the Creative Commons Attribution 4.0 License.
the Creative Commons Attribution 4.0 License.
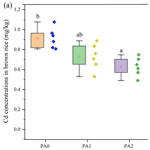
The application of biochar and oyster shell reduced cadmium uptake by crops and modified soil fertility and enzyme activities in contaminated soil
Jia Li
Mingping Sheng
He Peng
Dinghua Peng
Heng Xu
Soil pollution with cadmium (Cd) has been threatening the human health. In this study, we investigated the possibility of applying biochar and oyster shell to reduce Cd uptake by crops and modify soil quality. A field study based on the rice–oilseed rape rotation was done, and the treatments were comprised without amendments (PA0) and with 15 000 kg ha−1 biochar (PA1), 15 000 kg ha−1 oyster shell (PA2), and 7500 kg ha−1 biochar and 7500 kg ha−1 oyster shell (PA3). Results revealed that both oyster shell and biochar reduced the HOAc-extractable Cd in soil. Compared to PA0, the HOAc-extractable Cd in the PA1, PA2, and PA3 treatments was reduced by 4.76 %–20.79 %, 17.86 %–38.61 %, and 5.95 %–10.89 %, respectively. The cooperative application of biochar and oyster shell reduced the Cd accumulation in brown rice and oilseed by 29.67 % and 19.74 %, respectively, compared to control and thus decreased the hazard quotient (HQ) from the consumption of brown rice and oilseed. The addition of biochar slightly increased soil organic matter. In addition, the available P in the PA2 and PA3 treatments was significantly (p<0.05) increased by 200.96 %–295.92 % and 187.46 %–280.04 % compared to the control. Moreover, the cooperative application of biochar and oyster shell enhanced the activities of urease, catalase, and β-galactosidase by 139.44 %–147.56 %, 10.71 %–34.31 %, and 82.08 %–244.38 %, respectively. These results demonstrated that the utilization of biochar and oyster shell might be an effective pathway to reducing Cd uptake by crops and improving soil fertility and enzyme activities.
- Article
(1881 KB) - Full-text XML
-
Supplement
(191 KB) - BibTeX
- EndNote
Cadmium (Cd) contamination of agricultural soils is a worldwide environmental problem which has been seriously threatening to human health (Yang et al., 2021). The excessive intake of Cd by the food chain can cause serious damages to the bones, thyroid, and kidneys (Y. Ma et al., 2021). According to the latest national survey on the status of soil environmental quality in China, Cd has been ranked as the highest contaminant (7 %) among all heavy metals (Mou et al., 2020). In southwestern China, intensive industrialization is the reason for the farmlands being contaminated with Cd (Chen et al., 2018). Soil acidification also aggravated the bioavailability and solubility of Cd, thus enhancing Cd uptake by crops (Feng et al., 2020). Therefore, the development of cost-effective and eco-friendly remediation technologies is crucial for food safety and soil quality.
In recent years, the in situ immobilization technology through the application of soil amendments has raised attention widely in the remediation of Cd-contaminated farmlands, which could reduce the Cd uptake by plants without delaying agricultural production (Palansooriya et al., 2020). Biochar derived from bio-wastes has been widely recommended as a soil amendment (Zong et al., 2021). The amounts of nutrients (such as C, N, P, K, and Mg) in biochar can improve soil fertility and promote plant growth (Lu et al., 2015). Moreover, biochar has a large surface area and plenty of functional groups (such as −COOH, C−O, and C=O), which are reactive to immobilize heavy metals, including Cd, lead (Pb), and nickel (Ni; Wang et al., 2021). However, the high price of biochar limits its large application. In addition, the application of biochar cannot effectively change soil pH in acidic fields; thus, it cannot effectively reduce the bioavailability of Cd in soil (Liu et al., 2018). Oyster shell is a low-cost and largely available bio-waste product from oyster farming (Li et al., 2020). Oyster shell is a promising slow-release alkaloid, which has outstanding effects on pH adjustment and Cd immobilization in soil (Lee et al., 2008). In this sense, we think that the joint use of biochar and oyster shell might be a low-cost and effective pathway to decreasing Cd uptake by crops and improving soil quality in acidic fields.
Rice and oilseed rape are the main food and economic crops in southwestern China, and the rice–oilseed rape rotation is the dominant production model (Liu et al., 2014). Previous studies mainly focused on the effects of amendments for reducing the Cd uptake by rice (Tang et al., 2020; Yin et al., 2022), while the remediation efficiency of amendments under the rice–oilseed rape rotation is little known. Based on the above opinions, a field experiment under the rice–oilseed rape rotation was designed (1) to investigate the effects of biochar and oyster shell on Cd immobilization in soil, (2) to evaluate the effects of biochar and oyster shell on decreasing human health risk of consuming contaminated crops, and (3) to reveal the effects of biochar and oyster shell on soil fertility and enzyme activities.
2.1 Experimental site and soil properties
A field trial was conducted during 2019–2020 in a rice–oilseed rape rotation cultivated site where the soil was moderately contaminated with Cd. The field site was located in a dominant agricultural cultivation region around industrial parks in Chengdu plain, Sichuan province, China (104∘18′ N, 31∘81′ E). This region belongs to a subtropical monsoon humid climate, with an average temperature of 16.1 ∘C and annual rainfall of about 1000 mm. The main properties of the topsoil (0–20 cm) collected from the site in 2019 and 2020 were shown in Table S1 in the Supplement.
