the Creative Commons Attribution 4.0 License.
the Creative Commons Attribution 4.0 License.
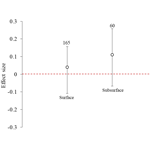
Effects of environmental factors on the influence of tillage conversion on saturated soil hydraulic conductivity obtained with different methodologies: a global meta-analysis
Kaihua Liao
Juan Feng
Xiaoming Lai
Qing Zhu
The saturated hydraulic conductivity (Ksat) is a key soil hydraulic property governing agricultural production. However, the influence of the conversion from the conventional tillage (CT) to conservation tillage (CS; including no tillage, NT, and reduced tillage, RT) on the Ksat of soils is not well understood and still debated. In this study, we applied a global meta-analysis method to synthesize 227 paired observations for soil Ksat from 69 published studies and investigated factors influencing the effects of conversion to CS on Ksat. Results showed that soil layer, conservation tillage type, soil texture type, and cropping system management did not have significant effects on the influence of conversion to CS on Ksat. When the Ksat was measured by the rainfall simulator, the conversion to CS significantly (p<0.05) increased the surface and subsurface soil Ksat by 41.7 % and 36.9 %, respectively. In addition, the subsurface Ksat also tended to increase under CS practices when the Ksat was measured by a tension disc infiltrometer. However, when the Ksat was measured by a hood infiltrometer, ring infiltrometer, constant/falling head, and Guelph permeameter, the conversion to CS had no significant effects on the Ksat. It is observed that, when the conversion period was less than 15 years, the Ksat under CS showed a greater increase for a longer conversion period. Climatic and topographic factors, including the mean annual temperature (MAT) and the mean annual precipitation (MAP), were statistically related to the responses of Ksat to tillage conversion at the global scale. Quadratic polynomials can describe the relationships between them. These findings suggested that quantifying the effects of tillage conversion on soil Ksat needed to consider experimental conditions, especially the measurement technique and conversion period.
- Article
(1969 KB) - Full-text XML
-
Supplement
(582 KB) - BibTeX
- EndNote
The saturated hydraulic conductivity (Ksat), which reflects soil permeability when the soil is saturated, is critical for calculating water flux in the soil profile and designing irrigation and drainage systems (Bormann and Klaassen, 2008). It is also an essential soil parameter in agro-ecological, hydrological, and biogeochemical models across different scales. The Ksat changes greatly in space and time due to factors such as texture, organic matter content, bulk density, porosity, vegetation types, or tillage practices (Schaap et al., 1998; Zhu et al., 2014; Liao et al., 2018; Schlüter et al., 2020). Infiltration experiments are often applied to measure the infiltration rate of soils in field by different techniques, such as a hood infiltrometer (Schwärzel and Punzel, 2007), tension disc infiltrometer (Perroux and White, 1988), and single- or double-ring infiltrometer (Bouwer, 1986). Permeameters are also adopted to measure Ksat, such as the Guelph permeameter (Reynolds and Elrick, 1985) used in fields and the constant/falling head permeameter applied on intact (undisturbed) or repacked soil cores (Klute and Dirksen, 1986). In addition, rainfall simulators have been applied to simulate rainfall events for the infiltration runs (Gupta et al., 1994).
Tillage is one of the main causes of spatiotemporal variability in Ksat. Conventional tillage (CT), mainly referred to as heavy tillage practices down to 25–30 cm soil depths, is a widely adopted management practice which could significantly affect soil aggregation and hydraulic properties (Pittelkow et al., 2014; Y. Li et al., 2019). Conservation tillage (CS) is often defined as no tillage (NT) or reduced tillage (RT), with/without residue retention. NT is confined to soil disturbance associated with crop seeding or planting, while in RT a cultivator or disc harrow is used to loosen the soil superficially (Licht and Al-Kaisi, 2005). The CS practices directly affect soil physical properties by increasing the residue retention and decreasing soil disturbance (Turmel et al., 2015). The conversion from CT to CS has been demonstrated to improve the physical environment of the soil (Y. Li et al., 2019). In a wheat/soybean–corn rotation field in the Argentinian Pampas, Sasal et al. (2006) found that aggregates of silty cultivated soils were 30 % more stable in CS than under CT due to 21 % increase in organic matter. Based on long-term wheat–fallow tillage experiments, Blanco-Canqui et al. (2009) observed that the near-surface soil maximum bulk density of the CT was higher than that of the NT soil by about 6 % at Akron, Hays, and Tribune in the central Great Plains. However, it is still controversial whether the change from CT to CS can increase Ksat. Several studies (Jarecki and Lal, 2005; Abid and Lal, 2009; Nouri et al., 2018) have reported systematic improvements in the Ksat under CS practices, which may be attributed to the decomposition of aggregates, the formation of surface seal by the raindrop impact, the increase in compactness, and the decrease in the average pore size distribution of topsoil under CT. In contrast, pores in CS soil may be well connected and protected from raindrop impact and other disturbances by residual mulch (Blanco-Canqui and Lal, 2007; Shukla et al., 2003). However, other studies have shown that Ksat under CS is not higher than that under CT (Anikwe and Ubochi, 2007; Abu and Abubakar, 2013; Busari, 2017). Tillage conversion may also lead to different degrees of changes in the factors (e.g. soil structure, organic matter content, and bulk density) influencing Ksat (Cameira et al., 2003). There, the response of Ksat to tillage was complex and not well understood. In addition to CS practices, there are many other agricultural practices that may increase Ksat, such as compost addition, straw returning, and biochar returning (Olson et al., 2013; Xiao et al., 2020). However, addressing these agricultural practices is beyond the scope of this study.
