the Creative Commons Attribution 4.0 License.
the Creative Commons Attribution 4.0 License.
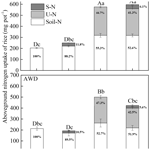
Effects of mild alternate wetting and drying irrigation and rice straw application on N2O emissions in rice cultivation
Kaikuo Wu
Wentao Li
Zhanbo Wei
Zhi Dong
Yue Meng
Na Lv
Lili Zhang
The shortage of water resources and the decline in soil organic matter (SOM) are critical limiting factors affecting the improvement in rice productivity, while alternate wetting and drying (AWD) irrigation and recycling application of rice straw (S) are considered favorable mitigation measures. However, the impact of such measures on rice yield and greenhouse gas (GHG) emissions, especially nitrous oxide (N2O) emissions, needs to be further clarified to ensure that agronomic practices save water, conserve soil, and reduce GHG emissions. Therefore, we explored the effects of mild AWD irrigation combined with on-site rice straw recycling on N2O emissions and rice yield through rice pot experiments. This experiment included 2 irrigation methods (continuous flooding (CF) irrigation and mild AWD irrigation), 2 nitrogen (N) application levels (0 and 225 kg N ha−1) and 2 rice straw return levels (0 and 9000 kg ha−1), for a total of 10 treatments, and each treatment had 3 replicates. The 15N-urea and 15N-S were added to the soil. The results showed that N2O emissions were primarily affected by urea application and irrigation methods, with urea application being most important. Compared with CF irrigation, mild AWD irrigation increased cumulative N2O emissions, with an average increase of 28.8 %. In addition, adding rice straw to mild AWD irrigation further stimulated N2O emissions by 18.1 %. Under the condition of urea application, compared with CF irrigation, mild AWD irrigation increased the yield-scaled N2O emissions by 17.9 %, and the addition of rice straw further promoted the yield-scaled N2O emissions under mild AWD irrigation by 17.4 % but reduced the global warming potential (GWP) (methane (CH4) + N2O) by 62.9 %. Under the condition of urea application, compared with CF irrigation, mild AWD irrigation reduced the uptake of soil-derived N and aboveground biomass of rice but did not reduce rice yield. Therefore, mild AWD irrigation combined with rice straw return may be a promising agronomic method to maintain rice yield, reduce GHGs, and protect or improve soil fertility.
- Article
(2197 KB) - Full-text XML
-
Supplement
(329 KB) - BibTeX
- EndNote
Rice is a staple food for more than half of the world's population and ensuring rice production is crucial to food security (Tang and Cheng, 2018). More than 135×106 ha of rice are cultivated worldwide, and approximately 90 % of paddy fields are submerged (J. Wang et al., 2017). Feeding a growing population under water scarcity will be a major challenge to Asia's food security in the coming decades (Lampayan et al., 2015). In China, more than 60 % of freshwater resources are consumed by rice cultivation every year, which represents a great waste of freshwater and causes many environmental problems, such as nonpoint source pollution, eutrophication, and greenhouse gas (GHG) emissions (Liao et al., 2020). Therefore, it is urgent to explore new methods for managing paddy field fertilization that can ensure high rice yield and reduced water waste and pollution.
Alternate wetting and drying (AWD) irrigation is an effective water-conserving irrigation method that can save approximately 23 % of freshwater resources compared with CF irrigation (Bouman and Tuong, 2001; Chu et al., 2014). There are usually two approaches to AWD irrigation: severe AWD irrigation (soil water potential kPa) and mild AWD irrigation (soil water potential kPa) (Zhou et al., 2017). Severe AWD irrigation could reduce rice yield by 22.6 % due to water stress, but under mild AWD irrigation, rice yield can be stable or slightly increased (Carrijo et al., 2017; Zhou et al., 2017). Therefore, mild AWD irrigation may offer a more promising paddy field management model. Previous studies have shown that AWD irrigation can significantly reduce CH4 emissions but considerably promotes N2O emissions, while global warming potential (GWP) mitigation is dependent on the magnitude of the increase in the release of N2O (Lagomarsino et al., 2016; Liang et al., 2017; Kritee et al., 2018; Tan et al., 2018). Therefore, exploring the impact of mild AWD irrigation on GHG emissions, especially N2O, is conducive to reducing paddy field emissions and maximizing agricultural and environmental benefits.
Long-term cultivation without organic matter supplementation leads to serious degradation of cultivated land and reduction in soil organic matter (SOM) content (Zhou et al., 2021), which is not conducive to rice production and sustainable agricultural development (Chen et al., 2016). Straw (S) return to the field is a valuable measure for improving SOM (Huang et al., 2021), and it is beneficial for reducing the environmental pollution caused by burning straw or discarding it randomly (Wang et al., 2018). In addition, rice straw return may cause changes in paddy field GHG emissions (Naser et al., 2007; Ye and Horwath, 2017; Sun et al., 2018; Yu et al., 2021), N-use efficiency (Liu et al., 2021), and rice yield (Chen et al., 2016; Ku et al., 2019). Although there have been many studies on mild paddy AWD irrigation or rice straw return, few studies have focused on the effects of mild AWD irrigation combined with rice straw return on rice cultivation. Under the conventional fertilization mode, whether mild AWD irrigation combined with rice straw return can achieve the optimal goals of water saving, yield increase and reduction in GHG emissions has remained unclear. Therefore, the purpose of this study was to investigate the effect of mild AWD irrigation combined with rice straw return on N2O emissions and rice yield in rice cultivation and to explore the supply of N to rice growth from the soil, urea and rice straw using 15N labeling technology. Our initial hypotheses were as follows: (1) mild AWD irrigation would promote N2O emissions in rice cultivation; and (2) mild AWD irrigation would maintain or promote rice yield.