2.2 Characteristics of experimental materials
Biochar was purchased from Zhenjiang Zedi Agricultural and Biological Co., Ltd., which was produced from rice straw in a reactor with N2 and 500 ∘C for about 4 h. Oyster shell was purchased from Fujian Mata Ecological Technology Co., Ltd (<0.3 mm mesh). The main properties of biochar and oyster shell are presented in Table S1 in the Supplement. The main composition of oyster shell is shown in Table S2. The surface structures of biochar and oyster shell were analyzed by scanning electron microscope (SEM; JSM-7500F). The functional groups of biochar and oyster shell were measured by Fourier transform infrared spectra (Nicolet 6700). The seeds of rice (Yixiang 2115) and seeds of oilseed rape (Yiyou 15) were obtained from the Rice Research Institute, Sichuan Academy of Agricultural Science.
2.3 Experimental setup
The field experiment was conducted during 2019–2020. The treatments were PA0 (control), PA1 (15 000 kg ha−1 of biochar), PA2 (15 000 kg ha−1of oyster shell), and PA4 (7500 kg ha−1 and 7500 kg ha−1 of biochar and oyster shell, respectively). The concentrations of biochar and oyster shell used in this study were derived from a the previous report (Ameloot et al., 2014). Each experimental plot was 56 m2 (7 × 8 m) and arranged in a randomized design with three replicates. Before rice planting, the amendments were sufficiently mixed with topsoil. After harvesting the rice, the oilseed rape was planted following the conventional tillage pattern without extra amendments.
2.4 Plant analysis
The rice grain and oilseed samples were dried and ground to powder. Then, 0.2 g samples were digested with in a mixture of (), and the mixture was then diluted into 10 mL with 1 % HNO3 (Wu et al., 2019b). The Cd concentrations in the mixture were determined by atomic absorption spectroscopy (AAS; Varian SpectrAA 220Fs).
2.5 Soil analysis
Soil pH was determined by a pH meter (Mettler S220) with a soil water ratio of 5 g 25 mL. The bioavailable Cd of soil was measured by the TCLP (toxicity characteristic leaching procedure) method (Xu et al., 2020). Briefly, 2 g of soil sample was mixed with 40 mL of 0.11 M acetic acid (HOAc) and shaken for 16 h at 25 ∘C, 150 rpm (revolutions per minute). The mixture was centrifuged for 5 min at 8000 rpm, and then the supernatant was collected to determine Cd concentrations by AAS. Available P, available K, and available N were measured according to the method described by Wu et al. (2018). Soil TOC (total organic carbon) and OM (organic matter) were determined by the method described by Walz et al. (2017).
In addition, activities of soil enzyme were analyzed to reflect the biological quality in this study. Dehydrogenase activity was evaluated by the production of triphenylfornazan (TPF) at OD492nm and expressed as µg TPF g−1 of soil per 24 h (Benefield et al., 1977). Acid phosphate activity was assayed by the p-nitrophenol (pNP) release at OD400nm and expressed as µg−1 pNP g−1 of soil per 24/,h (van Aarle and Plassard, 2010). Urease activity was determined by the NH4 complex at OD578nm and expressed as µg−1 NH4-N g−1 of soil per 24/,h (Yan et al., 2013). Catalase activity was measured by the back-titration of H2O2 added to soil with 0.1 M KMnO4 and expressed as mL 0.1 M KMnO4 g−1 of soil per hour (Zhang et al., 2011). Invertase activity was assayed by the amount of glucose production at OD508nm and expressed as µg−1 glucose g−1 of soil per 24/,h (Wu et al., 2019b). β-galactosidase activity was measured by the released 4-methylumbelliferone (MUF) and expressed as µg−1 MUF µmol g−1 of soil per hour (Martínez-Iñigo et al., 2009).
2.6 Human health risk assessment of consuming crops
The human health risks of consuming crops were assessed by the hazard quotient (HQ) according to the method introduced by Environmental Protection Agency (EPA) in the USA (Wei et al., 2020). When HQ is lower than 1, it demonstrates no risk for human health (Mehdizadeh et al., 2021). Hazard quotient values were calculated using the following equation:
where the IR (ingestion rate) for rice grain is 0.3892 kg d−1 for adults and 0.1984 kg d−1 for children, respectively. For rape oil, the IR is 0.025 kg d−1 for adults and 0.0125 kg d−1 for children, respectively.
2.7 Statistical analysis
In this study, statistical significance was analyzed using the SPSS (Statistical Package for the Social Sciences) 18.0 software package, and means values were considered to be different when P <0.05, using the least significant difference (LSD). Figures were performed using Origin 8.0 (OriginLab, USA).
3.1 Characteristics of soil and amendments
The main characteristics of soil, biochar, and oyster shell are shown in Table S1. The soil was acidic soil, with pH values of 5.27–5.51. The biochar and oyster shell used in the field study were alkaline materials, and their pH values were 8.22 and 8.52, respectively. The OM of biochar (54.15 %) was significantly higher than that of soil (3.93 %) and oyster shell (1.26 %). The carbon percentage of biochar was 92.50 %.
The surface of the oyster shell (Fig. 1a) was a filamentous layer with some disordered deposition, which might be calcium compounds. The structure of biochar (Fig. 1b) was lamellar and polyporous, which might be in favor of Cd absorption. In addition, Fourier transform infrared spectroscopy (FTIR) was operated to detect functional groups of oyster shell and biochar (Fig. 1c). The characteristic peaks of calcium carbonate in oyster shell were observed at 1427 and 879 cm−1 (Lu et al., 2021). Biochar showed obvious peaks at 1089 and 790 cm−1, which were related to the C-O and C-H bending vibration, respectively (Wu et al., 2019a). In addition, an obvious feature at 3436 cm−1 corresponding to −OH was loaded on oyster shell and biochar (Lian et al., 2021).