The effects of tillage on Ksat may partly depend on measurement techniques (Morbidelli et al., 2017). The Ksat measured by different measurement techniques may differ by an order of magnitude, which is mainly due to the following reasons: (1) the geometry of water application to the soil is different, (2) the strategies to prevent surface sealing and pore plugging are different, (3) the soil wetted (or saturated) volume is different, and (4), for laboratory procedures, the sample size and sampling method may alter the soil core conditions (Fodor et al., 2011; Schlüter et al., 2020). The uncertainty of measurement techniques can mask the influence of the conversion from CT to CS on Ksat. Soil layer, texture, and CS type may also influence the tillage effect on Ksat (Alletto et al., 2010). For example, Yu et al. (2015) observed that the tillage of cropland created temporarily well-structured topsoil but compacted the subsoil, as indicated by low subsoil Ksat. Soil texture is one of the main factors controlling soil infiltration and hydraulic conductivity. Coarse-textured soils lose moisture much more easily than fine-textured soils because of the weaker capillary forces in the large pore spaces. CS has direct and indirect effects on the soil structure. Generally, soil compaction begins with the conversion to CS, which may lead to a decrease in air capacity and increase in the bulk density and permeability resistance of surface soil (Abdollahi and Munkholm, 2017). In addition, climatic and topographic factors were also found to be related to Ksat. For instance, Jarvis et al. (2013) proposed that climatic factors can affect Ksat through the effects of soil moisture on soil biota and plant growth and thus the abundance of root and faunal biopores. Yang et al. (2018) found that elevation and soil properties dominated the Ksat spatial distribution in the Loess Plateau of China. Previous studies have related the response of Ksat to tillage and environmental conditions (Strudley et al., 2008; Bodner et al., 2013). However, there has not yet been a global synthetic analysis specifically focusing on how environmental conditions could affect the tillage effect on Ksat. Recently, Y. Li et al. (2019) applied a global meta-analysis to investigate the direction and magnitude of changes in Ksat in response to CS practices. They found that CS practices improved Ksat in croplands compared with CT. However, the generalizable patterns and regulating factors of tillage effects on Ksat remain unclear at the global scale. Therefore, it is necessary to synthesize all available data to reveal the global-scale response of Ksat and to identify the main regulating factors for its response under CS practices.
The objective of this study was to detect the influences of different experimental conditions (i.e. measurement technique, soil layer, texture, CS type, conversion period, cropping system management, mean annual precipitation (MAP), mean annual temperature (MAT), and elevation) on the effects of conversion from CT to CS on the Ksat, based on a global meta-analysis of 65 studies. We specifically hypothesized that conversion to CS can increase the soil Ksat measured by a ring infiltrometer and rainfall simulator.
2.1 Source of data and selection criteria
Peer-reviewed journal articles and dissertations related to Ksat under CT and CS were searched using the Web of Science and China National Knowledge Infrastructure (CNKI; http://www.cnki.net, last access: 22 January 2022). The keywords used for the literature search were related to “saturated hydraulic conductivity”, “steady-state infiltration rate”, “conventional tillage”, “conservation tillage”, and “till”. Using these keywords, a total of 128 papers were searched. To minimize bias, our criteria were as follows: (1) the selected articles included paired observations comparing CT and CS based on field experiments; (2) specific CS practices included RT and NT; (3) other agronomic measures, such as residue retention and film mulching, must be similar between paired controls (CT) and treatments (CS) during the selection process; (4) means, standard deviations (SD; or standard errors, SE), and sample sizes were directly provided or could be calculated from the studies; (5) if one article contained Ksat in multiple years, then only the latest results were applied since the observations should be independent in the meta-analysis (Hedges et al., 1999); and (6) for the ring infiltrometer, the diameter of a single ring, or the diameter of the inner ring of a double ring, should be greater than 50 cm in this study, although inner and outer ring diameters of about 30 and 60 cm have been widely applied to measure the soil infiltration process (e.g. Ronayne et al., 2012; Zhang et al., 2017). A recent study (M. Li et al., 2019) has demonstrated that the ring infiltrometer with an inner diameter of 40 cm is not enough to completely overcome the scale effect. (7) For the Guelph permeameter, only the one-head technique was considered for meta-analysis. Previous studies (Reynolds and Elrick, 1985; Jabro and Evans, 2006) have shown that, for a significant percentage of times, the two-head method produced unreliable results when using Guelph permeameter. In total, 69 published studies conducted around the world were selected from 128 published articles (Fig. 1). The locations of these studies and their site information are presented in Tables S1 and S2 in the Supplement.