2.1 Experimental setup
A pot experiment was conducted in an open greenhouse at the Shenyang Experimental Station of the Institute of Applied Ecology, Liaoning Province, China (43∘32′ N, 123∘23′ E) from 17 June to 27 October 2020. The test soil was an Alfisol with a total C content of 16.01 g kg−1 and a total N content of 1.36 g kg−1.
The pot experiment used a random block design, including 30 pots (30 cm diameter × 20 cm height). This experiment included two irrigation methods, two N application levels and two rice straw return levels, with three replicates of each combination, for a total of 30 rice pots (urea and straw were labeled with 15N, respectively). The two irrigation methods were continuous flooding (CF) irrigation and mild AWD irrigation. The CF irrigation maintained a water level depth of approximately 3–5 cm throughout the rice-growing season. Mild AWD irrigation water management in the first 7 d was consistent with CF irrigation and was allowed to evaporate under monitoring; when the soil negative pressure gauge reached −15 kPa, it was sub-flooded to a depth of 3–5 cm again and was then naturally allowed to dry again. This step was repeated until harvest. The CF and mild AWD irrigation were halted 2 weeks before harvest. Nitrogen was applied at 0 kg N ha−1 (control check (CK) and rice straw (15S)) and 225 kg N ha−1 (106.13 mg kg−1 dry soil) (urea (15U), urea + rice straw (U15S) and urea + rice straw (15US)). The abundance of urea 15N was 10.20 %. Urea was applied three times: base fertilizer 40 % (17 June), tiller topdressing 30 % (4 August) and heading topdressing 30 % (25 August). Rice straw return was applied at 0 kg ha−1 (CK and 15U) and 9000 kg ha−1 (4.25 g kg−1 dry soil) (15S, U15S and 15US). The total N content of unlabeled rice straw was 0.72 %, and the isotope abundance of 15N was 0.59 %. The total N content of labeled rice straw was 0.73 %, and the 15N isotope abundance was 22.94 %. The rice straw was ground and applied together with the base fertilizer. Phosphate fertilizer was superphosphate (150 kg P2O5 ha−1), and potassium fertilizer was potassium chloride (185 kg K2O ha−1) as a one-time application of basic fertilizer. Every pot was filled with 10.51 kg (9 kg dry soil) of sieved (2 mm) fresh soil. Two hills of rice were planted in each pot. At maturity, the rice yield and aboveground biomass were recorded after being oven-dried (105 ∘C for 0.5 h and 60 ∘C for 12 h).
2.2 Soil sample collection and analysis
At the stages of regreening, tillering, jointing, booting, filling and maturity, five points were randomly selected from the 0–10 cm soil layer of each pot and mixed. The soil NH-N and NO-N were extracted with 2 mol L−1 KCl solution (Wu et al., 2019), filtered and analyzed with a continuous flow analyzer (AA3, Bran + Luebbe, Germany). The extraction of soil 15N-NH-N followed Yu et al. (2020). Soil microbial biomass N (MBN) was fumigated with chloroform, extracted with 0.5 mol L−1 K2SO4 (soil : solution = 5 g : 20 mL) (Joergensen and Mueller, 1996), and determined by a total organic carbon (TOC) analyzer (Elementar vario TOC Analyzer, Germany). The soil 15N-NH-N content, 15N-MBN and 15N of rice aboveground biomass were determined by a stable isotope ratio mass spectrometer (253 MAT, Thermo Finnigan, Germany).
2.3 Gas sampling and calculation
The static chamber method was used to determine the N2O flux (J. L. Li et al., 2018). The static chamber with a top seal made of transparent plexiglass consisted of two parts, namely, the base and the gas-collecting chamber. The base had a diameter of 31 cm, a groove in the middle, and a height of 10 cm. The gas-collecting chamber had a diameter of 30 cm with a height of 70 cm. A small fan and a thermometer were installed in the gas-collecting chamber. Nitrous oxide (N2O) was collected every 2 d in the first week after fertilization or irrigation and every 7 d during other periods; N2O was sampled at 08:00–11:00 LT each sampling day. Every pot was sealed with water when N2O was collected. Three gas samples were collected at 0, 30 and 60 min after the chamber was airtight, and N2O was collected with a 50 mL injector and then injected into 200 mL gasbags.
The N2O concentration was analyzed using a gas chromatograph (Agilent 7890B, Gas Chromatograph, Delaware, USA). The calculation of N2O fluxes was as follows (J. L. Li et al., 2018):
where F is the N2O flux (µg m−2 h−1); ρ is the N2O standard-state density (1.964 kg m−3); h is the chamber height above the soil (m); c is the N2O concentration; is the slope of the N2O concentration curve, estimated using a linear regression model (Vitale et al., 2017); 273 is the gas constant; and T is the average air temperature inside the chamber during N2O collection (∘C).
Cumulative N2O emissions (CE) were calculated using the following formula according to Wang et al. (2011):
where i is the various sampling times, t is the sampling date, n is the total measurement time and 10−2 is the conversion factor.
The contribution of 15N markers to NH-N and MBN as well as the calculation of the N source of the aboveground biomass of rice followed Ma et al. (2015). Yield-scaled N2O was calculated as the ratio between N2O and rice yield (J. L. Li et al., 2018).
2.4 Statistical analysis
All analyses were performed using SPSS Statistics 16.0 (SPSS, Inc., Chicago, USA). One-way ANOVA was conducted to test the treatment effects with Duncan's test. Significance was set at P < 0.05. Univariate analysis of variance was used to analyze the response of cumulative N2O emissions to irrigation method, N level and rice straw application (Table 2). Tables and figures were prepared with Excel 2016 (Microsoft Corp., USA) and Origin 8 (Origin Lab Corp., USA), respectively. The data in the figures and tables are the average value ± standard error.