3.2 Analysis of soil Cd bioavailability
To evaluate the effect of different amendments on Cd bioavailability, the concentrations of HOAc-extractable Cd in soils were determined by the TCLP (toxicity characteristic leaching procedure) method (Halim et al., 2003). Figure 2 showed the variations in HOAc-extractable Cd with different amendments in the rice–oilseed rape rotation. Both biochar and oyster shell resulted in the reduction of HOAc-extractable Cd in soils. In the rice planting, the HOAc-extractable Cd in the PA1, PA2, and PA3 treatments was significantly decreased by 20.79 %, 40.59 %, and 10.89 %, respectively, compared to the control. In the oilseed rape planting, the HOAc-extractable Cd in the PA1, PA2, and PA3 treatments was also reduced by 5.76 %, 17.85 %, and 5.95 % respectively, compared to the control. The Cd immobilization efficiency in the PA3 treatment was higher than that in the PA1 treatment, which demonstrated that the addition of oyster shell could strength the Cd immobilization capacity of biochar.
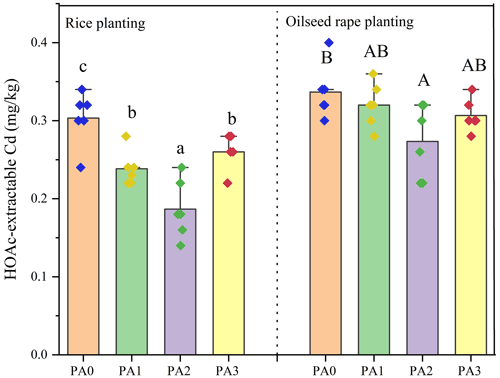
Figure 2The effects of amendments on Cd bioavailability in soil. Dots represent the value of each sample. Bars followed with different lowercase letters (a–c) and capital letters (A and B) indicate the significant (p≤0.05) difference among different treatments in rice planting and oilseed rape planting according to the LSD test. Values represent means ± standard deviation.
3.3 Analysis of Cd concentrations in brown rice and oilseed
As shown in Fig. 3, the application of biochar and oyster shell reduced the Cd concentrations in brown rice and oilseed. In the PA0 treatment, the Cd concentration in brown rice was 0.91 mg kg−1. Compared to the control (PA0), the Cd concentration in brown rice decreased by 20.88 % and 30.77 %, respectively, in the PA1 and PA2 treatments. The Cd concentration in oilseed was reduced in the PA1 and PA3 by about 27.63 % and was 19.74 % lower than that in PA0, respectively. Moreover, the cooperative application of biochar and oyster shell contributed to a higher reduction in Cd in brown rice (29.67 %) than that in biochar alone (20.88 %).
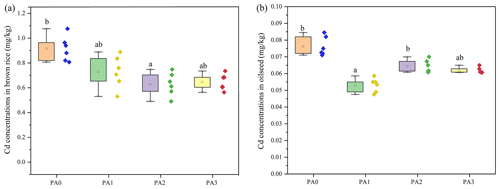
Figure 3The effects of the amendments on the Cd concentrations in brown rice (a) and oilseed (b). Dots represent the value of each sample. Bars with different lowercase letters indicate the significant (p<0.05) difference among different treatments according to the LSD test. Values represent means ± standard deviation.
3.4 Health risk assessment of consuming crops
Hazard quotient values of consuming crops in different treatments were analyzed. The HQ order of consuming rice and oilseed was children > adult females > adult males, which indicated that children had more of a health risk than adults for the intake of contaminated crops (Fig. 4). Without the application of amendments, the HQ values of consuming brown rice for adult males, adult females, and children were 5.66, 6.44, and 7.07, respectively. For children, HQ values for brown rice intake in PA1, PA2, and PA3 decreased by 20.87 %, 31.11 % and 29.76 %, respectively, compared to the control. In addition, it was also observed that the application of amendments decreased the HQ values of consuming oilseed by 17.27 %–28.14 % compared to the control.
3.5 Analysis of soil pH and cation exchange capacity
It was observed that soil pH was weakly increased by biochar but significantly increased by oyster shell (Fig. 5a). After the oyster shell application, the soil pH increased from acid (5.2–5.5) to neutral (6.9–7.3). Meanwhile, the cooperative application of biochar and oyster shell also increased soil pH to 7.10–7.24. The application of oyster shell slightly increased the cation exchange capacity (CEC) of soil in the rice planting, while both oyster shell and biochar had no significant effects on the CEC of soil in the oilseed rape planting (Fig. 5b).
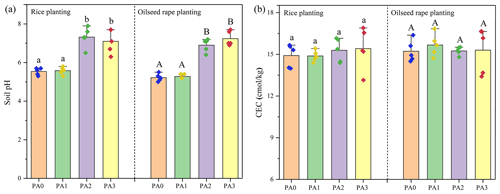
Figure 5The effects of different amendments on soil pH (a) and CEC (b). Dots represent the value of each sample. Bars followed with different lowercase letters (a–c) and capital letters (A and B) indicate the significant (p≤0.05) difference among different treatments in rice planting and oilseed rape planting according to the LSD test. Values represent means ± standard deviation.
3.6 Analysis of soil fertility
To analyze the effects of amendments on soil fertility, the concentrations of TOC, OM, available P, available K, and available N were determined during the rice–oilseed rape rotation (Table S3). Biochar application slightly increased TOC and OM in the rice–oilseed rape rotation. In the rice planting, soil TOC and OM in the PA3 treatment increased by 10.09 % and 9.92 %, respectively, compared to the control. In the oilseed rape planting, soil TOC and OM in the PA1 treatment were enhanced by 11.06 % and 11.32 %, respectively, compared to the control. More obviously, available P was significantly increased by the addition of oyster shell. Compared to the control, the available P significantly increased by 200.96 %–295.92 % and 184.73 %–187.46 %, respectively, in the PA2 and PA3 treatments.