Of the 69 studies, 15 did not provide Ksat values but rather steady-state infiltration rate values. The Ksat refers to flow through a saturated porous medium, and the infiltration rate represents the imbibition of water from free water above the soil to pore water beneath the soil surface. In this case, there are interface issues such as surface tension, surface crust and seal effects, the influence of litter, mulch, and other factors. Nevertheless, the steady-state infiltration rate was assumed to be the Ksat by convention in this study (Yolcubal et al., 2004; Kirkham, 2014; Table S2). A total of six measurement techniques for infiltration rate and Ksat were involved in these 65 studies, including the hood infiltrometer, tension disc infiltrometer, ring infiltrometer, rainfall simulator, Guelph permeameter used in the field, and constant/falling head applied on undisturbed soil cores. The first four techniques determined the infiltration rate based on the water entry into an unsaturated soil at the soil–atmosphere boundary, while the last two measured the flow of water from one point to another within the soil mass. The final infiltration rate measured by a single or double ring infiltrometer and by tension and hood infiltrometer methods at zero tension were often equated to Ksat of the soil. In the selected literature, the infiltration rate has been converted to Ksat for the first four techniques.
2.2 Data extraction and statistical analysis
For each study, the mean, the standard error (SE), or standard deviation (SD), and sample size values for treatment and control groups were extracted for Ksat. The units of Ksat for all studies were converted to centimetres per day (cm d−1). For studies that did not provide SD or SE, SD was predicted as 0.1 times the mean (Y. Li et al., 2019). In addition to Ksat, the measurement technique of Ksat, soil depth, texture, CS type, conversion period (time since the conversion), cropping system management, MAP, MAT, and elevation were also recorded if they could be obtained. All data were extracted from words or tables or digitized from graphs with the software GetData v2.2.4 (http://www.getdata-graph-digitizer.com, last access: 7 December 2021).
The MetaWin 2.1 software (Sinauer Associates Inc., Sunderland, MA, USA; Rosenberg et al., 2000) was used to perform the meta-analysis in this study. The natural logarithm of the response ratio (R) was used to estimate the effects of changes in tillage practices on Ksat as follows (Hedges et al., 1999):
where and are the mean value of Ksat under CS (treatment) and CT practices (control), respectively. The natural log was applied for meta-analysis, since its bias is relatively small, and its sampling distribution is approximately normal (Luo et al., 2006). In addition, the variance (VAR) of ln(R) was calculated as follows:
where ns and nt are the sample sizes for the CS and CT practices, respectively, and Ss and St are the SDs for CS and CT practices, respectively. To examine whether the experimental conditions alter the response direction and magnitude of Ksat, observations were divided into subgroups according to the measurement techniques (hood infiltrometer, tension disc infiltrometer, Guelph permeameter, ring infiltrometer, rainfall simulator used in the field, and constant/falling head used on undisturbed soil cores), soil layer (surface (0–20 cm) and subsurface (>20 cm depth)), CS practices (NT and RT), soil texture (fine-, medium-, and coarse-textured soil), conversion period (1–5, 6–10, 11–15, 16–20, 21–30, and >30 years), and cropping system management (single cropping and crop rotation). To differentiate among soil textural classes, we applied the United States Department of Agriculture (USDA) soil textural triangle and considered clay, sandy clay, and silty clay soils as having a fine texture, silt, silt loam, silty clay loam, loam, sandy clay loam, and clay loam soils as having a medium texture, and sand, loamy sand, and sandy loam soils as having a coarse texture (Daryanto et al., 2016).
A random effects model with a grouping variable was used to compare the responses among different subgroups. In this model, there are two sources of variance, including the within-study variance (VAR) and between-study variance (τ2), both of which were used to calculate the weighting factor , with , where Q is the observed weighted sum of squares, df are the degrees of freedom, and C is a normalization factor. The calculation equations of Q, df, and C can be found in Borenstein et al. (2010). The weighted ln (R) (ln (R∗)), which was used as the effect size, was then determined based on the ω. ln (R∗) is defined as , where ωi and ln (Ri) are ω and ln(R) of the ith observation, respectively. The ln (R∗) value indicated the magnitude of the treatment impact. Positive or negative ln (R∗) values represented an increase or decrease effect of the tillage treatment, respectively. Zero meant no difference between treatment (CS) and control (CT) group. Finally, resampling tests were incorporated into our meta-analysis using the bootstrap method (999 random replicates). The mean effect size (; calculated from 999 iterations) and 95 % bootstrap confidence intervals (CIs) were generated. If the 95 % CI values of ln (R∗) did not overlap zero, then the effect of the changes in tillage practices on Ksat were considered significant at p<0.05. The percentage change between CS and CT was calculated as .
Regression analyses were performed by SPSS software (version 13.0; SPSS Inc., Chicago, Illinois, USA) to evaluate the relationships between the ln (R) for soil Ksat under CS with MAP, MAT, and elevation.
The mean effect sizes of Ksat under CS conversion were 0.040 (95 % CI, from −0.108 to 0.156) and 0.110 (95 % CI, from −0.068 to 0.259) for surface and subsurface layers, respectively (Fig. 2). For surface soil Ksat, the mean effect sizes under CS conversion were 0.102 (95 % CI, from −0.422 to 0.415), −0.002 (95 % CI, from −0.087 to 0.069), 0.114 (95 % CI, from −0.213 to 0.412), −0.106 (95 % CI, from −0.402 to 0.159), 0.046 (95 % CI, from −0.187 to 0.269), and 0.348 (95 % CI, from 0.142 to 0.558) for hood infiltrometer, tension disc infiltrometer, ring infiltrometer, constant/falling head, Guelph permeameter, and rainfall simulator, respectively (Fig. 3a). However, the mean effect sizes of subsurface Ksat under CS conversion were 0.623 (95 % CI, from 0.164 to 0.997), 0.036 (95 % CI, from −0.161 to 0.231), 0.213 (95 % CI, from −0.028 to 0.486), and 0.314 (95 % CI, from 0.062 to 0.566) for the tension disc infiltrometer, constant/falling head, Guelph permeameter, and rainfall simulator, respectively (Fig. 3b).