3.1 N2O flux
Three higher N2O flux peaks appeared after basal fertilizer and two topdressing treatments (Fig. 1), and the N2O flux peak after basal fertilizer application was significantly larger than the last two peaks. After basal fertilizer was applied, the N2O flux peaks of CF irrigation and mild AWD irrigation were similar. After the first topdressing, the N2O flux peak of mild AWD irrigation was significantly greater than that of CF irrigation, approximately 1.4 and 9.1 times under the U and US treatments, respectively. In contrast, the N2O flux peak of CF irrigation after the second topdressing was 3.5 and 1.6 times higher than that of mild AWD irrigation under the U and US treatments, respectively. In addition to the above three peaks, the N2O flux of CF irrigation was close to zero, and mild AWD irrigation had a lower flux peak with alternating wet and dry conditions. The flux of N2O ranged from −103.93 to 2770.50 µg m−2 h−1. The N2O flux appeared negative in the late stage of rice growth. The N2O fluxes of CF irrigation and mild AWD irrigation were similar in the later stage of rice growth, indicating that drainage had little effect on it. During the entire rice growth cycle, the N2O flux of the CK and S treatments was low. There were significant differences in the peak N2O fluxes between the different treatments. Compared with CK, the application of S alone significantly promoted the N2O flux on the first day after CF irrigation and mild AWD irrigation. Compared with U, the addition of S in CF irrigation reduced the N2O flux, while the addition of S in mild AWD irrigation increased the N2O flux.
3.2 Soil NH-N, NO-N and MBN concentrations
The soil NH-N, NO-N, and MBN concentrations varied with the growing stage of rice (Figs. 2a, c and 3a). The soil NH-N concentration first increased and then decreased. The NH-N concentration under CF irrigation and mild AWD irrigation was low in the late rice growth period (Fig. 2a). The concentration of NO-N in CF-irrigated soil showed a trend of first increasing and then decreasing and was at a low level in the later growth period of rice, while the concentration of NO-N in mild AWD irrigation showed a trend of first decreasing and then increasing, with repeated decreases and increases (Fig. 2c). The concentration of MBN in CF-irrigated soil first decreased and then increased and then decreased to a lower level, while the concentration of MBN in mild AWD-irrigated soil decreased with the growth stage of rice (Fig. 3a).
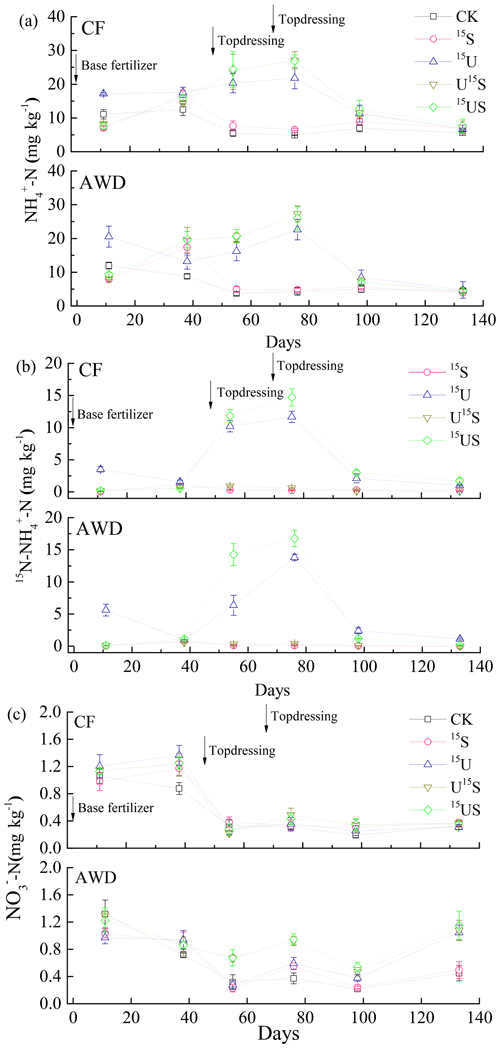
Figure 2Changes in soil ammonium nitrogen (NH-N) concentration (a), the contribution of 15N markers to NH-N (b), and changes in soil nitrate nitrogen (NO-N) concentration (c) during the growth period of rice (n=3).
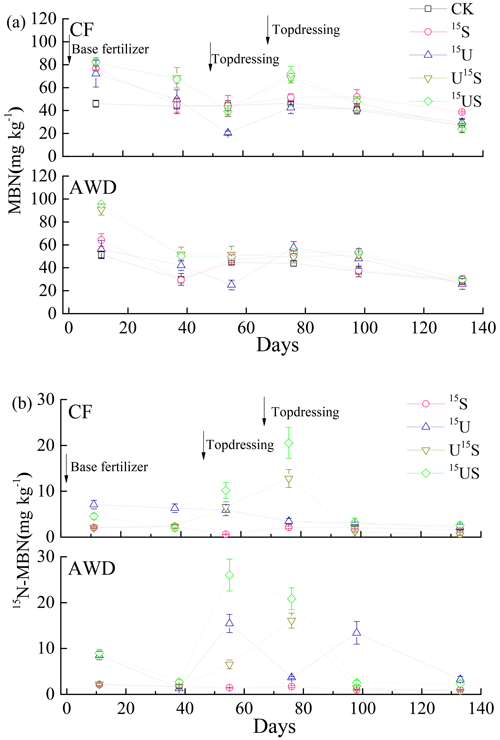
Figure 3Changes in the concentration of microbial biomass N (MBN) (a) in the soil during the growth period of rice and the contribution of 15N markers to MBN (b) (n=3).
