the Creative Commons Attribution 4.0 License.
the Creative Commons Attribution 4.0 License.
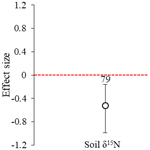
Soil δ15N is a better indicator of ecosystem nitrogen cycling than plant δ15N: A global meta-analysis
Kaihua Liao
Xiaoming Lai
Qing Zhu
The nitrogen-15 (15N) natural abundance composition (δ15N) in soils or plants is a useful tool to indicate the openness of ecosystem N cycling. This study aimed to evaluate the influence of the experimental warming on soil and plant δ15N. We applied a global meta-analysis method to synthesize 79 and 76 paired observations of soil and plant δ15N from 20 published studies, respectively. Results showed that the mean effect sizes of the soil and plant δ15N under experimental warming were −0.524 (95 % CI (confidence interval): −0.987 to −0.162) and 0.189 (95 % CI: −0.210 to 0.569), respectively. This indicated that soil δ15N had negative response to warming at the global scale, where warming had no significant effect on plant δ15N. Experimental warming significantly (p<0.05) decreased soil δ15N in Alkali and medium-textured soils, in grassland/meadow, under air warming, for a 4–10-year warming period and for an increase of >3 ∘C in temperature, whereas it significantly (p<0.05) increased soil δ15N in neutral and fine-textured soils and for an increase of 1.5–3 ∘C in temperature. Plant δ15N significantly (p<0.05) increased with increasing temperature in neutral and fine-textured soils and significantly (p<0.05) decreased in alkali soil. Latitude did not affect the warming effects on both soil and plant δ15N. However, the warming effect on soil δ15N was positively controlled by the mean annual temperature, which is related to the fact that the higher temperature can strengthen the activity of soil microbes. The effect of warming on plant δ15N had weaker relationships with environmental variables compared with that on soil δ15N. This implied that soil δ15N was more effective than plant δ15N in indicating the openness of global ecosystem N cycling.
- Article
(1293 KB) - Full-text XML
- BibTeX
- EndNote
Nitrogen (N) is one of the most important nutrient elements for plant growth and the key limiting factor for vegetation productivity (McLay et al., 2001; Zhu et al., 2018; Lu et al., 2020). On the one hand, if the available N in the soil is insufficient, it will damage and weaken the ecosystem service function, including the supply of primary material products, water conservation, climate regulation, etc. (Averill and Waring, 2018). On the other hand, if the available N in the soil is over supplied, it will also damage the structure and function of the ecosystem, resulting in a series of environmental problems such as soil acidification and imbalance of ecosystem nutrient (Schrijver et al., 2008). The intermediate products of the N cycling processes, such as nitrate nitrogen (-N), nitrous oxide (N2O) and nitric oxide (NO), may also cause eco-environmental pollution such as eutrophication of water bodies and aggravation of climate-related issues (Liao et al., 2019). Therefore, it is of great significance to reveal the openness of the ecosystem N cycle process for understanding the plant N fixation and long-term trend of N cycling and protecting the eco-environment (Wang et al., 2014; Wu et al., 2019). Openness is a measure of both N inputs and outputs relative to internal cycling and determines both the potential rate of N accumulation in the ecosystem and the potential for N losses following a disturbance (Rastetter et al., 2021).
The 15N natural abundance composition (δ15N) in soils or plants (leaves, shoots, fine roots, and litter) is often used to indicate the openness of ecosystem N cycling (Robinson, 2001). This is because the lighter isotope of 14N is always preferentially lost from the ecosystem. Thus, the isotopic fractionation effect results in gradual 15N enrichment in the ecosystem (Aranibar et al., 2004). The larger the δ15N value, the higher degree of openness of N cycling. In addition, soil δ15N also appears to reflect the degree of decomposition of the organic matter, showing that δ15N increases with processing (Craine et al., 2015). A large number of studies have confirmed that climate was the main factor regulating the soil and plant δ15N (Craine et al., 2015; Soper et al., 2015). Previous studies have demonstrated that precipitation had a negative effect on soil and plant δ15N from in situ evidence to cross-site syntheses (Swap et al., 2004; Soper et al., 2015). However, the influence of temperature on soil and plant δ15N remains controversial. Some studies have shown that soil and plant δ15N increased with temperature (Amundson et al., 2003; Craine et al., 2015), while others have indicated that δ15N decreased with temperature (Cheng et al., 2009; Sheng et al., 2014) or that they were not correlated (Yang et al., 2013). The various studies suggested that the responses of soil and plant δ15N to warming were very complex and not well understood. In addition to climate factor, soil and plant δ15N are affected by a variety of other environmental factors, such as vegetation type, topography, soil properties and management practices (Gurmesa et al., 2017; Wang et al., 2019). However, we know little about the influences of environmental factors on the warming effect on ecosystem N cycling, in terms of soil and plant δ15N.