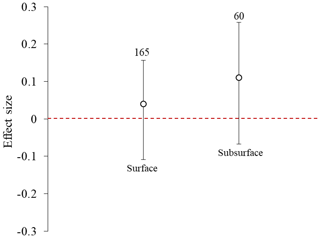
Figure 2Influence of the soil layer on the effect sizes of the soil saturated hydraulic conductivity under conservation tillage (CS) from a global meta-analysis of 69 studies. The error bars indicate the effect sizes and 95 % bootstrap confidence intervals (CIs). The effect of CS was statistically significant if the 95 % CI did not bracket zero. The sample size for each variable is shown next to the bar.
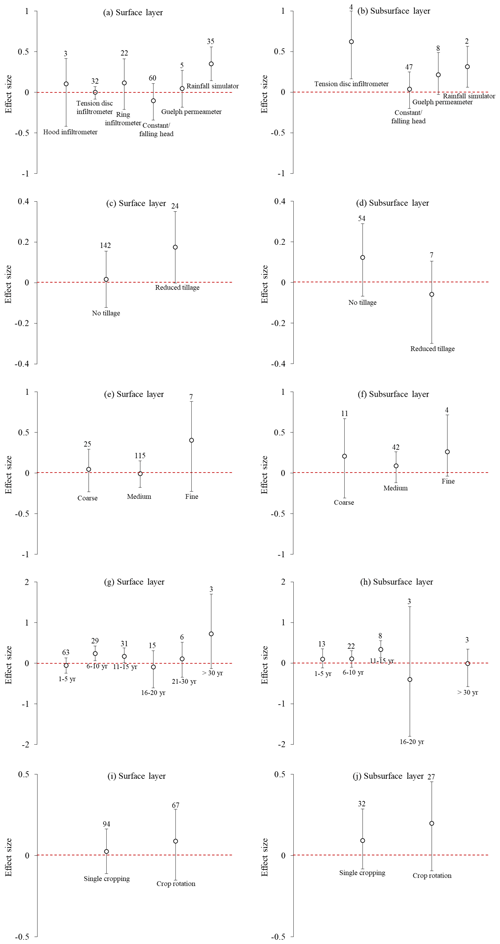
Figure 3Factors influencing the effect sizes of the surface and subsurface saturated hydraulic conductivity under conservation tillage (CS) from a global meta-analysis of 69 studies, including the (a, b) measurement technique, (c, d) conservation tillage type, (e, f) soil texture type, (g, h) time since conversion, and (i, j) cropping system management. The error bars indicate effect sizes and 95 % bootstrap confidence intervals (CIs). The effect of CS was statistically significant if the 95 % CI did not bracket zero. The sample size for each variable is shown next to the bar.
The CS type, soil texture, and cropping system management had no significant (p>0.05) influences on the effect of the conversion to CS on Ksat, either in the surface layer or the subsurface layer (Fig. 3c–f, i, and j). In addition, the mean effect sizes of surface Ksat under CS were −0.057 (95 % CI, from −0.248 to 0.127), 0.239 (95 % CI, from 0.056 to 0.419), 0.168 (95 % CI, from 0.002 to 0.377), −0.097 (95 % CI, from −0.608 to 0.302), 0.106 (95 % CI, from −0.352 to 0.517), and 0.723 (95 % CI, from −0.130 to 1.699) for the conversion periods of 1–5, 6–10, 11–15, 16–20, 21–30 and >30 years, respectively (Fig. 3g), while those of subsurface Ksat under CS conversion were 0.097 (95 % CI, from −0.120 to 0.354), 0.109 (95 % CI, from −0.102 to 0.306), 0.339 (95 % CI, from 0.138 to 0.550), −0.399 (95 % CI, from −1.802 to 1.387), and −0.009 (95 % CI, from −0.580 to 0.343) for the conversion periods of 1–5, 6–10, 11–15, 16–20 and > 30 years, respectively (Fig. 3h).
The relationships between the ln (R) of Ksat and MAT, MAP, and elevation can be fitted by quadratic polynomials, with the R2 values ranging between 0.005 and 0.099 (Fig. 4).