3.7 Analysis of soil enzyme activities
As shown in Fig. 6, adding amendments variously changed the activities of soil enzymes. In the rice–oilseed rape rotation, the application of biochar (PA1) increased the dehydrogenase activity to about 20.12 %–25.49 % higher than that of the control (PA0). Urease activity was markedly enhanced by the oyster shell treatment. Compared to the control, urease activity significantly increased by 205.56 %–268.88 % and 139.44 %–147.56 %, respectively, in the PA2 and PA3 treatments. However, biochar had no obvious effect on the activities of acid phosphate and invertase, but oyster shell significantly reduced the acid phosphate activity by 43.30 % in the rice planting. In addition, the cooperative application of biochar and oyster shell enhanced the activities of catalase and β-galactosidase activity by 10.71 %–34.31 % and 82.08 %–244.38 %, respectively, compared to the control.
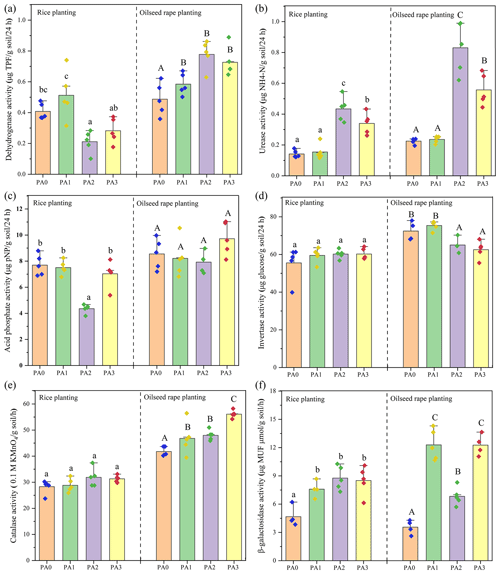
Figure 6The effects of different amendments on the activities of soil enzymes. Dots represent the value of each sample. Bars followed with different lowercase letters (a–c) and capital letters (A–C) indicate the significant (p≤0.05) difference among different treatments in rice planting and oilseed rape planting according to the LSD test. Values represent means ± standard deviation.
3.8 Analysis of correlation coefficient
The Pearson correlation analysis was used to analyze the relationship among different parameters. As shown in Fig. 7a, the Cd concentration in brown rice was positively correlated to Cd bioavailability (r=0.90) but negatively correlated to soil pH (). Meanwhile, the activities of soil enzymes, except acid phosphate, were positively connected to available N, available P, available K, and TOC. Figure 7b showed a weak correlation between Cd uptake by oilseed rape and Cd bioavailability. Soil pH was positively correlated to available P and β-galactosidase activity (r>0.95), which further demonstrated that alkaline substances could increase available P and β-galactosidase activity by adjusting soil pH in acidic fields.
3.9 Cost approach for amendments
Considering the remediation of large areas of the contaminated agricultural soil, the cost of amendments is a key parameter in the practical application. The market price of biochar (>USD 180 t−1) was much higher than that of oyster shell (USD 75 t−1; see the Supplement). In this study, the dosage of amendments was 15 000 kg ha−1. The cost of the biochar amendment was at least USD 270 ha−1, while the joint use of biochar and oyster shell decreased the cost of amendments by 29.17 %. Based on these results, the collaborative application of biochar and oyster shell might be an economical pathway to immobilizing Cd and improving soil properties.
3.10 Discussion
Rice and oilseed rape are the most important crops worldwide. Simultaneously, the rice–oilseed rape rotation was the main cultivated model in China (Huang et al., 2020). However, the Cd contamination in agricultural lands, especially in acidic soils, has severely threatened food production safety and human health (Shi et al., 2022). Cd accumulation in the crops poses a great human health risk due to the Cd uptake by crops possibly resulting in kidney damage and adverse effects on the lungs and cardiovascular and musculoskeletal systems (Wei et al., 2020).
In situ immobilization was an effective pathway to decreasing the Cd uptake by crops through the application of amendments (Kumpiene et al., 2008). In this study, two bio-wastes, namely biochar and oyster shell, were used to decrease the Cd uptake by crops and modify the soil quality. The application of biochar and oyster shell both reduced the HOAc-extractable Cd in soil (Fig. 2). The HOAc-extractable Cd has been widely used to evaluate the bioavailability of Cd in soils (Liu et al., 2021). Previous studies have revealed that biochar had a great potential to immobilize Cd by surface absorption and co-precipitation (He et al., 2019; Liu et al., 2018). However, the reduction of the HOAc-extractable Cd in the oyster shell treatments was significantly higher than that in the biochar treatments, which might result from the enhancement of soil pH in the oyster shell treatments (Lee et al., 2008). Soil pH is one of the main factors influencing the bioavailability of Cd in soils (Huang et al., 2020). It has been widely verified that soil pH determines the solid solution equilibria of heavy metals in soils (Zhao and Masaihiko, 2007). Oyster shell has been regarded as a low-release alkaloid in soils due to primarily consisting of CaCO3 (Ok et al., 2010). The dissolution of CaCO3 from oyster shell can produce hydroxyl ion (OH−; Ok et al., 2010). The increase in soil pH can result in the increase in the negative soil surface charge, which easily causes an increased capacity of cationic metal adsorption (Ok et al., 2010). The precipitants of metal oxy hydroxides could be formed due to increased hydroxyl ions (Bolan et al., 2014). The Cd uptake by crops was positively related to the Cd bioavailability in soil (Huang et al., 2020). Similar to other reports (Jing et al., 2020; Mehdizadeh et al., 2021), the Cd concentrations in brown rice and oilseed decreased after the application of biochar and oyster shell. Furthermore, the health risk related to the polluted crops consumption with Cd has been estimated by HQ, and the decreased HQ values demonstrated that the human health risk from consuming crops decreased through the application of amendments (L. Ma et al., 2021).
