fig. 3c), as evidenced, for example, by soil aggregates formed by tillage which can be seen with the unaided eye (Fig. 2a), or at smaller spatial scales where advanced imaging techniques are necessary to visualize this pore space.
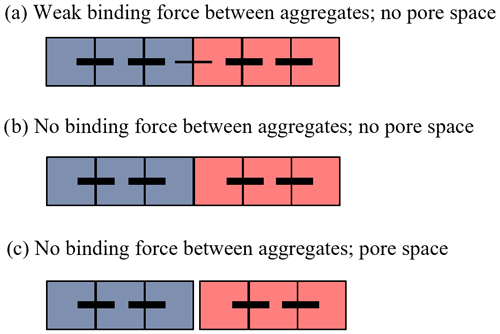
Figure 3Types of physical boundaries between aggregates. Simplified conceptual illustration showing the possible arrangements between two individual soil aggregates (indicated in blue and red). Soil particles are represented by squares; black horizontal lines indicate bonds between soil particles, with the bond strength indicated by line thickness. The three cases shown are examples of aggregates that (a) share a weak binding force between adjacent aggregates and therefore show no visible pore space, (b) have no binding force between adjacent aggregates but still show no visible pore space, and (c) have no binding force between aggregates and are physically distinct from each other, resulting in visible pore space between them.
As we have discussed, the term “soil aggregate” is used to indicate that certain soil particles cohere to each other more strongly than to neighboring particles. It does not give any indication of the size, shape, strength, or general arrangement of the particles and voids that make up that aggregate. In contrast to these naturally formed, in situ soil aggregates, the size, shape, and composition of destructively sampled soil aggregates are often described in relation to the technique used and the force applied to isolate them. Over the past decades, numerous methods have been developed to investigate and categorize soil aggregates, including wet sieving, dry sieving, drop–shatter tests, laser diffraction, sedimentation, and visual assessments (Yudina et al., 2018; Yudina and Kuzyakov, 2019). For example, microaggregates (<250 µm in diameter) have been shown to contain organic matter strongly stabilized via mineral associations that are relatively long-lasting and, thus, contribute more prominently to carbon sequestration, whereas macroaggregates (>250 µm in diameter) typically contain more labile particulate organic matter pools and, thus, are more strongly linked with microbial and plant community dynamics (Blanco-Canqui and Lal, 2004; Lavallee et al., 2019). While aggregates isolated from soils using such destructive techniques cannot be directly linked to in situ soil aggregates using current methods, understanding how differences in mineral–mineral and organo-mineral binding sites impact chemical and physical soil characteristics including soil porosity (Fukumasu et al., 2021; Weng et al., 2022) is paramount.
Given this aforementioned conceptual description of a soil aggregate, we now illustrate how they may form and subsequently dissipate within intact soils using the growth of a plant as an example (Fig. 4). We do not aim to model real processes nor discuss how the hierarchy of aggregate and pore formation influences their physical and functional properties (Yudina and Kuzyakov, 2023); instead, we aim to show how aggregates may appear and disappear as the binding strengths between soil aggregates and particles strengthen and weaken over time. To simplify our point, we start with a soil structure consisting of a heterogeneous mixture of aggregates (formed as described in Fig. 3), unaggregated primary soil particles and organic matter, and pore spaces prior to plant growth (t0 in Fig. 4). As the plant roots develop and elongate through the soil profile, the existing aggregates and unaggregated soil particles become bound to each other and neighboring particles through a combination of abiotic and biotic processes, as described above (t1 in Fig. 4). As the plant continues to grow, assimilating additional carbon-rich substances derived from the atmosphere via photosynthesis into the soil, this additional organic matter as well as the increased microbial activity surrounding these organic matter hot spots create further bonds between soil particles (t2 in Fig. 4). Additionally, changes in temperature and moisture patterns as well as the oxygen availability at microsites during plant growth impact various abiotic processes (e.g., shrinking–swelling and interactions of carbonates, clays, and iron and aluminum oxides), thereby further influencing aggregation either directly or through interactive effects with biological processes. Over time, this activity can either form new aggregates or combine two or more aggregates into larger aggregates, depending on the relative bond strengths between the soil particles. Once the plant completes its lifecycle and ultimately dies, its tissues are decomposed by soil microorganisms. As these more labile organic compounds are consumed and eventually