Soil and air warming experiments have often been conducted to study the effect of warming on the ecosystem N cycling at the site scale (Schindlbacher et al., 2009). At present, the effect of experimental warming on soil and plant δ15N has not been studied on a global scale. The objectives of this study were to (i) detect the effect of experimental warming on the soil and plant δ15N based on a global meta-analysis of 20 studies and (ii) identify the main factors influencing the warming effect on the soil and plant δ15N. In addition, previous studies (e.g., Liu and Wang, 2009; Wang et al., 2014) have found that the correlation between soil δ15N and environmental factors was stronger than that for plant, which may be due to the fact that soil samples represented a long-term average for a given location, while plant samples were affected by the microenvironment or the short-term environmental fluctuations. Therefore, we specifically hypothesized that soil δ15N is a better indicator of ecosystem N cycling than plant δ15N.
Table 1Site characteristics from a global meta-analysis of 20 studies.
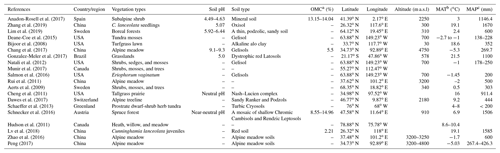
a Soil organic matter content; b Mean annual temperature; c Mean annual precipitation. If the soil organic carbon content was provided in the literature, soil organic matter content was determined by multiplying the organic carbon content by a coefficient of 1.724.
2.1 Source of data and selection criteria
Peer-reviewed journal articles and dissertations related to soil and plant δ15N under experimental warming were searched using Web of Science and China National Knowledge Infrastructure (CNKI; http://www.cnki.net) last access: 31 March 2020 (Table 1). The keywords used for the literature search were related to “nitrogen isotope composition”, “experimental warming”, and “ecosystems nitrogen cycling”.
Our criteria were as follows: at least one of the target variables was contained, including soil (different fractions, e.g., sand, silt, clay, aggregate, and bulk soil) and plant (leaves, shoots, roots, and litter) δ15N; studies with climate gradients (space-time substitution) were excluded and only field warming experimental studies were included; only data from control and warming treatments were applied for multifactor experiments; means, standard deviation (SD) (or standard error (SE)), and sample sizes were directly provided or could be calculated from the studies; if one article contained soil or plant δ15N in multiple years, only the latest results were applied since the observations should be independent in the meta-analysis (Hedges et al., 1999).
2.2 Data extraction and statistical analysis
In total, 20 published papers were selected from 54 published papers. The locations of warming experiments were presented, and their site information is listed in Table 1. For each study, the means, the statistical variation (SE or SD), and the sample size values for treatment and control groups were extracted for each response variable (δ15N). In addition to δ15N, the latitude, longitude, altitude, soil pH, organic matter content, vegetation type, mean annual precipitation (MAP), and mean annual temperature (MAT) were also extracted if they were provided (Table 1). All data were extracted from tables or digitized from graphs with the software GetData v2.2.4 (http://www.getdata-graph-digitizer.com, last access: 17 October 2020). A total of 79 and 76 paired observations for soil and plant δ15N were obtained, respectively.