There were significant differences in the NH-N, NO-N, and MBN concentrations between the different treatments. In CF irrigation and mild AWD irrigation, the U treatment had a higher NH-N concentration in the early stage of rice growth, while the other treatments had no significant difference, but as rice grew, the NH-N concentration of the US treatment increased, which was significantly greater than that of the U, S and CK treatments (Fig. 2a). In CF irrigation, the NO-N concentration of the U treatment was slightly higher than that of the other treatments, and all treatments had little variance. In mild AWD irrigation, the NO-N concentration of the US treatment was significantly higher than that of the U, S and CK treatments. The U treatment had a higher NO-N concentration than the S and CK treatments in the later stage of rice growth (Fig. 2c). The US treatment in CF irrigation and mild AWD irrigation had the highest MBN concentration during the growth period of rice (Fig. 3a).
Figure 2b shows that the NH-N in CF irrigation and mild AWD irrigation mainly came from urea rather than rice straw, and the combined application of urea and rice straw further promoted the release of NH-N from urea. Regardless of CF irrigation or mild AWD irrigation, rice straw N was difficult to utilize by microorganisms in the first year under single rice straw application, but the combined application of rice straw and urea significantly promoted the utilization of rice straw N by microorganisms. The application of urea combined with rice straw may be more easily utilized by microorganisms than urea applied alone (Fig. 3b).
3.3 Sources of aboveground biomass N in rice
As shown in Fig. 4, under CF irrigation and mild AWD irrigation, compared with CK, a single application of rice straw did not increase the aboveground N absorption of rice, while the U and US treatments significantly promoted the aboveground N absorption of rice. Under mild AWD irrigation, the US treatment reduced N uptake in rice shoots compared with the U treatment. The U and US treatments under CF irrigation promoted the N uptake of the aboveground rice more than those under mild AWD irrigation.
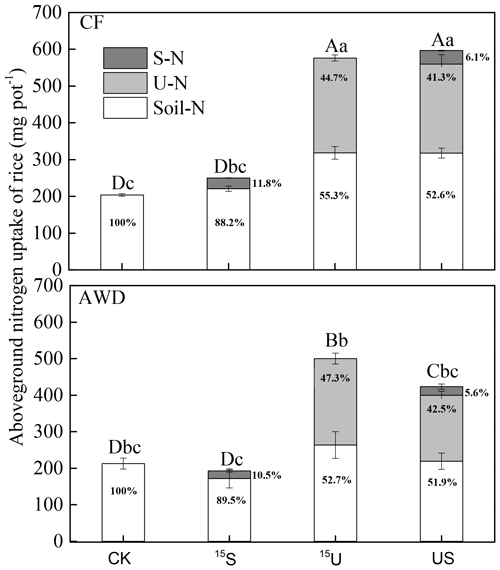
Figure 4The source of nitrogen in the aboveground biomass of rice at the maturity stage (n=3). CF: continuous flooding irrigation, AWD: mild alternate wetting and drying irrigation, Soil-N: soil-derived nitrogen, U-N: urea-derived nitrogen, S-N: Rice straw-derived nitrogen. Different capital letters indicate significant differences in total nitrogen uptake of rice above ground (P<0.05), and different lowercase letters indicate significant differences in soil-nitrogen supply (P<0.05).
With different irrigation methods, the effects of urea and rice straw addition on the N absorption of the aboveground rice were varied. Compared with the CK and S treatments, the U and US treatments under CF irrigation significantly promoted the absorption of soil-N by rice, while only the S and U treatments had significant differences under mild AWD irrigation. Compared with mild AWD irrigation, the U and US treatments under CF irrigation significantly promoted the absorption of soil-N by rice. Regardless of the irrigation and fertilization method, the soil was the main source of N in the aboveground parts of rice, followed by urea and finally rice straw (Fig. 4).
3.4 Cumulative N2O emissions, rice agronomic properties and yield-scaled N2O emissions
In addition to the CK treatment, compared with CF irrigation, mild AWD irrigation significantly promoted the accumulation of N2O during the growth period of rice, with an average increase of 28.8 % (Table 1). Under CF irrigation, there was no significant difference in the accumulation of N2O between S and CK or between US and U. However, the addition of rice straw under mild AWD irrigation significantly increased the accumulation of N2O by 18.1 % (Table 1). Compared with the CK and S treatments, the U and US treatments significantly promoted cumulative N2O emissions under the two irrigation modes. As shown in Table 2, irrigation methods, N application level, and rice straw return affected cumulative N2O emissions, of which the N application level had the greatest impact. The interaction between irrigation level and N fertilizer or rice straw significantly affected cumulative N2O emissions.
Table 1Effects of different treatments on cumulative nitrous oxide (N2O) emissions, rice aboveground biomass, rice yield and yield-scaled N2O emissions. The values denote means ± standard errors (n=3). Different lowercase letters indicate significant differences (P<0.05).
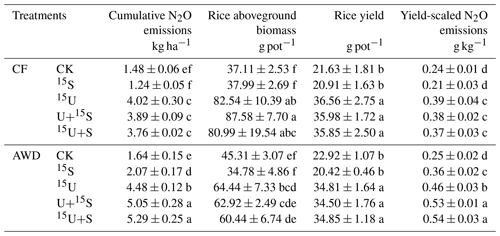
As shown in Table 1, compared with CF irrigation, mild AWD irrigation significantly reduced rice aboveground biomass under US treatment but had no effect on other treatments. Regardless of whether CF irrigation or mild AWD irrigation was applied, there was no significant difference in the rice aboveground biomass between S and CK or between US and U. Compared with the CK and S treatments, the U and US treatments significantly promoted the rice aboveground biomass under the two irrigation modes. Irrigation level had no effect on rice yield under all treatments. Regardless of CF irrigation or mild AWD irrigation, rice yield under the U and US treatments was significantly higher than that under the CK and S treatments, but there was no difference between the former two and the latter two.