depleted, the biological complexes and bonds between soil particles become weaker, and many of the relatively weakly bound aggregates eventually disintegrate (Tisdall and Oades, 1982; Oades, 1984; Six et al., 2004) (t3 in Fig. 4). However, the ease and speed at which these aggregates disintegrate, or “turnover” as it is often described (Six et al., 2000b), is directly related to the size and the strength of the bonds between particles. For example, some aggregates have relatively fast turnover times (between 30 and 88 d; De Gryze et al., 2006), whereas more strongly bound aggregates have been shown to endure for decades up to centuries (Totsche et al., 2018; Yudina and Kuzyakov, 2023) (t4 in Fig. 4). We note that disintegration does not necessarily mean physical separation; rather, the bonds between the soil particles of aggregates may simply weaken until they are no longer associated (see Fig. 4). Over time, any remaining aggregates will either dissipate completely or, in the case of the relatively more stable aggregates, can become incorporated into newly forming soil aggregates, where the cycle continues (tx in Fig. 4). It is important to note, however, that (even in our simplified example of one main process of root growth) soil particles are aggregated together heterogeneously and via numerous and simultaneously acting mechanisms, with distinct differences in aggregate stability depending on the specific combination of mechanisms involved (Yudina and Kuzyakov, 2023).
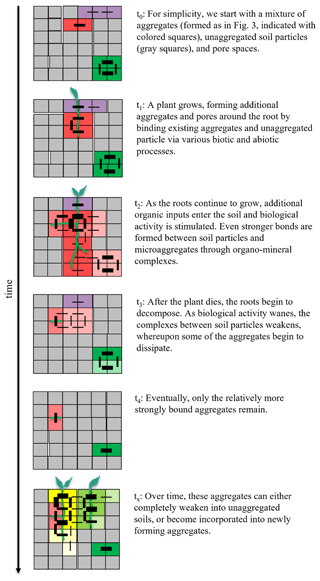
Figure 4Formation and dissipation of soil aggregates embedded in soil. In this conceptual illustrative example of in situ aggregate formation, unaggregated soil particles, existing soil aggregates (formed as in Fig. 3), and pore spaces are represented as individual gray squares, and the outer edges of each square represent one or more of the various different biotic and abiotic binding agents (i.e., microbial or plant-derived polysaccharides and electrostatic interactions between clay particles). The lines represent bonds connecting neighboring particles, with the relative strength of the bond indicated by the thickness of the line. The strength of bonds in this example are arbitrarily attributed but still mimic a realistic scenario of how mineral particles and their abiotically and biotically derived bonds and their decomposition result in the growth and disintegration of aggregates embedded in soil, without considering the exact nature of bonds (i.e., our illustration does not aim at explicitly simulating mechanisms). Different colors indicate different aggregates.
While the above simplified illustrative conceptualization is not novel in terms of describing the general formation and turnover processes of soil aggregates during plant growth and decay, we highlight the fact that these processes can, and most often do, occur within intact soils, with aggregates seamlessly embedded in soil. On the one hand, the fact that aggregates are not always visible in intact soils via various imaging techniques, just as in the classic representation of detached, isolated aggregates (i.e., Figs. 1, 2a), is logical, considering that they are surrounded by soil and must therefore be in physical contact to some degree (i.e., they are not levitating); on the other hand, we propose that there is also some confusion due to the issue of scale. For example, destructively isolated aggregates typically range from micrometers up to several centimeters in diameter, depending on the technique used and the force applied to separate them (Six et al., 2004; Nimmo, 2013; Yudina and Kuzyakov, 2019). Considering that aggregates have been described ranging in sizes of up to 10 000 times difference, they subsequently vary with respect to ease of visibility, from being seen with the unaided eye (Fig. 2a) to being visible only with microscopy (see Vidal et al., 2018) or X-ray imaging tools (Koestel et al., 2021; Fig. 2c). Therefore, what we actually see in an X-ray micro-computed tomography image, and can subsequently conclude regarding the arrangement of solids and pores, often greatly depends on the scale and resolution of a sample, as nicely illustrated in Lucas (2021). Moreover, if soil aggregates do not necessarily have to be bound by pore space, as we propose here, then current imaging techniques would not be able to visually capture these aggregates regardless of the scale, as we cannot “see” the mechanical dimension of the soil structure, i.e., the strength of the bonds between soil particles. Thus, to conclude that soil aggregates are not related to soil architecture simply because they are not visible at one particular spatial scale does not allow for a valid assessment of soil structure.