The MetaWin 2.1 software (Sinauer Associates Inc., Sunderland, MA, USA) (Rosenberg et al., 2000) was used to perform meta-analysis in this study. The Hedges' d value was used as the effect size (Hedges et al., 1999). The absolute d value indicated the magnitude of the treatment impact. Positive or negative d values represented an increase or decrease effect of the treatment, respectively. Zero meant no difference between treatment and control groups. Resampling tests were incorporated into our meta-analysis using the bootstrap method (999 random replicates). The mean effect size (calculated from 999 iterations) and 95 % bootstrap confidence interval (CI) were then generated. If the 95 % CI values of d did not overlap zero, the effects of experimental warming on δ15N were considered significant at p<0.05. We used a random effects model to test whether warming had a significant effect on δ15N. To examine whether experimental conditions alter the response direction and magnitude of soil and plant δ15N, observations were further divided into subgroups according to the soil acidity–alkalinity (acid (pH < 6.5), neutral (6.5 < pH < 7.5), and alkali (pH > 7.5)), vegetation types (forest/shrub, moss/lichen, and grassland/meadow), warming treatments (soil warming, air warming, and both soil and air warming), soil texture (fine-, medium-, and coarse-textured soil), length of warming (< 4, 4–10, and > 10 years), and increase in temperature (< 1.5, 1.5–3, and > 3 ∘C). A random effects model with a grouping variable was used to compare responses among different subgroups. Linear regression analyses were applied to assess the relationships between the Hedges' d values and environmental factors (i.e., latitude, altitude, MAT, and MAP).
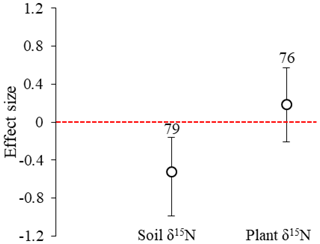
Figure 1Effect sizes of the experimental warming on soil and plant δ15N from a global meta-analysis of 20 studies. The error bars indicate effect sizes and 95 % bootstrap confidence interval (CI). The warming effect was statistically significant if the 95 % CI did not bracket zero. The sample size for each variable is shown next to the bar.
Across all sites, the mean effect sizes of the soil and plant δ15N under experimental warming were −0.524 (95 % CI: −0.987 to −0.162) and 0.189 (95 % CI: −0.210 to 0.569), respectively (Fig. 1). Experimental warming significantly (p<0.05) decreased soil δ15N in Alkali (mean effect size = −2.484; 95 % CI: −2.931 to −2.060) and medium-textured (mean effect size = −0.676; 95 % CI: −1.153 to −0.249) soils, in grassland/meadow (mean effect size = −0.609; 95 % CI: −1.076 to −0.190), under air warming (mean effect size = −0.652; 95 % CI: −1.081 to −0.273), for a 4–10-year warming period (mean effect size = −0.652; 95 % CI: −1.081 to −0.273) and for an increase of > 3 ∘C in temperature (mean effect size = -0.652; 95 % CI: −1.081 to −0.273). However, it significantly (p<0.05) increased soil δ15N in neutral (mean effect size = 0.359; 95 % CI: 0.078 to 0.620) and fine-texture soils (mean effect size = 2.394; 95 % CI: 1.770 to 3.735), and for an increase of 1.5–3 ∘C in temperature (mean effect size = 0.409; 95 % CI: 0.070 to 0.707) (Fig. 2). Experimental warming did not significantly (p > 0.05) change soil δ15N under other experimental conditions.