The change in Ksat caused by the conversion from CT to CS varied between the different measurement techniques employed (Fig. 3a and b). Our findings implied that the measurement technique had an important influence on the determination of Ksat (Reynolds et al., 2000; Rienzner and Gandolfi, 2014). When the Ksat was measured by the rainfall simulator, conversion to CS significantly (p<0.05) increased the surface and subsurface soil Ksat by 41.7 % and 36.9 %, respectively. This is consistent with the findings of previous studies. For instance, Singh et al. (1994) observed that rainfall can reduce surface roughness, especially the first rains after tillage due to the breakdown and sloughing of soil clods upon wetting during rainstorms. Lampurlanés and Cantero-Martínez (2006) proposed that, if a rainfall simulator had been used, then greater infiltration rates would probably have been found on NT because residues play a role similar to that of surface roughness, i.e. increasing the time for infiltration to take place. However, Gupta et al. (1997) found the lower Ksat values of soil measured by rainfall simulator in NT plots compared with those in CT plots, which was attributed to the fact that the NT practice allowed a consolidated layer to form. This was relatively impervious to the infiltrating water on the soil surface. The restricted downward movement of rain water produced lower Ksat under NT. Therefore, more data are needed to test the effect of conversion to CS on Ksat measured by rainfall simulator in the future. In addition, the subsurface Ksat measured by tension disc infiltrometer also tended to increase under CS practices. The possible reason is that the tension disc infiltrometer had a deep water infiltration depth and big infiltration area. Sasal et al. (2006) observed that, when using a tension disc infiltrometer, water entry into the soil profile under NT was mainly conditioned by pore orientation. However, when the Ksat was measured by hood infiltrometer, ring infiltrometer, constant/falling head, and Guelph permeameter, conversion to CS had no significant effects on the surface and subsurface Ksat.
It is noted that since studies comparing tillage conversion effects on Ksat using different methodologies are from different places, maybe there are other reasons that explain the differences found. For example, the study of Lozano et al. (2016) from the Argentinean Pampas region did not include the ring infiltrometer, hood infiltrometer, and rainfall simulator; maybe, in those soils, the results are not only affected by the measurement technique, MAT and MAP, but also by the clay type or other factors. Some cold weather soils present freezing–thawing processes that are important for pore generation.
The CS type, soil texture, and cropping system management had weak effects on the influence of tillage conversion on Ksat, suggesting that the single factor of CS, texture, or cropping system type could not explain the variations in Ksat under CS practices well. However, our results showed that the conversion period substantially affected the influence of conversion to CS on Ksat. Tillage conversion tended to decrease the surface Ksat for the conversion period of 1–5 years. The possible reason is that soil compaction under CS can lead to a reduction in macroporosity and an increase in bulk density and microporosity. Many previous studies have demonstrated the negative relationship between bulk density and Ksat (e.g. Vereecken et al., 1989; Huang et al., 2021). In this case, initially bulk density increased, while Ksat decreased. However, after several years, this reversed through a re-structuring of the soil by bioturbation (Schlüter et al., 2020). As can be seen from Fig. 3g and h, the Ksat under CS showed a greater increase for a longer conversion period when the conversion period was less than 15 years. It is noted that, when the conversion period exceeded 15 years, the improvement in the Ksat under CS is not significant. The reason may be that the decreased soil disturbance with long-term CS practices can increase soil bulk density over time, which can lead to lower water infiltration rate (Six et al., 2000; Y. Li et al., 2019).
The response of surface Ksat was generally negatively correlated with MAT and MAP (Fig. 4a and b). This indicated that climatic factors had a potential influence on the response of Ksat to tillage conversion. The possible reason is that climatic factors mainly indirectly control Ksat responses via other variables (e.g. soil moisture, biological processes, and effective porosity; Jarvis et al., 2013). In addition, the correlations between the response of Ksat and elevation were very weak (Fig. 4c). Based on these results, we argue that, in the cold and temperate regions, the improvement of Ksat by tillage conversion will be greater than that in the tropical regions. Although this study provided a global meta-analysis of the responses of Ksat to changes in tillage practices under different experimental conditions, the magnitude of these responses might be uncertain. For example, a relatively small number of observations were obtained with the hood infiltrometer, which would affect the results of the meta-analysis. Nevertheless, this study emphasized the importance of experimental conditions in judging the change in tillage practices for enhancing soil permeability.
Our global meta-analysis indicated that the conversion from CT to CS had no significant effects on surface and subsurface Ksat. However, these effects were related to experimental conditions, especially the measurement technique, conversion period, and climatic factors. The increase in Ksat measured by the rainfall simulator was substantially larger than the other techniques. In addition, the Ksat under CS showed a greater increase for a longer conversion period, when the conversion period was less than 15 years. Moreover, the lower the MAT or MAP, the more obvious the improvement effect of the tillage conversion on surface Ksat. Our findings should be useful for understanding the underlying mechanisms driving the change in soil Ksat with CS practices.
The data that support the findings of this study are available from the corresponding author upon request.
The supplement related to this article is available online at: https://doi.org/10.5194/soil-8-309-2022-supplement.
KL designed this study, KL, JF and XL performed the meta-analysis, KL and QZ obtained the funding, and KL wrote the paper, with contributions from QZ.
The contact author has declared that neither they nor their co-authors have any competing interests.
Publisher's note: Copernicus Publications remains neutral with regard to jurisdictional claims in published maps and institutional affiliations.
We thank the two anonymous reviewers and editor, for their efforts on this paper. Support for this research was provided by the National Natural Science Foundation of China and by the Chinese Academy of Sciences.
This research has been supported by the National Natural Science Foundation of China (grant nos. 42125103 and 42171077) and the Youth Innovation Promotion Association of the Chinese Academy of Sciences (grant no. 2020317).