Understanding the role of soil structure in driving various soil functions and ecosystem services has been at the forefront of research for decades and has led to many important findings and advancements in analytical technologies. Nevertheless, despite this progress, much remains unknown regarding the dynamic interplay between the physical, chemical, and biological components as well and the pore spaces of a soil's structure and how they together drive soil functioning across different spatial scales. To echo Vogel et al. (2021), a holistic approach is necessary to link the effects of soil structure on soil functioning. However, despite the numerous advancements in technology, there is not one single methodological approach that can provide a complete overview of the three-dimensional arrangement of soil pores, the mechanical properties of soil structure, the composition and bioavailability of compounds contained in the solid mineral particles and organic matter, and the composition and activity of biological communities contained in a given space over time. Therefore, a more integrative assessment must inevitably consider multiple complementary approaches (see Schlüter et al., 2019).
The limitations of assessing soil aggregates and their relation to soil functions using classic destructive analytical techniques are clear and have been discussed in detail (Rabot et al., 2018; Kravchenko et al., 2019; Vogel et al., 2021). The questions of if, how, and at what scale the chemical and physical components of soil aggregates can be assessed in situ as well as how this is related to environmentally relevant functions depends on instrumental capabilities and the specific research question at hand (Gerke et al., 2021; Amelung et al., 2023). However, despite methodological limitations, continuing to investigate the spatial arrangement of solid particles and pores as well as the flow and transfer of substances throughout the soil pore network and how the solid particles are connected remains critical. Knowledge of the composition and bioavailability of substances bound in aggregates as well as how this influences soil microbial communities and soil fauna living within or on the surface of aggregates and pores is paramount to better understanding how soil structure drives functioning. With the understanding that soil aggregate formation and turnover does occur in intact soils, without the need for distinct physical boundaries, we hope that future research can unite these important aspects of soil chemical, physical, and biological properties with those of soil pore networks for a better representation of soil structure and the functions it provides.
The widespread use of conceptual figures showing detached and isolated aggregates is misleading and has largely contributed to the confusion about the function or relevancy of soil aggregates for soil processes. Based on the spatial scale investigated and the processes that contribute to their formation and turnover, it is clear that they can, but do not need to be, separated by distinct physical boundaries. The fact that we often do not see aggregates (e.g., in X-ray images) in undisturbed soils or deeper soil layers with distinct pore boundaries comparable to those in topsoils of freshly tilled arable soils or of destructively isolated soil aggregates does not mean that they do not exist, only that they are seamlessly embedded in the soil. Rather than furthering the divide between researchers in the opposing pore space or architectural perspective compared with the solid-phase or aggregate perspective, we support previous research emphasizing the vital point that aggregates and pore space are intimately linked and that both soil aggregation and soil pore formation are important for furthering our understanding of soil structure dynamics. However, the question of how aggregates that are seamlessly embedded in soil can be studied in situ to better understand the role of soil structure in microscale processes remains a considerable challenge, although a worthwhile and crucial future research goal.
No data sets were used in this article.
The idea for this paper was conceptualized by GG, JK, AJ, OH, SD, DO, and TK. GG and TK wrote the initial draft of the manuscript. All authors were involved in the review and editing the manuscript.
The contact author has declared that none of the authors has any competing interests.