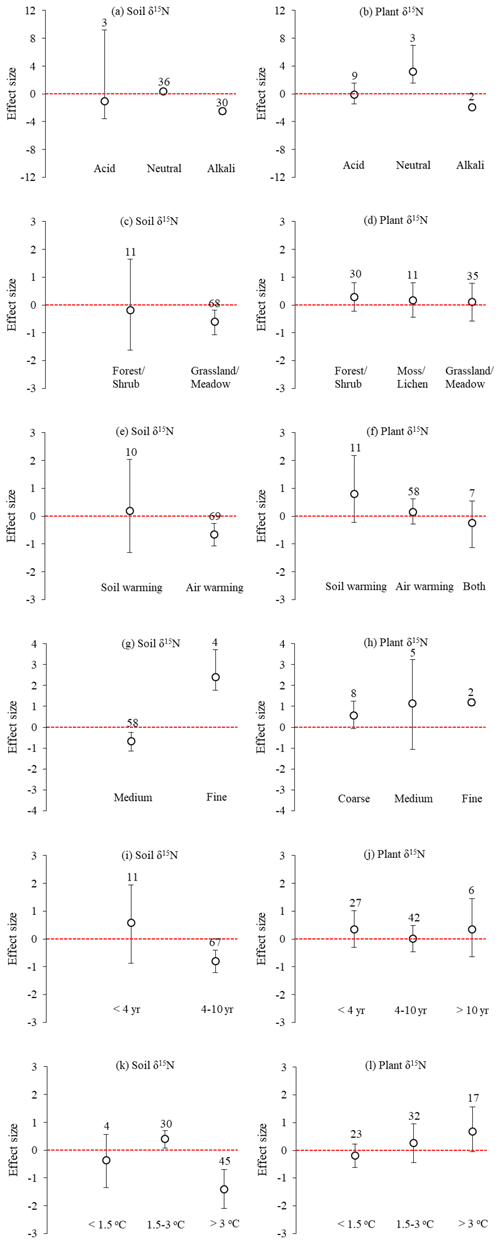
Figure 2Factors influencing the effect sizes of the soil and plant δ15N under experimental warming from a global meta-analysis of 20 studies, including (a–b) soil acidity–alkalinity, (c–d) vegetation types, (e–f) warming treatments, (g–h) soil texture, (i–j) length of warming, and (k–l) increase in temperature. The error bars indicate effect sizes and 95 % bootstrap confidence interval (CI). The warming effect was statistically significant if the 95 % CI did not bracket zero. The sample size for each variable is shown next to the bar.
In addition, experimental warming significantly (p<0.05) increased plant δ15N in neutral (mean effect size = 3.157; 95 % CI: 1.529 to 6.967) and fine-textured soils (mean effect size = 1.202; 95 % CI: 1.042 to 1.360), whereas it significantly (p<0.05) decreased plant δ15N in alkali soil (mean effect size = −1.930; 95 % CI: −2.325 to −1.573) (Fig. 2). Experimental warming did not significantly (p > 0.05) change plant δ15N under other experimental conditions.
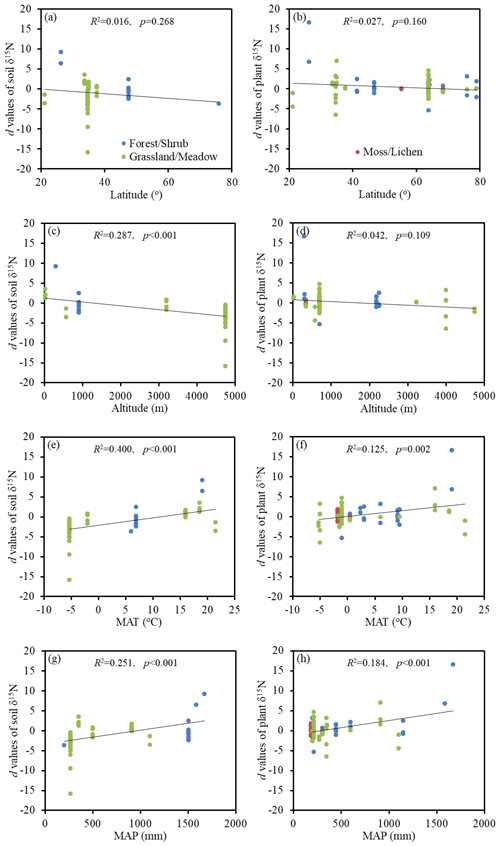
Figure 3Relationships between the Hedges' d values of soil and plant δ15N with the latitude, altitude, mean annual temperature (MAT), and mean annual precipitation (MAP) under experimental warming.
For soil and plant δ15N, their responses to experimental warming did not correlate well with latitude (p=0.268 and p=0.160, respectively) (Fig. 3a, b). However, the Hedges' d values of soil δ15N decreased significantly with altitude (p<0.001) (Fig. 3c) and increased significantly with MAT (p<0.001) and MAP (p<0.001) (Fig. 3e, g). In addition, the Hedges' d values of plant δ15N were also found to increase significantly with MAP (p<0.001) (Fig. 3h). However, the responses of plant δ15N to experimental warming did not correlate well with altitude (p=0.109) and MAT (p=0.002) (Fig. 3d, f).