This paper was edited by David Dunkerley and reviewed by two anonymous referees.
Abid, M. and Lal, R.: Tillage and drainage impact on soil quality: II. Tensile strength of aggregates, moisture retention and water infiltration, Soil Till. Res., 103, 364–372, https://doi.org/10.1016/j.still.2008.11.004, 2009.
Abdollahi, L. and Munkholm, L. J.: Eleven years' effect of conservation practices for temperate sandy loams: II. Soil pore characteristics, Soil Sci. Soc. Am. J., 81, 392–403, https://doi.org/10.2136/sssaj2016.07.0221, 2017.
Abu, S. T. and Abubakar, I. U.: Evaluating the effects of tillage techniques on soil hydro-physical properties in Guinea Savanna of Nigeria, Soil Till. Res., 126, 159–168, https://doi.org/10.1016/j.still.2012.09.003, 2013.
Alletto, L., Coquet, Y., and Roger-Estrade, J.: Two-dimensional spatial variation of soil physical properties in two tillage systems, Soil Use Manage., 26, 432–444, https://doi.org/10.1111/j.1475-2743.2010.00295.x, 2010.
Anikwe, M. A. N. and Ubochi, J. N.: Short-term changes in soil properties under tillage systems and their effect on sweet potato (Ipomea batatas L.) growth and yield in an Ultisol in south-eastern Nigeria, Aust. J. Soil Res., 45, 351–358, https://doi.org/10.1071/SR07035, 2007.
Blanco-Canqui, H. and Lal, R.: Soil and crop response to harvesting corn residues for biofuel production, Geoderma, 141, 355–362, https://doi.org/10.1016/j.geoderma.2007.06.012, 2007.
Blanco-Canqui, H., Stone, L. R., Schlegel, A. J., Lyon., D. J., Vigil, M. F., Mikha, M. M., Stahlman, P. W., and Rice, C. W.: No-till induced increase in organic carbon reduces maximum bulk density of soils, Soil Sci. Soc. Am. J., 73, 1871–1879, https://doi.org/10.2136/sssaj2008.0353, 2009.
Bodner, G., Scholl, P., Loiskandl, W., and Kaul, H.-P.: Environmental and management influences on temporal variability of near saturated soil hydraulic properties, Geoderma, 204–205, 120–129, https://doi.org/10.1016/j.geoderma.2013.04.015, 2013.
Borenstein, M., Hedges, L. V., Higgins, J. P., and Rothstein, H. R.: A basic introduction to fixed-effect and random-effects models for meta-analysis, Res. Synth. Methods, 1, 97–111, https://doi.org/10.1002/jrsm.12, 2010.
Bormann, H. and Klaassen, K.: Seasonal and land use dependent variability of soil hydraulic and soil hydrological properties of two Northern German soils, Geoderma, 145, 295–302, https://doi.org/10.1016/j.geoderma.2008.03.017, 2008.
Bouwer, H.: Intake rate: Cylinder infiltrometer, in: Methods of soil analysis, Part 1, 2nd Edn., edited by: Klute, A., Agron. Monogr., 9, ASA and SSSA, Madison, WI, 825–844, ISBN 978-08-9118-088-3, 1986.
Busari, M. A.: Soil physical properties in relation to maize (Zea mays) yield after tillage and application of organic and inorganic fertilisers in Abeokuta, southwestern Nigeria, Soil Res., 55, 704–714, https://doi.org/10.1071/SR16162, 2017.
Cameira, M. R., Fernando, R. M., and Pereira, L. S.: Soil macropore dynamics affected by tillage and irrigation for a silty loam alluvial soil in southern Portugal, Soil Till. Res., 70, 131–140, https://doi.org/10.1016/S0167-1987(02)00154-X, 2003.
Daryanto, S., Wang, L., and Jacinthe, P. A.: Global synthesis of drought effects on cereal, legume, tuber and root crops production: A review, Agr. Water Manage., 1, 18–33, https://doi.org/10.1016/j.agwat.2016.04.022, 2016.
Fodor, N., Sándor, R., Orfanus, T., Lichner, L., and Rajkai, K.: Evaluation method dependency of measured saturated hydraulic conductivity, Geoderma, 165, 60–68, https://doi.org/10.1016/j.geoderma.2011.07.004, 2011.
Gupta, R. K., Rudra, R. P., Dickinson, W. T., and Wall, G. J.: Spatial and seasonal variations in hydraulic conductivity in relation to four determination techniques, Can. Water Resour. J., 19, 103–113, https://doi.org/10.4296/cwrj1902103, 1994.
Gupta, R. K., Rudra, R. P., Dickinson, W. T., and Wall, G. J.: Surface water quality impacts of tillage practices under liquid swine manure application, J. Am. Water Resour. As., 33, 681–687. 1997.
Hedges, L. V., Gurevitch, J., and Curtis, P. S.: The meta-analysis of response ratios in experimental ecology, Ecology, 80, 1150–1156, 1999.
Huang, J., Jiang, D., Deng, Y., Ding, S., Cai, C., and Huang, Z.: Soil physicochemical properties and fertility evolution of permanent gully during ecological restoration in granite hilly region of South China, Forests, 12, 510, https://doi.org/10.3390/f12040510, 2021.