In addition to the CK treatment, compared with CF irrigation, mild AWD irrigation significantly promoted yield-scaled N2O emissions during the rice growth period. Under urea application conditions, compared with CF irrigation, mild AWD irrigation increased yield-scale N2O emissions by 17.9 %, and the addition of rice straw further promoted yield-scale N2O emissions by 17.4 % under mild AWD irrigation conditions (Table 1). Regardless of CF irrigation or mild AWD irrigation, yield-scaled N2O emissions under the U and US treatments were significantly higher than those under the CK and S treatments. Rice straw addition had no effect on the yield-scaled N2O emissions under CF irrigation but significantly increased the yield-scaled N2O emissions under mild AWD irrigation.
4.1 Effects of irrigation methods, N levels and rice straw return on N2O emissions
N2O emissions are significantly affected by water-filled pores and mineral N (NH-N and NO-N) content (Allen et al., 2010). The N2O emission peak in CF irrigation occurred only after N application, while mild AWD irrigation caused other N2O emission peaks, which might have been caused by the change in soil moisture conditions by mild AWD irrigation (Zhou et al., 2020). The peak of N2O after fertilization may be because a large amount of N application increases the soil inorganic-N concentration (Fig. 2a and c), which in turn promotes the generation of N2O, which comes from the denitrification process (L. Wang et al., 2017; Yano et al., 2014). During the denitrification process, it is easier for microorganisms to use NO-N as an electron acceptor (Fig. 2c), which affects the reduction process of N2O, resulting in an increase in the ratio of in the denitrification products (Pérez et al., 2000). Our results showed a negative N2O emission flux at the later stage of rice growth, which may be due to the decrease in surface soil N2O concentration due to the strengthening of the N2O reduction process or the weakening of the N2O diffusion process in the soil profile, which allowed atmospheric N2O to diffuse back into the soil (Chapuis-Lydie et al., 2007). Mild AWD irrigation promoted cumulative N2O emissions by 28.8 % on average, which was as proposed in our Hypothesis 1. Similar results were found in previous studies, which may be due to the increased N2O produced by nitrification and denitrification due to water-level alternation (Liang et al., 2017; Zhou et al., 2020) and temperature change (Wu et al., 2019) of mild AWD irrigation. To reduce N2O emissions from paddy fields, researchers generally regulate N2O production by optimizing N management (Liang et al., 2017), applying inhibitors to control the N supply rate of N fertilizers (Wu et al., 2019). In both CF and mild AWD irrigation, there was no obvious N2O emission peak at the later growth stage of rice, which may have been due to the decrease in soil inorganic-N content (Fig. 2a and c) and microbial biomass (Fig. 3a).
Compared with CK and S, N fertilizer application (U and US) significantly increased cumulative N2O emissions and was the most notable factor in N2O generation (Table 2), mainly because N fertilizer application provided sufficient substrates for soil nitrification and denitrification to generate N2O (Fiedler et al., 2017; Wu et al., 2021). The peak of N2O emissions after base fertilizer application was larger than that after two topdressings, which might have been due to a higher N application rate and simultaneous nitrification and denitrification during the initial flooding (Mathieu et al., 2006; L. Wang et al., 2017). The peak of N2O emissions after the two topdressing treatments with CF irrigation was similar, while the peak of N2O emissions after the first topdressing treatment with mild AWD irrigation was significantly larger than that following the second topdressing treatment. This may be because the soil environment (temperature, moisture, etc.) changed little during long-term flooding under CF irrigation (Lagomarsino et al., 2016; Verhoeven et al., 2018; Congreves et al., 2019), while the variations in the soil environment of the two topdressings under mild AWD irrigation changed the utilization of fertilizer N by microorganisms (Fig. 3b). Therefore, reducing the amount of the first topdressing under mild AWD irrigation and maintaining flooding for approximately 1 week after fertilization may benefit N2O reduction (Liao et al., 2020). The negative value of N2O emissions appeared at the later stage of rice growth, indicating that the paddy field could also become a sink for N2O (van Groenigen et al., 2015), which might be caused by the lack of N supply at the later stage. Increasing the second topdressing might be beneficial for alleviating N deficiency (Liao et al., 2020).
Table 2Cumulative nitrous oxide (N2O) emissions in response to irrigation method, nitrogen level and straw returning. Significant treatment effects within a main category (P<0.05) were indicated by * and indicated significant treatment effects within a main category (P<0.001).
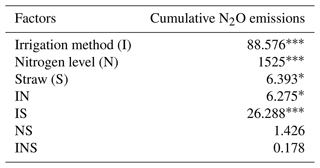
Unlike the CF irrigation, the addition of rice straw under mild AWD irrigation conditions promoted N2O emissions (Table 1), probably because the alternation of wet and dry conditions promoted the decomposition of rice straw (Andren et al., 1993; Buchen et al., 2016; Chen et al., 2016), which was beneficial to the growth of microorganisms (Fig. 3a), thus promoting the production of N2O (Said-Pullicino et al., 2014; Wang et al., 2018; Wu et al., 2021). In addition, compared with U and S, US promoted microbial absorption of urea and rice straw N (Fig. 3b), which also proved that US treatment was more conducive to the growth of microorganisms.
4.2 Effects of irrigation methods, N levels and rice straw return on rice production and yield-scaled N2O emissions
The change in irrigation method did not cause differences in rice yield (Table 1), but under the US treatment, mild AWD irrigation significantly reduced the aboveground biomass of rice and the uptake of soil N by rice (Table 1 and Fig. 4), which was consistent with our Hypothesis 2. Previous studies have also shown that mild AWD irrigation can stabilize or increase rice yield. This may be because mild AWD irrigation can promote the transport of nutrients from stems and leaves to grains during the reproductive growth stage of rice while inhibiting ineffective tillering and increasing the number of effective panicles, thereby reducing excessive vegetative growth of rice (Carrijo et al., 2017; Z. Li et al., 2018; Liao et al., 2020; Zhang et al., 2009). This may also be an important reason for the decrease in the uptake of soil N by rice under AWD irrigation. Urea application was a key factor in improving rice yield (J. Wang et al., 2017), but it also aggravated soil N uptake by rice, and soil was the largest source of N for rice in all treatments (Fig. 4). Compared with U, under CF irrigation and mild AWD irrigation, US reduced the uptake of soil-derived N by rice, and the trend was more obvious under mild AWD irrigation. Although the trend was not significant, rice straw return may be an effective way to maintain long-term soil fertility (Fig. 4).