A significant decreasing trend in soil δ15N and no significant trend in plant δ15N were found in this study. This is somewhat inconsistent with previous findings. Chang et al. (2017) observed that soil and plant δ15N values decreased under warming in the Tibetan permafrost. However, Zhang et al. (2019) found that the warming treatment significantly increased soil and plant δ15N in a subtropical forest. The various studies suggest that soil and plant δ15N are controlled by interactive effects of N fixation and mineralization. At the global scale, δ15N of N input (∼ 0) is generally lower than that of soil, so greater N fixation or higher N input (deposition and fertilization) under warming can result in a lower soil δ15N (Sorensen and Michelsen, 2011; Rousk and Michelsen, 2017; Wang et al., 2018).
Soil pH has an important influence on nitrification, denitrification, and N2O emissions from soils (Kyveryga et al., 2004). The results in this study showed that when the soil was alkaline the mean effect sizes of soil and plant δ15N under warming were negative, and when the soil was neutral they were positive (Fig. 2a, b). Compared with alkaline condition, the near-neutral conditions are more suitable for the biological activities of heterotrophic denitrifying bacteria (Simek and Cooper, 2002). Therefore, the denitrification activity is usually higher under neutral conditions, resulting in an enrichment of soil and plant N pools with 15N (Kyveryga et al., 2004). Vegetation type had limited effects on δ15N under warming, except for soil δ15N in grassland/meadow (Fig. 2c, d). This may be related to the differences in altitude, MAP, and MAT among three vegetation types (Table 1). The type of warming treatment was found to have a substantial effect on soil δ15N, showing that the mean effect size of soil δ15N under air warming was negative and less than that under soil warming (Fig. 2e, f). Salmon et al. (2016) have found that soil warming can increase N availability by stimulating mineralization of organic matter in the warmed active layer. In addition, air warming directly impacts aboveground temperatures and has an indirect effect on soil δ15N (Pardo et al., 2006). From Fig. 2g and h, the finer the soil texture, the more significant the positive effect of warming on soil and plant δ15N. One possible reason is that the finer the soil texture, the stronger the adsorption of various ions on the soil and the smaller the leaching loss of the soil, resulting in greater residual amount of 15N in the soil (Webster et al., 1986). In addition, the longer warming period and the greater increase in temperature resulted in a more negative effect of warming on soil δ15N (Fig. 2i, k). Chang et al. (2017) deduced that N fixation was greater under warming and consequently resulted in a lower soil δ15N.
In the study of Mayor et al. (2015), soil and plant δ15N values were significantly (p<0.001) and negatively correlated with latitude at the global scale. However, the Hedges' d values of soil and plant δ15N had weak correlations with latitude in this study (Fig. 3). The warming effect on soil δ15N was significantly (p<0.001) influenced by altitude, MAT and MAP. Among these, the strongest correlation was observed for MAT. It is possible that soil δ15N increased with increasing MAT when the MAT exceeded a certain threshold (e.g., 9.8 ∘C as proposed by Craine et al., 2015). In this case, the increase in MAT can enhance the positive effect of experimental warming on soil δ15N. In addition, the MAT can also affect ecosystem N cycle by influencing soil texture. Craine et al. (2015) reported that hot sites had greater clay concentrations than cold sites. As depicted in Fig. 2g, the finer the texture of the soil, the more significant the effect of experimental warming on soil δ15N. High d values of soil δ15N corresponded to MAT of about 20 ∘C, which was the most suitable temperature for nitrification and denitrification. However, warming had a substantial negative impact on soil δ15N when MAT decreased to around −5 ∘C. Recently, Rousk et al. (2018) also found that the increase in temperature in the Arctic promoted the biological N fixation, which can decrease the soil δ15N. The decrease of d values of soil δ15N with increasing altitude and decreasing MAP in this study might be caused by the positive response of d values to MAT.
The relationships between the d values and environmental variables for plant δ15N were weaker than those for soil δ15N (Fig. 3). One possible reason is that several other factors (e.g., plant N concentrations and species richness) might co-regulate plant δ15N (Wu et al., 2019). This is consistent with the study of Craine et al. (2009), who found different inflection points in soil and plant δ15N relationships with MAT. In addition, plants are generally depleted in 15N relative to soils. Above results implied that soil δ15N was more efficient in indicating the openness of ecosystem N cycling than plant δ15N at the global scale. Although the present study provided a global meta-analysis of the responses of δ15N to experimental warming, the magnitude of these responses might be uncertain. For example, a small number of observations were obtained in moss/lichen under soil warming and both soil and air warming treatments, which would affect the results of the meta-analysis. Future research should take more experimental data into account in order to better investigate the warming effects on δ15N.