Jabro, J. D. and Evans, R. G.: Discrepancies between analytical solutions of two borehole permeameters for estimating field-saturated hydraulic conductivity, Appl. Eng. Agric., 22, 549–554, https://doi.org/10.13031/2013.21223, 2006.
Jarecki, M. K. and Lal, R.: Soil organic carbon sequestration rates in two long-term no-till experiments in Ohio, Soil Sci., 170, 280–291, 2005.
Jarvis, N., Koestel, J., Messing, I., Moeys, J., and Lindahl, A.: Influence of soil, land use and climatic factors on the hydraulic conductivity of soil, Hydrol. Earth Syst. Sci., 17, 5185–5195, https://doi.org/10.5194/hess-17-5185-2013, 2013.
Kirkham, M. B.: Principles of Soil and Plant Water Relations, 2nd Edn., Elsevier, Amsterdam, the Netherlands, ISBN 978-0-12-420022-7, 2014.
Klute, A. and Dirksen, C.: Hydraulic conductivity and diffusivity: Laboratory methods, in: Methods of soil analysis. Part 1, 2nd edn., edited by: Klute, A., Agron. Monogr. 9, ASA and SSSA, Madison, WI, 687–734, https://doi.org/10.2136/sssabookser5.1.2ed.c28, 1986.
Lampurlanés, J. and Cantero-Martínez, C.: Hydraulic conductivity, residue cover and soil surface roughness under different tillage systems in semiarid conditions, Soil Till. Res., 85, 13–26, 2006.
Li, M., Liu, T., Duan, L., Luo, Y., Ma, L., Zhang, J., Zhou, Y., and Chen, Z.: The scale effect of double-ring infiltration and soil infiltration zoning in a semi-arid steppe, Water, 11, 1457, https://doi.org/10.3390/w11071457, 2019.
Li, Y., Li, Z., Cui, S., Jagadamma, S., and Zhang, Q.: Residue retention and minimum tillage improve physical environment of the soil in croplands: A global meta-analysis, Soil Till. Res., 194, 104292, https://doi.org/10.1016/j.still.2019.06.009, 2019.
Liao, K., Lai, X., Zhou, Z., Zhu, Q., and Han, Q.: A simple and improved model for describing soil hydraulic properties from saturation to oven dryness, Vadose Zone J., 17, 180082, https://doi.org/10.2136/vzj2018.04.0082, 2018.
Licht, M. A. and Al-Kaisi, M.: Strip-tillage effect on seedbed soil temperature and other soil physical properties, Soil Till. Res., 80, 233–249, https://doi.org/10.1016/j.still.2004.03.017, 2005.
Lozano, L. A., Soracco, C. G., Villarreal, R., Ressia, J. M., Sarli, G. O., and Filgueira, R. R.: Soil physical quality and soybean yield as affected by chiseling and subsoiling of a no-till soil, Rev. Bras. Cienc. Solo, 40, e0150160, https://doi.org/10.1590/18069657rbcs20150160, 2016.
Luo, Y., Hui, D., and Zhang, D.: Elevated CO2 stimulates net accumulations of carbon and nitrogen in land ecosystems: a meta-analysis, Ecology, 87, 53–63, https://doi.org/10.1890/04-1724, 2006.
Morbidelli, R., Saltalippi, C., Flammini, A., Cifrodelli, M., Picciafuoco, T., Corradini, C., and Govindaraju, R. S.: In situ measurements of soil saturated hydraulic conductivity: assessment of reliability through rainfall-runoff experiments, Hydrol. Process., 31, 3084–3094, https://doi.org/10.1002/hyp.11247, 2017.
Nouri, A., Lee, J., Yin, X., Tyler, D. D., Jagadamma, S., and Arelli, P.: Soil physical properties and soybean yield as influenced by long-term tillage systems and cover cropping in the Midsouth USA, Sustainability, 10, 4696, https://doi.org/10.3390/su10124696, 2018.
Olson, N. C., Gulliver, J. S., Nieber, J. L., and Kayhanian, M.: Remediation to improve infiltration into compact soils, J. Environ. Manage., 117, 85–95, https://doi.org/10.1016/j.jenvman.2012.10.057, 2013.
Perroux, K. M. and White, I.: Designs for disc permeameters, Soil Sci. Soc. Am. J., 52, 1205–1215, https://doi.org/10.2136/sssaj1988.03615995005200050001x, 1988.
Pittelkow, C. M., Liang, X., Linquist, B. A., van Groenigen, K. J., Lee, J., Lundy, M. E., van Gestel, N., Six, J., Venterea, R. T., and van Kessel, C.: Productivity limits and potentials of the principles of conservation agriculture, Nature, 517, 365, https://doi.org/10.1038/nature13809, 2014.
Reynolds, W. and Elrick, D.: In situ measurement of field-saturated hydraulic conductivity, sorptivity, and the α-parameter using the Guelph permeameter, Soil Sci., 140, 292–302, 1985.
Reynolds, W. D., Bowman, B. T., Brunke, R. R., Drury, C. F., and Tan, C. S.: Comparison of tension infiltrometer, pressure infiltrometer, and soil core estimates of saturated hydraulic conductivity, Soil Sci. Soc. Am. J., 64, 478–484, https://doi.org/10.2136/sssaj2000.642478x, 2000.