In our study, mild AWD irrigation, urea application and rice straw return all increased yield-scaled N2O emissions (Table 1), mainly due to improved soil aeration and increased inorganic N and rice straw decomposition, resulting in more N2O production (Andren et al., 1993; Buchen et al., 2016; Chen et al., 2016; Lagomarsino et al., 2016; Fiedler et al., 2017; Verhoeven et al., 2018; Congreves et al., 2019; Wu et al., 2021). Although mild AWD irrigation had higher yield-scaled N2O emissions than CF irrigation, the GWP (CH4 + N2O) under mild AWD irrigation was significantly lower than that under CF irrigation and decreased by 8.1 %, 57.9 %, 11.8 % and 62.9 % under CK, S, U and US, respectively (Table S1 in the Supplement). Therefore, mild AWD irrigation combined with rice straw return may be a promising agronomic measure that maintains rice yield, slows greenhouse effects (CO2 emissions are not considered), and also reduces soil fertility consumption.
The effects of irrigation methods, N levels and rice straw return on N2O emissions were explored through pot experiments using rice. We found that N2O emissions were affected by urea application and irrigation methods, with urea application being the most important. Compared with CF irrigation, mild AWD irrigation increased cumulative N2O emissions, with an average increase of 28.8 %. In addition, adding rice straw to mild AWD irrigation further stimulated N2O emissions by 18.1 %. Under the condition of urea application, compared with CF irrigation, mild AWD irrigation increased the yield-scaled N2O emissions by 17.9 %, and the addition of rice straw further promoted the yield-scaled N2O emissions under mild AWD irrigation by 17.4 % but reduced the GWP (CH4 + N2O) by 62.9 %. Under the condition of urea application, compared with CF irrigation, mild AWD irrigation reduced the uptake of soil-derived N and aboveground biomass of rice but did not reduce rice yield. Therefore, mild AWD irrigation combined with rice straw return may offer a promising agronomic measure to maintain high rice yield, reduce greenhouse effects, and maintain or improve soil fertility.
The data that support the findings of this study are available by request from the corresponding author (Lili Zhang).
The supplement related to this article is available online at: https://doi.org/10.5194/soil-8-645-2022-supplement.
KW, WL, ZW and ZD conceived and designed the experiments; YM and NL performed the experiments; KW analyzed the data; KW and LZ wrote the paper and all authors approved submission of the paper. All authors have read and agreed to the published version of the manuscript.
The contact author has declared that none of the authors has any competing interests.
Publisher's note: Copernicus Publications remains neutral with regard to jurisdictional claims in published maps and institutional affiliations.
The authors thank the editors and reviewers for their constructive comments and suggestions.
This work was supported by the Strategic Priority Research Program of the Chinese Academy of Sciences (grant no. XDA28090200), the National Scientific Foundation Project of China (grant no. 31971531), the Liaoning “Take the Lead” Project of 2021: Arable Land Productivity Improvement Strategies Development and Promotion (grant no. 2021JH1/10400039-2), the K.C.Wong Education Foundation (Research and application of environmental-friendly polymer compound fertilizers 2022-5), and the Youth Start-up Fund (grant no. 2022000008).
This paper was edited by Ping He and reviewed by two anonymous referees.
Allen, D. E., Kingston, G., Rennenberg, H., Dalal, R. C., and Schmidt, S.: Effect of nitrogen fertilizer management and waterlogging on nitrous oxide emission from subtropical sugarcane soils, Agr. Ecosyst. Environ., 136, 209–217, 2010.
Andren, O., Rajkai, K., and Katterer, T.: Water and temperature dynamics in a clay soil under winter wheat: influence on straw decomposition and N immobilization, Biol. Fert. Soils, 15, 1–8, 1993.
Bouman, B. A. M. and Tuong, T. P.: Field water management to save water and increase its productivity in irrigated lowland rice, Agr. Water Manage., 49, 11–30, 2001.
Buchen, C., Lewicka-Szczebak, D., Fuß, R., Helfrich, M., Flessa, H., and Well, R.: Fluxes of N2 and N2O and contributing processes in summer after grassland renewal and grassland conversion to maize cropping on a Plaggic Anthrosol and a Histic Gleysol, Soil Biol. Biochem., 101, 6–19, 2016.
Carrijo, D. R., Lundy, M. E., and Linquist, B. A.: Rice yields and water use under alternate wetting and drying irrigation: A meta-analysis, Field Crop. Res., 203, 173–180, 2017.
Chapuis-Lydie, L., Wrage, N., Metay, A., Chotte, J., and Bernoux, M.: Soils, a sink for N2O? A review, Global Change Biol., 13, 1–17, 2007.
Chen, A., Xie, X., Dorodnikov, M., Wang, W., Ge, T., Shibistova, O., Wei, W., and Guggenberger, G.: Response of paddy soil organic carbon accumulation to changes in long-term yield-driven carbon inputs in subtropical China, Agr. Ecosyst. Environ., 232, 302–311, 2016.
Chu, G., Chen, T., Wang, Z., Yang, J., and Zhang, J.: Morphological and physiological traits of roots and their relationships with water productivity in water-saving and drought-resistant rice, Field Crop. Res., 162, 108–119, 2014.