Publisher’s note: Copernicus Publications remains neutral with regard to jurisdictional claims made in the text, published maps, institutional affiliations, or any other geographical representation in this paper. While Copernicus Publications makes every effort to include appropriate place names, the final responsibility lies with the authors.
All authors contributed to the conceptualization, discussion, and writing of the manuscript. In addition, we thank the members of the Soil Quality and Use group at Agroscope for their helpful contributions during coffee breaks.
This research was funded by Agroscope, ETH Zurich, and the Swiss National Science Foundation (grant no. 193118).
This paper was edited by Hu Zhou and reviewed by Anna Yudina and Alexandra Kravchenko.
Amelung, W., Tang, N., Siebers, N., Aehnelt, M., Eusterhues, V., Felde, G., Kaiser, K., Kögel-Knaber, I., Klumpp, E., Knief, C., Kruse, J., Lehndorff, E., Mikutta, R., Peth, S., Ray, N., Prechtel, A., Ritschel, T., Schweizer, S., Woche, S., Wu, B., and Totsche, K.: Architecture of soil microaggregates: Advanced methodologies to explore properties and functions, J. Plant Nut. Soil Sci., 1–34, https://doi.org/10.1002/jpln.202300149, 2023.
Amézketa, E.: Soil aggregate stability: A Review, J. Sustain. Agric., 14, 83–151, https://doi.org/10.1300/J064v14n02_08, 1999.
Baveye, P. C.: Bypass and hyperbole in soil research: Worrisome practices critically reviewed through examples, Eur. J. Soil Sci., 72, 1–20, https://doi.org/10.1111/ejss.12941, 2020.
Blanco-Canqui, H. and Lal, R.: Mechanisms of carbon sequestration in soil aggregates, Crit. Rev. Plant Sci., 23, 481–504, https://doi.org/10.1080/07352680490886842, 2004.
Bronick, C. J. and Lal, R.: Soil structure and management: a review, Geoderma, 124, 3–22, https://doi.org/10.1016/j.geoderma.2004.03.005, 2005.
Chenu, C., Puget, P., and Balesdent, J.: Clay-organic matter associations in soils: Microstructure and contribution to soil physical stability, Proceedings of the 16th World Congress of Soil Science, Montpellier, France, 20–26 August 1998, https://www.researchgate.net/publication/232322209_Clay-organic_matter_associations_in_soils_microstructure_and_contribution_to_soil_physical_stability (last access: 8 January 2024), 1998.
De Gryze, S., Six, J. and Merckx, R.: Quantifying water-stable soil aggregate turnover and its implication for soil organic matter dynamics in a model study, Eur. J. Soil Sci., 57, 693–707, https://doi.org/10.1111/j.1365-2389.2005.00760.x, 2006.
Dexter, A. R.: Advances in characterization of soil structure, Soil Till. Res., 11, 199–238, https://doi.org/10.1016/0167-1987(88)90002-5, 1988.
Edwards, A. P. and Bremner, J. M.: Microaggregates in soils, J. Soil Sci., 18, 64–73, https://doi.org/10.1111/j.1365-2389.1967.tb01488.x, 1967.
Elliott, E. T. and D. C. Coleman: Let the Soil Work for Us, Ecol. Bull., 39, 23–32, http://www.jstor.org/stable/20112982 (last access: 7 January 2024), 1988.
Emerson, W.: The structure of soil crumbs, J. Soil Sci, 10, 235–244, https://doi.org/10.1111/j.1365-2389.1959.tb02346.x, 1959.
Food and Agriculture Organization of the United Nations (FAO): International Year of Soils 2015 Infographics, https://www.fao.org/soils-2015/resources/information-material/en/ (last access: 13 December 2021), 2015.
Fukumasu, J., Jarvis, N., Koestel, J., Kätterer, T., and Larsbo, M.: Relations between soil organic carbon content and the pore size distribution for an arable topsoil with large variations in soil properties, Eur. J. Soil Sci., 73, e13212, https://doi.org/10.1111/ejss.13212, 2021.