Soil nutrients play an important role in soil quality and plant growth. Phosphorus fractions are mainly dependent on soil pH, soil mineralogy, and the application of phosphate fertilizer (Lee et al., 2008). Fe-P and Al-P are the predominant forms in acidic soils, while calcium-bound P is the predominant form in alkaline soils (Dean, 1949). In acidic soils, the loosely bound phosphates are gradually converted into Fe-P and Al-P fractions, owing to the re-precipitation process. Previous studies found that the concentration of available P reached the maximum at neutral pH soils (Lee et al., 2008). Our results showed that the addition of oyster shell markedly increased the concentration of available P in soils, which might result from the enhancement of soil pH (Table S3). A correlation analysis (Fig. 7) further demonstrated that available P was highly correlated to the changes of soil pH (r>0.99). The concentrations of available K and available N also slightly increased with the application of biochar and oyster shell, indicating an improvement in soil fertility.
Activities of soil enzymes have been widely used to reflect soil biological quality (Lin et al., 2021). In this study, the activities of dehydrogenase, urease, catalase, and β-galactosidase increased following the treatments of biochar and oyster shell (Fig. 6). Dehydrogenase usually reflects the microbial degradation capacity for organic matter (Campos et al., 2019). Urease is often used as a biochemical indicator to reflect soil fertility, which played a crucial role in soil nitrogen mineralization (Lebrun et al., 2012). The addition of oyster shell increased the soil pH, which usually results in the enhancement of dehydrogenase and urease activities (Wen et al., 2021). Abd El-Azeem et al. (2013) reported that dehydrogenase activity was positively correlated to soil pH. Oyster shell can raise the urease activity, thus catalyzing the hydrolysis of urea to CO2 and NH3, with an optimum pH of around 7.4 (Lee et al., 2008). Soil β-galactosidase plays an important role in the microbial glycometabolism, and the significant increase in β-galactosidase by the application of biochar indicates a shift in the use of soil organic carbon from plant-derived sugars towards more recalcitrant C compounds (Giagnoni et al., 2019). In addition, the porous structure and rich nutrients of biochar can contribute to the growth of soil microorganisms and thus might increase the activities of soil enzymes (Liao et al., 2016). Moreover, the enhancement of enzyme activities in biochar and oyster shell treatments might also be related to the decrease in Cd bio-toxicity in the soil (Zhang et al., 2021). In conclusion, the enhancement of the activities of soil enzyme indicated that the cooperative application of biochar and oyster shell could improve the soil biological properties.
The current study revealed the impacts of applying oyster shell and biochar on Cd bioavailability, Cd uptake by crops, the human health risk of consuming contaminated crops, soil fertility, and enzyme activities during the rice–oilseed rape rotation. The application of oyster shell significantly (p<0.05) increased soil pH and thus decreased the bioavailability of Cd in soil. The cooperative application of biochar and oyster shell significantly reduced the Cd concentrations in crops and the human health risk of consuming contaminated brown rice and oilseed. In addition, the application of biochar increased OM and TOC, while the addition of oyster shell was suitable for improving available P. Moreover, the activities of soil enzymes were markedly enhanced by the cooperative application of oyster shell and biochar. These results suggested that the joint application of biochar and oyster shell is a low-cost pathway to effectively reducing the Cd uptake by crops and improving soil quality.
Data are available upon request to the authors.
The supplement related to this article is available online at: https://doi.org/10.5194/soil-8-409-2022-supplement.
BW led the investigation with MS, HP, and DP, wrote the original draft, and supervised the project. JL assisted with the review and editing of the paper. HP visualized the project, DP curated the data, and HX conceptualized the project, procured resources and acquired the funding.
The contact author has declared that neither they nor their co-authors have any competing interests.
Publisher's note: Copernicus Publications remains neutral with regard to jurisdictional claims in published maps and institutional affiliations.
This study was financially supported by Key Technologies Research and Development Program (CN; grant no. 2018YFC1802605), the Science and Technology Project of Sichuan Province (grant no. 2022YFN0066), Chengdu Science and Technology Project (grant no. 2021-YF05-00195-SN), State Key Laboratory of Geohazard Prevention and Geoenvironment Protection Independent Research Project (grant no. SKLGP2021Z030), and State Environmental Protection Key Laboratory of Synergetic Control and Joint Remediation for Soil and Water Pollution (grant no. GHBK-2020-012). The authors also wish to thank Guanglei Cheng and Hui Wang from Sichuan University, for the technical assistance.
This study has been supported by the Key Technologies Research and Development Program of China (grant no. 2018YFC1802605), Science and Technology Project of Sichuan Province (grant no. 2022YFN0066), Chengdu Science and Technology Project (grant no. 2021-YF05-00195-SN), State Key Laboratory of Geohazard Prevention and Geoenvironment Protection Independent Research Project (grant no. SKLGP2021Z030), and State Environmental Protection Key Laboratory of Synergetic Control and Joint Remediation for Soil & Water Pollution (grant no. GHBK-2020-012).
This paper was edited by Rafael Clemente and reviewed by two anonymous referees.
Abd El-Azeem, S. A. M., Ahmad, M., Usman, A. R. A., Kim, K.-R., Oh, S.-E., Lee, S. S., and Ok, Y. S.: Changes of biochemical properties and heavy metal bioavailability in soil treated with natural liming materials, Environ. Earth. Sci., 70, 3411–3420, https://doi.org/10.1007/s12665-013-2410-3, 2013.