Rienzner, M. and Gandolfi, C.: Investigation of spatial and temporal variability of saturated soil hydraulic conductivity at the field-scale, Soil Till. Res., 135, 28–40, https://doi.org/10.1016/j.still.2013.08.012, 2014.
Ronayne, M. J., Houghton, T. B., and Stednick, J. D.: Field characterization of hydraulic conductivity in a heterogeneous alpine glacial till, J. Hydrol., 458, 103–109.
Rosenberg, M., Adams, D., and Gurevitch, J.: MetaWin: Statistical Software for Meta-Analysis, Sinauer Associates, Sunderland, MA, USA, ISBN 978-08-7893-761-5, 2000.
Sasal, M. C., Andriulo, A. E., and Taboada, M. A.: Soil porosity characteristics and water movement under zero tillage in silty soils in Argentinian Pampas, Soil Till. Res., 87, 9–18, https://doi.org/10.1016/j.still.2005.02.025, 2006.
Schaap, M. G., Leij, F. J., and van Genuchten, M. Th.: Neural network analysis for hierarchical prediction of soil water retention and saturated hydraulic conductivity, Soil Sci. Soc. Am. J., 62, 847–855, https://doi.org/10.2136/sssaj1998.03615995006200040001x, 1998.
Schlüter, S., Albrecht, L., Schwärzel, K., and Kreiselmeier, J.: Long-term effects of conventional tillage and no-tillage on saturated and near-saturated hydraulic conductivity – Can their prediction be improved by pore metrics obtained with X-ray CT?, Geoderma, 361, 114082, https://doi.org/10.1016/j.geoderma.2019.114082, 2020.
Schwärzel, K. and Punzel, J.: Hood infiltrometer – a new type of tension infiltrometer, Soil Sci. Soc. Am. J., 71, 1438–1447, https://doi.org/10.2136/sssaj2006.0104, 2007.
Shukla, M. K., Lal, R., and Ebinger, M.: Tillage effects on physical and hydrological properties of a typic argiaquoll in Central Ohio, Soil Sci., 168, 802–811, 2003.
Singh, B., Chanasyk, D. S., McGill, W. B., and Nybork, M. P. K.: Residue and tillage management effects on soil properties of a typic cryoboroll under continuous barley, Soil Till. Res., 32, 117–133, 1994.
Six, J., Paustian, K., Elliott, E. T., and Combrink, C.: Soil structure and organic matter I. Distribution of aggregate-size classes and aggregate-associated carbon, Soil Sci. Soc. Am. J. 64, 681–689, https://doi.org/10.2136/sssaj2000.642681x, 2000.
Strudley, M. W., Green, T. R., and Ascough II, J. C.: Tillage effects on soil hydraulic properties in space and time: State of the science, Soil Till. Res., 99, 4–48, https://doi.org/10.1016/j.still.2008.01.007, 2008.
Turmel, M. S., Speratti, A., Baudron, F., Verhulst, N., and Govaerts, B.: Crop residue management and soil health: a systems analysis, Agr. Syst., 134, 6–16, https://doi.org/10.1016/j.agsy.2014.05.009, 2015.
Vereecken, H., Maes, J., and Darius, P.: Estimating the soil moisture retention characteristics from texture, bulk density and carbon content, Soil Sci., 148, 389–403, 1989.
Xiao, L., Yuan, G., Feng, L., Bi, D., Wei, J., Shen, G., and Liu, Z.: Coupled effects of biochar use and farming practice on physical properties of a salt-affected soil with wheat–maize rotation, J. Soil. Sediment., 20, 3053–3061, https://doi.org/10.1007/s11368-020-02616-0, 2020.
Yang, Y., Jia, X., Wendroth, O., and Liu, B.: Estimating saturated hydraulic conductivity along a south-north transect in the Loess Plateau of China, Soil Sci. Soc. Am. J., 82, 1033–1045, https://doi.org/10.2136/sssaj2018.03.0126, 2018.
Yolcubal, I., Brusseau, M. L., Artiola, J. F., Wierenga, P., and Wilson, L. G.: 12-Environmental physical properties and processes, in: Environmental Monitoring and Characterization, edited by: Artiola, J. F., Pepper, I. L., and Brusseau, M. L., Academic Press, 207–239, ISBN 978-0-12-064477-3, 2004.
Yu, M., Zhang, L., Xu, X., Feger, K., Wang, Y., Liu, W., and Schwärzel, K.: Impact of land-use changes on soil hydraulic properties of Calcaric Regosols on the Loess Plateau, NW China, J. Plant Nutr. Soil Sci., 178, 486–498, https://doi.org/10.1002/jpln.201400090, 2015.
Zhang, J., Lei, T., Qu, L., Chen, P., Gao, X., Chen, C., Yuan, L., Zhang, M., and Su, G.: Method to measure soil matrix infiltration in forest soil, J. Hydrol., 552, 241–248, https://doi.org/10.1016/j.jhydrol.2017.06.032, 2017.
Zhu, Q., Nie, X., Zhou, X., Liao, K., and Li, H.: Soil moisture response to rainfall at different topographic positions along a mixed land-use hillslope, Catena, 119, 61–70, https://doi.org/10.1016/j.catena.2014.03.010, 2014.