Gerke, K. M., Korostilev, E. V., Romanenko, K. A., and Karsanina, M.: Going submicron in the precise analysis of soil structure: A FIB-SEM imaging study at nanoscale, Geoderma, 383, 114739, https://doi.org/10.1016/j.geoderma.2020.114739, 2021.
Hemingway, J. D., Rothman, D. H., Grant, K. E., Rosengard, S. Z., Eglinton, T. I., Derry, L. A., and Galy, V. V.: Mineral protection regulates long-term global preservation of natural organic carbon, Nature 570, 228–231, https://doi.org/10.1038/s41586-019-1280-6, 2019.
Kay, B. V.: Rates of change of soil structure under different cropping systems, in: Advances in Soil Science, edited by: Stewart, B. A., vol. 12, Springer, New York, NY, 1–41, https://doi.org/10.1007/978-1-4612-3316-9_1, 1990.
Kemper, W. D. and Chepil, W. S.: Size distribution of aggregates, In Methods of soil analysis: Part 1 physical and mineralogical properties, including statistics of measurement and sampling, vol. 9, American Society of Agronomy, Wisconsin, USA, 499–510, https://doi.org/10.2134/agronmonogr9.1.c39, 1965.
Kemper, W. D. and Rosenau, R. C.: Aggregate stability and size distribution, In Methods of soil analysis, part 1: Physical and mineralogical methods, Soil Sci. Soc. Amer. Inc., 425–442, https://doi.org/10.2136/sssabookser5.1.2ed.c17, 1986.
Koestel, J., Fukumasu, J., Garland, G., Larsbo, M., and Svensson, D.: Approaches to delineate aggregates in intact soil using X-ray imaging, Geoderma, 402, 115360, https://doi.org/10.1016/j.geoderma.2021.115360, 2021.
Kravchenko, A., Otten, W., Garnier, P., Pot, V., and Baveye, P. C.: Soil aggregates as biogeochemical reactors: Not a way forward in the research on soil–atmosphere exchange of greenhouse gases, Glob. Change Biol., 25, 2205–2208, https://doi.org/10.1111/gcb.14640, 2019.
Lal, R.: Soil structure and sustainability, J. Sustain. Agric., 1, 67–92, https://doi.org/10.1300/J064v01n04_06, 1991.
Lal, R., Bouma, J., Brevik, E., Dawson, L., Field, D. J., Glaser, B., Hatano, R., Hartemink, A. E., Kosaki, T., Lascelles, B., Monger, C., Muggler, C., Ndzana, G. M., Norra, S., Pan, X., Paradelo, R., Reyes-Sánchez, L. B., Sandén, T., Singh, B. R., Spiegel, H., Yanai, J., and Zhang, J.: Soils and sustainable development goals of the United Nations: An international union of soil sciences perspective, Geoderma Reg., 25, e00398, https://doi.org/10.1016/j.geodrs.2021.e00398, 2021.
Lavallee, J., Soong, J., and Cotrufo, M. F.: Conceptualizing soil organic matter into particulate and mineral-associated forms to address global change in the 21st century, Glob. Change Biol., 26, 261–273, https://doi.org/10.1111/gcb.14859, 2019.
Lehmann, A., Leifheit, E. F., and Rillig, M. C.: Chapter 14 – Mycorrhizas and Soil Aggregation, in: Mycorrhizal Mediation of Soil, edited by: Collins Johnson, N., Gehring, C., and Jansa, J., Elsevier, 241–262, Paperback ISBN 9780128043127, eBook ISBN 9780128043837, 2017.
Lehmann, J., Bossio, D., Kögel-Knabner, I., and Rillig, M.: The concept and future prospects of soil health, Nat. Rev. Earth Environ., 1, 544–553, https://doi.org/10.1038/s43017-020-0080-8, 2020.
Letey, J.: The study of soil structure- science or art, Aust. J. Soil Res., 29, 699–707, https://doi.org/10.1071/SR9910699, 1991.