Ameloot, N., Sleutel, S., Case, S., Alberti, G., Mcnamara, N. P., Zavalloni, C., Vervisch, B., Vedove, G. D., and Neve, S. D.: C mineralization and microbial activity in four biochar field experiments several years after incorporation, Soil Biol. Biochem., 78, 195–203, https://doi.org/10.1016/j.soilbio.2014.08.004, 2014.
Benefield, C. B., Howard, P. J. A., and Howard, D. M.: The estimation of dehydrogenase activity in soil, Soil Biol. Biochem., 9, 67–70, https://doi.org/10.1016/0038-0717(77)90063-3, 1977.
Bolan, N., Kunhikrishnan, A., Thangarajan, R., Kumpiene, J., Park, J., Makino, T., Kirkham, M. B., and Scheckel, K.: Remediation of heavy metal(loid)s contaminated soils – To mobilize or to immobilize?, J. Hazard. Mater., 266, 141–166, https://doi.org/10.1016/j.jhazmat.2013.12.018, 2014.
Campos, J. A., Peco, J. D., and García-Noguero, E.: Antigerminative comparison between naturally occurring naphthoquinones and commercial pesticides. Soil dehydrogenase activity used as bioindicator to test soil toxicity, Sci. Total. Environ., 694, 133672, https://doi.org/10.1016/j.scitotenv.2019.133672, 2019.
Chen, H., Yang, X., Wang, P., Wang, Z., Li, M., and Zhao, F.-J.: Dietary cadmium intake from rice and vegetables and potential health risk: A case study in Xiangtan, southern China, Sci. Total. Environ., 639, 271–277, https://doi.org/10.1016/j.scitotenv.2018.05.050, 2018.
Dean, L. A.: Fixation of Soil Phosphorus, Adv. Agron., 1, 391–411, https://doi.org/10.1016/S0065-2113(08)60754-3, 1949.
Feng, L., Yan, H., Dai, C., Xu, W., Gu, F., Zhang, F., Li, T., Xian, J., He, X., Yu, Y., Ma, M., Wang, F., and He, Z.: The systematic exploration of cadmium-accumulation characteristics of maize kernel in acidic soil with different pollution levels in China, Sci. Total. Environ., 729, 138972, https://doi.org/10.1016/j.scitotenv.2020.138972, 2020.
Giagnoni, L., Maienza, A., Baronti, S., Vaccari, F. P., Genesio, L., Taiti, C., Martellini, T., Scodellini, R., Cincinelli, A., Costa, C., Mancuso, S., and Renella, G.: Long-term soil biological fertility, volatile organic compounds and chemical properties in a vineyard soil after biochar amendment, Geoderma, 344, 127–136, https://doi.org/10.1016/j.geoderma.2019.03.011, 2019.
Halim, C. E., Amal, R., Beydoun, D., Scott, J. A., and Low, G: Evaluating the applicability of a modified toxicity characteristic leaching procedure (TCLP) for the classification of cementitious wastes containing lead and cadmium, J. Hazard. Mater., 103, 125–140, https://doi.org/10.1016/S0304-3894(03)00245-0, 2003.
He, L., Zhong, H., Liu, G., Dai, Z., Brookes, P. C., and Xu, J.: Remediation of heavy metal contaminated soils by biochar: Mechanisms, potential risks and applications in China, Environ. Pollut., 252, 846–855, https://doi.org/10.1016/j.envpol.2019.05.151, 2019.
Huang, S., Rao, G., Ashraf, U., He, L., Zhang, Z., Zhang, H., Mo, Z., Pan, S., and Tang, X.: Application of inorganic passivators reduced Cd contents in brown rice in oilseed rape-rice rotation under Cd contaminated soil, Chemosphere, 259, 127404, https://doi.org/10.1016/j.chemosphere.2020.127404, 2020.
Jing, F., Chen, C., Chen, X., Liu, W., Wen, X., Hu, S., Yang, Z., Guo, B., Xu, Y., and Yu, Q.: Effects of wheat straw derived biochar on cadmium availability in a paddy soil and its accumulation in rice, Environ. Pollut., 257, 113592, https://doi.org/10.1016/j.envpol.2019.113592, 2020.
Kumpiene, J., Lagerkvist, A., and Maurice, C.: Stabilization of As, Cr, Cu, Pb and Zn in soil using amendments – A review, Waste Manag., 28, 215–225, https://doi.org/10.1016/j.wasman.2006.12.012, 2008.
Lebrun, J. D., Trinsoutrot-Gattin, I., Vinceslas-Akpa, M., Bailleul, C., Brault, A., Mougin, C., and Laval, K.: Assessing impacts of copper on soil enzyme activities in regard to their natural spatiotemporal variation under long-term different land uses, Soil Biol. Biochem., 49, 150–156, https://doi.org/10.1016/j.soilbio.2012.02.027, 2012.
Lee, C. H., Lee, D. K., Ali, M. A., and Kim, P. J.: Effects of oyster shell on soil chemical and biological properties and cabbage productivity as a liming materials, Waste Manag, 28, 2702–2708, https://doi.org/10.1016/j.wasman.2007.12.005, 2008.
Lian, W., Li, H., Yang, J., Joseph, S., Bian, R., Liu, X., Zheng, J., Drosos, M., Zhang, X., Li, L., Shan, S., and Pan, G.: Influence of pyrolysis temperature on the cadmium and lead removal behavior of biochar derived from oyster shell waste, Bioresource Technology Reports, 15, 100709, https://doi.org/10.1016/j.biteb.2021.100709, 2021.