Congreves, K. A., Phan, T., and Farrell, R. E.: A new look at an old concept: using 15N2O isotopomers to understand the relationship between soil moisture and N2O production pathways, SOIL, 5, 265–274, https://doi.org/10.5194/soil-5-265-2019, 2019.
Fiedler, S. R., Augustin, J., Wrage-Mönnig, N., Jurasinski, G., Gusovius, B., and Glatzel, S.: Potential short-term losses of N2O and N2 from high concentrations of biogas digestate in arable soils, SOIL, 3, 161–176, https://doi.org/10.5194/soil-3-161-2017, 2017.
Huang, W., Wu, J. F., Pan, X. H., Tan, X. M., Zeng, Y. J., Shi, Q. H., Liu, T. J., and Zeng, Y. H.: Effects of long-term straw return on soil organic carbon fractions and enzyme activities in a double-cropped rice paddy in South China, J. Integr. Agr., 20, 236–247, 2021.
Joergensen, R. G. and Mueller, T.: The fumigation-extraction method to estimate soil microbial biomass: Calibration of the k(EN) value, Soil Biol. Biochem., 28, 33–37, 1996.
Kritee, K., Nair, D., Zavala-Araiza, D., Proville, J., Rudek, J., Adhya, T. K., Loecke, T., Esteves, T., Balireddygari, S., Dava, O., Ram, K., S, R. A., Madasamy, M., Dokka, R. V., Anandaraj, D., Athiyaman, D., Reddy, M., Ahuja, R., and Hamburg, S. P.: High nitrous oxide fluxes from rice indicate the need to manage water for both long- and short-term climate impacts, P. Natl. Acad. Sci. USA, 115, 9720–9725, 2018.
Ku, H. H., Ryu, J. H., Bae, H. S., Jeong, C., and Lee, S. E.: Modeling a long-term effect of rice straw incorporation on SOC content and grain yield in rice field, Arch. Agron. Soil Sci., 65, 1941–1954, 2019.
Lagomarsino, A., Agnelli, A. E., Linquist, B., Adviento-Borbe, M. A., Agnelli, A., Gavina, G., Ravaglia, S., and Ferrara, R. M.: Alternate wetting and drying of rice reduced CH4 emissions but triggered N2O peaks in a clayey soil of central Italy, Pedosphere, 26, 533–548, 2016.
Lampayan, R. M., Faronilo, J. E., Tuong, T. P., Espiritu, A. J., de Dios, J. L., Bayot, R. S., Bueno, C. S., and Hosen, Y.: Effects of seedbed management and delayed transplanting of rice seedlings on crop performance, grain yield, and water productivity, Field Crop. Res., 183, 303–314, 2015.
Li, J. L., Li, Y. E., Wan, Y. F., Wang, B., Waqas, M. A., Cai, W. W., Guo, C., Zhou, S. H., Su, R. S., Qin, X. B., Gao, Q. Z., and Wilkes, A.: Combination of modified nitrogen fertilizers and water saving irrigation can reduce greenhouse gas emissions and increase rice yield, Geoderma, 315, 1–10, 2018.
Li, Z., Li, Z., Muhammad, W., Lin, M. H., Azeem, S., Zhao, H., Lin, S., Chen, T., Fang, C. X., Letuma, P., Zhang, Z. X., and Lin, W. X.: Proteomic analysis of positive influence of alternate wetting and moderate soil drying on the process of rice grain filling, Plant Growth Regul., 84, 533–548, 2018.
Liang, K., Zhong, X., Huang, N., Lampayan, R. M., Liu, Y., Pan, J., Peng, B., Hu, X., and Fu, Y.: Nitrogen losses and greenhouse gas emissions under different N and water management in a subtropical double-season rice cropping system, Sci. Total Environ., 609, 46–57, 2017.
Liao, B., Wu, X., Yu, Y., Luo, S., Hu, R., and Lu, G.: Effects of mild alternate wetting and drying irrigation and mid-season drainage on CH4 and N2O emissions in rice cultivation, Sci. Total Environ., 698, 134212, https://doi.org/10.1016/j.scitotenv.2019.134212, 2020.
Liu, J., Jiang, B., Shen, J., Zhu, X., Yi, W., Li, Y., and Wu, J.: Contrasting effects of straw and straw-derived biochar applications on soil carbon accumulation and nitrogen use efficiency in double-rice cropping systems, Agr. Ecosyst. Environ., 311, 107286, https://doi.org/10.1016/j.agee.2020.107286, 2021.
Ma, Q., Wu, Z., Shen, S., Zhou, H., Jiang, C., Xu, Y., Liu, R., and Yu, W.: Responses of biotic and abiotic effects on conservation and supply of fertilizer N to inhibitors and glucose inputs, Soil Biol. Biochem., 89, 72–81, 2015.
Mathieu, O., Henault, C., Leveque, J., Baujard, E., Milloux, M. J., and Andreux, F.: Quantifying the contribution of nitrification and denitrification to the nitrous oxide flux using 15N tracers, Environ. Pollut., 144, 933–940, 2006.
Naser, H. M., Nagata, O., Tamura, S., and Hatano, R.: Methane emissions from five paddy fields with different amounts of rice straw application in central Hokkaido, Japan, Soil Sci. Plant Nutr., 53, 95–101, 2007.
Pérez, T., Trumbore, S. E., Tyler, S. C., Davidson, E. A., Keller, M., and de Camargo, P. B.: Isotopic variability of N2O emissions from tropical forest soils, Global Biogeochem. Cy., 14, 525–535, 2000.
Said-Pullicino, D., Cucu, M. A., Sodano, M., Birk, J. J., Glaser, B., and Celi, L.: Nitrogen immobilization in paddy soils as affected by redox conditions and rice straw incorporation, Geoderma, 228, 44–53, 2014.