Our global meta-analysis indicated a significant decreasing trend in soil δ15N and no significant trend in plant δ15N under experimental warming. Latitude did not affect the warming effects on δ15N. However, the warming effect on δ15N was related to soil acidity–alkalinity; texture; vegetation type; warming treatment and period; and increase in temperature, altitude, MAT, and MAP. The effect of warming on soil δ15N was better correlated with environmental variables compared with that on plant δ15N. Our findings should be useful for understanding the underlying mechanisms of the response of ecosystem N cycling to global warming.
The data that support the findings of this study are available from the corresponding author upon request.
KL and QZ designed this study, KL and XL performed the meta-analysis, KL and QZ obtained funding, and KL and XL wrote the paper with contributions from QZ.
The contact author has declared that neither they nor their co-authors have any competing interests.
Publisher’s note: Copernicus Publications remains neutral with regard to jurisdictional claims in published maps and institutional affiliations.
We thank the two anonymous reviewers and editor for their efforts on this paper. Support for this research was provided by the National Natural Science Foundation of China and by Chinese Academy of Sciences.
his study was financially supported by the National Natural Science Foundation of China (grant nos. 42125103 and 42171077), and the Youth Innovation Promotion Association, Chinese Academy of Sciences (grant no. 2020317).
This paper was edited by Kate Buckeridge and reviewed by Kate Buckeridge and one anonymous referee.
Aerts, R., Callaghan, T. V., Dorrepaal, E., van Logtestijn, R. S. P., and Cornelissen, J. H. C.: Blackwell Publishing Ltd Seasonal climate manipulations result in species-specific changes in leaf nutrient levels and isotopic composition in a sub-arctic bog. Funct. Ecol., 23, 680–688, https://doi.org/10.1111/j.1365-2435.2009.01566.x, 2009.
Amundson, R., Austin, A. T., Schuur, E. A. G., Yoo, K., Matzek, V., Kendall, C., Uebersax, A., Brenner, D., and Baisden, W. T.: Global patterns of the isotopic composition of soil and plant nitrogen, Global Biogeochem. Cy., 17, 1031, https://doi.org/10.1029/2002GB001903, 2003.
Anadon-Rosell, A., Ninot, J. M., Palacio, S., Grau, O., Nogués, S., Navarro, E., Carmen Sancho, M., and Carrillo, E.: Four years of experimental warming do not modify the interaction between subalpine shrub species, Oecologia, 183, 1167–1181, https://doi.org/10.1007/S00442-017-3830-7, 2017.
Aranibar, J. N., Otter, L., Macko, S. A., Feral, C. J. W., Epstein, H. E., Dowty, P. R., Eckardt, F., Shugart, H. H., and Swap, R. J.: Nitrogen cycling in the soil-plant system along a precipitation gradient in the Kalahari sands. Global Change Biol., 10, 359–373, https://doi.org/10.1111/j.1365-2486.2003.00698.x, 2004.
Averill, C. and Waring, B.: Nitrogen limitation of decomposition and decay: How can it occur?, Global Change Biol., 4, 1417–1427, https://doi.org/10.1111/gcb.13980, 2018.
Bijoor, N. S., Czimczik, C. I., Pataki, D. E., and Billings, S. A.: Effects of temperature and fertilization on nitrogen cycling and community composition of an urban lawn. Global Change Biol., 14, 2119–2131, https://doi.org/10.1111/j.1365-2486.2008.01617.x, 2008.
Chang, R., Wang, G., Yang, Y., and Chen, X.: Experimental warming increased soil nitrogen sink in the Tibetan permafrost, J. Geophys. Res.-Biogeo., 122, 1870–1879, https://doi.org/10.1002/2017JG003827, 2017.
Cheng, W. X., Chen, Q. S., Xu, Y. Q., Han, X. G., and Li, L. H.: Climate and ecosystem 15N natural abundance along a transect of Inner Mongolian grasslands: Contrasting regional patterns and global patterns, Global Biogeochem. Cy., 23, GB2005, https://doi.org/10.1029/2008GB003315, 2009.