Lucas, M.: Perspectives from the Fritz-Scheffer Awardee 2020 – The mutual interactions between roots and soil structure and how these affect rhizosphere processes, J. Plant Nutr. Soil Sci., 185, 8–18, https://doi.org/10.1002/jpln.202100385, 2021.
Martin, J., Martin, W., Page, J., Raney, W. A., and de Ment, J. D.: Soil aggregation, Adv. Agron., 7, 1–37, https://doi.org/10.1016/S0065-2113(08)60333-8, 1955.
Nimmo, J.: Aggregation: Physical Aspects, Reference Module in Earth Systems and Environmental Sciences, Elsevier, https://doi.org/10.1016/B978-0-12-409548-9.05087-9, 2013.
Oades, J. M.: Soil organic matter and structural stability: mechanisms and implications for management, Plant Soil, 76, 319–337, https://doi.org/10.1007/BF02205590, 1984.
Or, D., Keller, T., and Schlesinger, W.: Natural and managed soil structure: on the fragile scaffolding for soil functioning, Soil Till. Res., 208, 104912, https://doi.org/10.1016/j.still.2020.104912, 2021.
Papadopoulos, A.: Soil aggregates, structure, and stability, in: Encyclopedia of Agrophysics, Encyclopedia of Earth Sciences Series, edited by: Gliński, J., Horabik, J., and Lipiec, J., Springer, Dordrecht, the Netherlands, https://doi.org/10.1007/978-90-481-3585-1_142, 2011.
Pihlap, E., Steffens, M., and Kögel-Knabner, I.: Initial soil aggregate formation and stabilization in soils developed from calcareous loess, Geoderma, 385, 114854, https://doi.org/10.1016/j.geoderma.2020.114854, 2021.
Piron, D., Boizard, H., Heddadj, D., Peres, G., Hallaire, V., and Cluzeau, D.: Indicators of earthworm bioturbation to improve visual assessment of soil structure, Soil Till. Res., 173, 53–63, https://doi.org/10.1016/j.still.2016.10.013, 2017.
Rabot, E., Wiesmeier, M., Schlueter, S., and Vogel, H. J.: Soil structure as an indicator of soil functions: A review, Geoderma, 314, 122–137, https://doi.org/10.1016/j.geoderma.2017.11.009, 2018.
Rillig, M. C. and Mummey, D. L.: Mycorrhizas and soil structure, New Phytol., 171, 41–53, https://doi.org/10.1111/j.1469-8137.2006.01750.x, 2006.
Schlüter, S., Eickhorst, T., and Mueller, C. W: Correlative Imaging Reveals Holistic View of Soil Microenvironments. Environ. Sci. Technol., 53, 829–837, 2019.
Schlüter, S., Sammartino, S., and Koestel, J.: Exploring the relationship between soil structure and soil functions via pore-scale imaging, Geoderma, 370, 114370, https://doi.org/10.1016/j.geoderma.2020.114370, 2020.
Six, J., Elliott, E. T., and Paustian, K.: Soil structure and soil organic matter: II. A normalized stability index and the effect of mineralogy, Soil Sci. Soc. Am. J., 64, 1042–1049, https://doi.org/10.2136/sssaj2000.6431042x, 2000a.
Six, J., Elliott, E. T., and Paustian, K.: Soil macroaggregate turnover and microaggregate formation: a mechanism for C sequestration under no-tillage agriculture, Soil Biol. Biochem., 32, 2099–2103, https://doi.org/10.1016/S0038-0717(00)00179-6, 2000b.
Six, J., Bossuyt, H., Degryze, S., and Denef, K.: A history of research on the link between (micro)aggregates, soil biota, and soil organic matter dynamics, Soil Till. Res., 79, 7–31, https://doi.org/10.1016/j.still.2004.03.008, 2004.
Soil Science Society of America (SSSA): Glossary of soil science terms, SSSA, Madison, WI, https://www.soils.org/publications/soils-glossary/browse/ (last access: 8 January 2024), 1997.