Sun, L., Ma, Y., Li, B., Xiao, C., Fan, L., and Xiong, Z.: Nitrogen fertilizer in combination with an ameliorant mitigated yield-scaled greenhouse gas emissions from a coastal saline rice field in southeastern China, Environ. Sci. Pollut. R., 25, 15896–15908, 2018.
Tan, X., Shao, D., and Gu, W.: Effects of temperature and soil moisture on gross nitrification and denitrification rates of a Chinese lowland paddy field soil, Paddy Water Environ., 16, 687–698, 2018.
Tang, D. and Cheng, Z.: From Basic Research to Molecular Breeding – Chinese Scientists Play A Central Role in Boosting World Rice Production, Genom. Proteom. Bioinf., 16, 389–392, 2018.
van Groenigen, J. W., Huygens, D., Boeckx, P., Kuyper, T. W., Lubbers, I. M., Rütting, T., and Groffman, P. M.: The soil N cycle: new insights and key challenges, Soil, 1, 235–256, 2015.
Verhoeven, E., Decock, C., Barthel, M., Bertora, C., Sacco, D., Romani, M., Sleutel, S., and Six, J.: Nitrification and coupled nitrification-denitrification at shallow depths are responsible for early season N2O emissions under alternate wetting and drying management in an Italian rice paddy system, Soil Biol. Biochem., 120, 58–69, 2018.
Vitale, L., Polimeno, F., Ottaiano, L., Maglione, G., Tedeschi, A., Mori, M., De Marco, A., Di Tommasi, P., and Magliulo, V.: Fertilizer type influences tomato yield and soil N2O emissions, Plant Soil Environ., 63, 105–110, 2017.
Wang, B., Shen, X., Chen, S., Bai, Y., Yang, G., Zhu, J., Shu, J., and Xue, Z.: Distribution characteristics, resource utilization and popularizing demonstration of crop straw in southwest China: A comprehensive evaluation, Ecol. Indic., 93, 998–1004, 2018.
Wang, J., Zhao, Y., Zhang, J., Zhao, W., Müller, C., and Cai, Z.: Nitrification is the key process determining N use efficiency in paddy soils, J. Plant Nutr. Soil Sc., 180, 648–658, 2017.
Wang, J. Y., Jia, J. X., Xiong, Z. Q., Khalil, M. A. K., and Xing, G. X.: Water regime–nitrogen fertilizer–straw incorporation interaction: Field study on nitrous oxide emissions from a rice agroecosystem in Nanjing, China, Agr. Ecosyst. Environ., 141, 437–446, 2011.
Wang, L., Sheng, R., Yang, H., Wang, Q., Zhang, W., Hou, H., Wu, J., and Wei, W.: Stimulatory effect of exogenous nitrate on soil denitrifiers and denitrifying activities in submerged paddy soil, Geoderma, 286, 64–72, 2017.
Wu, K., Zhang, Z., Feng, L., Bai, W., Feng, C., Song, Y., Gong, P., Meng, Y., and Zhang, L.: Effects of corn stalks and urea on N2O production from corn field soil, Agronomy-Basel, 11, 2009, https://doi.org/10.3390/agronomy11102009, 2021.
Wu, K. K., Gong, P., Zhang, L. L., Wu, Z. J., Xie, X. S., Yang, H. Z., Li, W. T., Song, Y. C., and Li, D. P.: Yield-scaled N2O and CH4 emissions as affected by combined application of stabilized nitrogen fertilizer and pig manure in rice fields, Plant Soil Environ., 65, 497–502, 2019.
Yano, M., Toyoda, S., Tokida, T., Hayashi, K., Hasegawa, T., Makabe, A., Koba, K., and Yoshida, N.: Isotopomer analysis of production, consumption and soil-toatmosphere emission processes of N2O at the beginning of paddy field irrigation, Soil Biol. Biochem., 70, 66–78, 2014.
Ye, R. and Horwath, W. R.: Influence of rice straw on priming of soil C for dissolved organic C and CH4 production, Plant Soil, 417, 231–241, 2017.
Yu, C., Xie, X., Yang, H., Yang, L., Li, W., Wu, K., Zhang, W., Feng, C., Li, D., Wu, Z., and Zhang, L.: Effect of straw and inhibitors on the fate of nitrogen applied to paddy soil, Sci. Rep.-UK, 10, 21582, https://doi.org/10.1038/s41598-020-78648-w, 2020.
Yu, C., Zhang, L., Yang, L., Bai, W., Feng, C., Li, W., Wu, K., Li, D., and Wu, Z.: Effect of a urea and urease/nitrification inhibitor combination on rice straw hydrolysis and nutrient turnover on rice growth, Bioresources, 16, 3059–3074, 2021.
Zhang, H., Xue, Y. G., Wang, Z. Q., Yang, J. C., and Zhang, J. H.: An Alternate Wetting and Moderate Soil Drying Regime Improves Root and Shoot Growth in Rice, Crop Sci., 49, 2246–2260, 2009.
Zhou, Q., Ju, C. X., Wang, Z. Q., Zhang, H., Liu, L. J., Yang, J. C., and Zhang, J. H.: Grain yield and water use efficiency of super rice under soil water deficit and alternate wetting and drying irrigation, J. Integr. Agr., 16, 1028–1043, 2017.
Zhou, S., Sun, H., Bi, J., Zhang, J., Riya, S., and Hosomi, M.: Effect of water-saving irrigation on the N2O dynamics and the contribution of exogenous and endogenous nitrogen to N2O production in paddy soil using 15N tracing, Soil Till. Res., 200, 104610, https://doi.org/10.1016/j.still.2020.104610, 2020.
Zhou, Y., Li, X., and Liu, Y.: Cultivated land protection and rational use in China, Land Use Policy, 106, 105454, https://doi.org/10.1016/j.landusepol.2021.105454, 2021.