Liao, N., Li, Q., Zhang, W., Zhou, G. W., Ma, L. J., Min, W., Ye, J., and Hou, Z. N.: Effects of biochar on soil microbial community composition and activity in drip-irrigated desert soil, Eur. J. Soil Biol., 72, 27–34, https://doi.org/10.1016/j.ejsobi.2015.12.008, 2016.
Lin, H., Liu, C., Li, B., and Dong, Y.: Trifolium repens L. regulated phytoremediation of heavy metal contaminated soil by promoting soil enzyme activities and beneficial rhizosphere associated microorganisms, J. Hazard. Mater., 402, 123829, https://doi.org/10.1016/j.jhazmat.2020.123829, 2021.
Liu, C., Lin, H., Li, B., Dong, Y., Yin, T., and Chen, X.: Endophyte inoculation redistributed bioavailable Cd and nutrient in soil aggregates and enhanced Cd accumulation in Phytolacca acinosa, J. Hazard. Mater., 416, 125952, https://doi.org/10.1016/j.jhazmat.2021.125952, 2021.
Liu, H.-B., Gou, Y., Wang, H.-Y., Li, H.-M., and Wu, W.: Temporal changes in climatic variables and their impact on crop yields in southwestern China, Int. J. Biometeorol., 58, 1021–1030, https://doi.org/10.1007/s00484-013-0686-3, 2014.
Liu, H., Xu, F., Xie, Y., Wang, C., Zhang, A., Li, L., and Xu, H.: Effect of modified coconut shell biochar on availability of heavy metals and biochemical characteristics of soil in multiple heavy metals contaminated soil, Sci. Total. Environ., 645, 702–709, https://doi.org/10.1016/j.scitotenv.2018.07.115, 2018.
Li, Y., Huang, P., Guo, S., and Nie, M.: A promising and green strategy for recycling waste oyster shell powder as bio-filler in polypropylene via mycelium-enlightened interfacial interlocking, J. Clean. Prod., 272, 122694, https://doi.org/10.1016/j.jclepro.2020.122694, 2020.
Lu, H., Li, Z., Fu, S., Méndez, A., Gascó, G., and Paz-Ferreiro, J.: Combining phytoextraction and biochar addition improves soil biochemical properties in a soil contaminated with Cd, Chemosphere, 119, 209–216, https://doi.org/10.1016/j.chemosphere.2014.06.024, 2015.
Lu, M., Shi, X., Feng, Q., Li, X., Lian, S., Zhang, M., and Guo, R.: Effects of humic acid modified oyster shell addition on lignocellulose degradation and nitrogen transformation during digestate composting, Bioresource Technol., 329, 124834, https://doi.org/10.1016/j.biortech.2021.124834, 2021.
Ma, L., Liu, Y., Wu, Y., Wang, Q., Sahito, Z. A., Zhou, Q., Huang, L., Li, T., and Feng, Y.: The effects and health risk assessment of cauliflower co-cropping with Sedum alfredii in cadmium contaminated vegetable field, Environ. Pollut., 268, 115869, https://doi.org/10.1016/j.envpol.2020.115869, 2021.
Martínez-Iñigo, M. J., Pérez-Sanz, A., Ortiz, I., Alonso, J., Alarcón, R., García, P., and Lobo, M. C.: Bulk soil and rhizosphere bacterial community PCR–DGGE profiles and β-galactosidase activity as indicators of biological quality in soils contaminated by heavy metals and cultivated with Silene vulgaris (Moench) Garcke, Chemosphere, 75, 1376–1381, https://doi.org/10.1016/j.chemosphere.2009.03.014, 2009.
Ma, Y., Ran, D., Shi, X., Zhao, H., and Liu, Z.: Cadmium toxicity: A role in bone cell function and teeth development, Sci. Total. Environ., 769, 144646, https://doi.org/10.1016/j.scitotenv.2020.144646, 2021.
Mehdizadeh, L., Farsaraei, S., and Moghaddam, M.: Biochar application modified growth and physiological parameters of Ocimum ciliatum L. and reduced human risk assessment under cadmium stress, J. Hazard. Mater., 409, 124954, https://doi.org/10.1016/j.jhazmat.2020.124954, 2021.
Mou, H., Chen, W., Xue, Z., Li, Y., Ao, T., and Sun, H.: Effect of irrigation water system's distribution on rice cadmium accumulation in large mild cadmium contaminated paddy field areas of Southwest China, Sci. Total. Environ., 746, 141248, https://doi.org/10.1016/j.scitotenv.2020.141248, 2020.
Ok, Y. S., Oh, S.-E., Ahmad, M., Hyun, S., Kim, K.-R., Moon, D. H., Lee, S. S., Lim, K. J., Jeon, W.-T., and Yang, J. E.: Effects of natural and calcined oyster shells on Cd and Pb immobilization in contaminated soils, Environ. Earth. Sci., 61, 1301–1308, https://doi.org/10.1007/s12665-010-0674-4, 2010.
Palansooriya, K. N., Shaheen, S. M., Chen, S. S., Tsang, D. C. W., Hashimoto, Y., Hou, D. Y., Bolan, N. S., Rinklebe, J., and Ok, Y. S.: Soil amendments for immobilization of potentially toxic elements in contaminated soils: A critical review, Environ Int, 134, 105046, https://doi.org/10.1016/j.envint.2019.105046, 2020.
Shi, J., Du, P., Luo, H., Wu, H., Zhang, Y., Chen, J., Wu, M., Xu, G., and Gao, H.: Soil contamination with cadmium and potential risk around various mines in China during 2000–2020, J. Environ. Manage., 310, 114509, https://doi.org/10.1016/j.jenvman.2022.114509, 2022.