Sullivan, P. L., Billings, S., Hirmas, D., Li, L., Zhang, X., Ziegler, S., Murenbeeld, K., Ajami, H., Guthrie, A., Singha, K., Gimenez, D., Duro, A., Moreno, V., Flores, A., Cueva, A., Koop, Aronson, E. L., Barnard, H. R., Banwart, S. A., Keen, R. M., Nemes, A., Nikolaidis, N. P., Nippert, J. B., Richter, D., Robinson, D. A., Sadayappan, K., de Souza, L. F. T., Unruh, M., and Wen, H.: Embracing the dynamic nature of soil structure: A paradigm illuminating the role of life in critical zones of the Anthropocene, Earth Sci. Rev., 225, 103873, https://doi.org/10.1016/j.earscirev.2021.103873, 2021.
Tisdall, J. M. and Oades, J. M.: Organic matter and water stable aggregates in soils, J. Soil Sci., 33, 141–163, https://doi.org/10.1111/j.1365-2389.1982.tb01755.x, 1982.
Totsche, K. U., Amelung, W., Gerzabek, M. H., Guggenberger, G., Klumpp, E. Knief, C., Lehndorff, E., Mikutta, R., Peth, S., Prechtel, A., Ray, N., and Kögel-Knabner, I.: Microaggregates in soils, J. Plant Nutr. Soil Sci., 181, 104–136, https://doi.org/10.1002/jpln.201600451, 2018.
Vidal, A., Hirte, J., Bender, S. F., Mayer, J., Gattinger, A., Höschen, C., Schädler, S., Iqbal, T., and Mueller, C.: Linking 3D soil structure and plant-microbe-soil carbon transfer in the rhizosphere, Front. Environ. Sci., 6, 9, https://doi.org/10.3389/fenvs.2018.00009, 2018.
Vogel, H. J., Balseiro-Romero, M., Kravchenko, A., Otten, W., Pot, V., Schlüter, S., Weller, U., and Baveye, P. C.: A holistic perspective on soil architecture is needed as a key to soil functions, Eur. J. Soil Sci., 73, e1315, https://doi.org/10.1111/ejss.13152, 2021.
Wang, B., Brewer, P. E., Shugart, H. H., Lerdau, M. T., and Allison, S. D.: Soil aggregates as biogeochemical reactors and implications for soil–atmosphere exchange of greenhouse gases – A concept, Glob. Chang. Biol., 25, 373–385, https://doi.org/10.1111/gcb.14515, 2019.
Weng, Z., Lehmann, J., Van Zwieten, L., Joseph, S., Archanjo, B., Cowie, B., Thomsen, L., Tobin, M., Vongsvivut, J., Klein, A., Doolette, C., Hou, H., Mueller, C., Lombi, E., and Kopittke, P.: Probing the nature of the soil organic matter, Crit. Rev. Environ. Sci. Technol., 52, 4072–4093, https://doi.org/10.1080/10643389.2021.1980346, 2022.
Wilkinson, M., Richards, P., and Humphreys, G.: Breaking ground: Pedological, geological, and ecological implications of soil bioturbation, Earth Sci. Rev., 97, 257–272, https://doi.org/10.1016/j.earscirev.2009.09.005, 2009.
Young, I. M., Crawford, J. W., and Rappoldt, C.: New methods and models for characterizing structural heterogeneity of soil, Soil Till. Res., 61, 33–45, https://doi.org/10.1016/S0167-1987(01)00188-X, 2001.
Yudina, A. and Kuzyakov, Y.: Saving the face of soil aggregates, Glob. Change Biol., 25, 3574–3577, https://doi.org/10.1111/gcb.14779, 2019.
Yudina, A. and Kuzyakov, Y.: Dual nature of soil structure: The unity of aggregates and pores, Geoderma, 434, 116478, https://doi.org/10.1016/j.geoderma.2023.116478, 2023.
Yudina, A., Fomin, D. S., Kotelnikova, A. D., and Milanovskii, E. Y.: From the notion of elementary soil particle to the particle-size and microaggregate-size distribution analyses: A review, Eur. J. Soil Sci., 51, 136–1347, https://doi.org/10.1134/S1064229318110091, 2018.