Tang, X., Shen, H., Chen, M., Yang, X., Yang, D., Wang, F., Chen, Z., Liu, X., Wang, H., and Xu, J.: Achieving the safe use of Cd- and As-contaminated agricultural land with an Fe-based biochar: A field study, Sci. Total Environ., 706, 135898, https://doi.org/10.1016/j.scitotenv.2019.135898, 2020.
van Aarle, I. M. and Plassard, C.: Spatial distribution of phosphatase activity associated with ectomycorrhizal plants is related to soil type, Soil Biol. Biochem., 42, 324–330, https://doi.org/10.1016/j.soilbio.2009.11.011, 2010.
Walz, J., Knoblauch, C., Boehme, L., and Pfeiffer, E.-M.: Regulation of soil organic matter decomposition in permafrost-affected Siberian tundra soils – Impact of oxygen availability, freezing and thawing, temperature, and labile organic matter, Soil Biol. Biochem., 110, 34–43, https://doi.org/10.1016/j.soilbio.2017.03.001, 2017.
Wang, J., Shi, L., Zhai, L., Zhang, H., Wang, S., Zou, J., Shen, Z., Lian, C., and Chen, Y.: Analysis of the long-term effectiveness of biochar immobilization remediation on heavy metal contaminated soil and the potential environmental factors weakening the remediation effect: A review, Ecotox. Eviron. Safe., 207, 111261, https://doi.org/10.1016/j.ecoenv.2020.111261, 2021.
Wei, R., Wang, X., Tang, W., Yang, Y., Gao, Y., Zhong, H., and Yang, L.: Bioaccumulations and potential human health risks assessment of heavy metals in ppk-expressing transgenic rice, Sci. Total Environ., 710, 136496, https://doi.org/10.1016/j.scitotenv.2020.136496, 2020.
Wen, E., Yang, X., Chen, H., Shaheen, S. M., Sarkar, B., Xu, S., Song, H., Liang, Y., Rinklebe, J., Hou, D., Li, Y., Wu, F., Pohořelý, M., Wong, J. W. C., and Wang, H.: Iron-modified biochar and water management regime-induced changes in plant growth, enzyme activities, and phytoavailability of arsenic, cadmium and lead in a paddy soil, J. Hazard. Mater., 407, 124344, https://doi.org/10.1016/j.jhazmat.2020.124344, 2021.
Wu, B., Hou, S., Peng, D., Wang, Y., Wang, C., Xu, F., and Xu, H.: Response of soil micro-ecology to different levels of cadmium in alkaline soil, Ecotoxicol Environ Saf, 166, 116–122, https://doi.org/10.1016/j.ecoenv.2018.09.076, 2018.
Wu, B., Wang, Z., Zhao, Y., Gu, Y., Wang, Y., Yu, J., and Xu, H.: The performance of biochar-microbe multiple biochemical material on bioremediation and soil micro-ecology in the cadmium aged soil, Sci. Total Environ., 686, 719–728, https://doi.org/10.1016/j.scitotenv.2019.06.041, 2019a.
Wu, B., Wang, Z., Zhao, Y., Gu, Y., Wang, Y., Yu, J., and Xu, H.: The performance of biochar-microbe multiple biochemical material on bioremediation and soil micro-ecology in the cadmium aged soil, Sci. Total Environ., 686, 719–728, https://doi.org/10.1016/j.scitotenv.2019.06.041, 2019b.
Xu, M., Zhao, Z., Song, Y., Li, J., You, Y., and Li, J.: Evaluation of ferrihydrite-humic acid coprecipitate as amendment to remediate a Cd- and Pb-contaminated soil, Geoderma, 361, 114131, https://doi.org/10.1016/j.geoderma.2019.114131, 2020.
Yan, J., Quan, G., and Ding, C.: Effects of the Combined Pollution of Lead and Cadmium on Soil Urease Activity and Nitrification, Procedia Environ. Sci., 18, 78–83, https://doi.org/10.1016/j.proenv.2013.04.011, 2013.
Yang, Y., Li, Y., Dai, Y., Wang, M., Chen, W., and Wang, T.: Historical and future trends of cadmium in rice soils deduced from long-term regional investigation and probabilistic modeling, J. Hazard. Mater., 415, 125746, https://doi.org/10.1016/j.jhazmat.2021.125746, 2021.
Yin, Z., Sheng, H., Xiao, H., Xue, Y., Man, Z., Huang, D., and Zhou, Q.: Inter-annual reduction in rice Cd and its eco-environmental controls in 6-year biannual mineral amendment in subtropical double-rice cropping ecosystems, Environ. Pollut., 293, 118566, https://doi.org/10.1016/j.envpol.2021.118566, 2022.
Zhang, C., Liu, G., Xue, S., and Song, Z.: Rhizosphere soil microbial activity under different vegetation types on the Loess Plateau, China, Geoderma, 161, 115–125, https://doi.org/10.1016/j.geoderma.2010.12.003, 2011.
Zhang, M., Zhang, L., Riaz, M., Xia, H., and Jiang, C.: Biochar amendment improved fruit quality and soil properties and microbial communities at different depths in citrus production, J. Clean. Prod., 292, 126062, https://doi.org/10.1016/j.jclepro.2021.126062, 2021.
Zhao, X.-L. and Masaihiko, S.: Amelioration of Cadmium Polluted Paddy Soils by Porous Hydrated Calcium Silicate, Water, Air, Soil Poll., 183, 309–315, https://doi.org/10.1007/s11270-007-9379-z, 2007.
Zong, Y., Xiao, Q., and Lu, S.: Biochar derived from cadmium-contaminated rice straw at various pyrolysis temperatures: Cadmium immobilization mechanisms and environmental implication, Bioresource Techno., 321, 124459, https://doi.org/10.1016/j.biortech.2020.124459, 2021.