Cheng, X., Luo, Y., Xu, X., Sherry, R., and Zhang, Q.: Soil organic matter dynamics in a North America tallgrass prairie after 9 yr of experimental warming, Biogeosciences, 8, 1487–1498, https://doi.org/10.5194/bg-8-1487-2011, 2011.
Craine, J. M., Elmore, A. J., Wang, L., Augusto, L., Baisden, W. T., Brookshire, E. N. J., Cramer, M. D., Hasselquist, N. J., Hobbie, E. A., Kahmen, A., Koba, K., Kranabetter, J. M., Mack, M. C., Marin-Spiotta, E., Mayor, J. R., McLauchlan, K. K., Michelsen, A., Nardoto, G. B., Oliveira, R. S., Perakis, S. S., Peri, P. L., Quesada, C. A., Richter, A., Schipper, L. A., Stevenson, B. A., Turner, B. L., Viani, R. A. G., Wanek, W., and Zeller, B.: Convergence of soil nitrogen isotopes across global climate gradients. Sci. Rep., 5, 8280, https://doi.org/10.1038/srep08280, 2015.
Dawes, M. A., Schleppi, P., Hättenschwiler, S., Rixen, C., and Hagedorn, F.: Soil warming opens the nitrogen cycle at the alpine treeline, Global Change Biol., 23, 421–434, https://doi.org/10.1111/gcb.13365, 2017.
Deane-Coe, K. K., Mauritz, M., Celis, G., Salmon, V., Crummer, K. G., Natali, S. M., and Schuur, E. A. G.: Experimental warming alters productivity and isotopic signatures of tundra mosses, Ecosystems, 18, 1070–1082, https://doi.org/10.1007/s00442-015-3427-y, 2015.
Gonzalez-Meler, M. A., Silva, L. B. C., Dias-De-Oliveira, E., Flower, C. E., and Martinez, C. A.: Experimental air warming of a Stylosanthes capitata, vogel dominated tropical pasture affects soil respiration and nitrogen dynamics. Front. Plant Sci., 8, 46, https://doi.org/10.3389/fpls.2017.00046, 2017.
Gurmesa, G. A., Lu, X., Gundersen, P., Fang, Y., Mao, Q., Hao, C., and Mo, J.: Nitrogen input 15N signatures are reflected in plant 15N natural abundances in subtropical forests in China, Biogeosciences, 14, 2359–2370, https://doi.org/10.5194/bg-14-2359-2017, 2017.
Hedges, L. V., Gurevitch, J., and Curtis, P. S.: The meta-analysis of response ratios in experimental ecology, Ecology, 80, 1150–1156, https://doi.org/10.1890/0012-9658(1999)080[1150:TMAORR]2.0.CO;2, 1999.
Hudson, J. M. G., Henry, G. H. R., and Cornwell, W. K.: Taller and larger: shifts in Arctic tundra leaf traits after 16 years of experimental warming, Global Change Biol., 17, 1013–1021, https://doi.org/10.1111/j.1365-2486.2010.02294.x, 2011.
Kyveryga, P. M., Blackmer, A. M., Ellsworth, J. W., and Isla, R.: Soil pH effects on nitrification of fall-applied anhydrous ammonia, Soil Sci. Soc. Am. J., 68, 545–551, https://doi.org/10.2136/sssaj2004.0545, 2004.
Liao, K., Lai, X., Zhou, Z., Zeng, X., Xie, W., Castellano, M. J., and Zhu, Q.: Whether the rock fragment content should be considered when investigating nitrogen cycle in stony soils?, J. Geophys. Res.-Biogeo., 124, 521–536, https://doi.org/10.1029/2018JG004780, 2019.
Lim, H., Oren, R., Näsholm, T., Strömgren, M., Lundmark, T., Grip, H., and Linder, S.: Boreal forest biomass accumulation is not increased by two decades of soil warming, Nat. Clim. Change, 9, 49–52, https://doi.org/10.1038/s41558-018-0373-9, 2019.
Liu, W., and Wang, Z.: Nitrogen isotopic composition of plant-soil in the Loess Plateau and its responding to environmental change, Chin. Sci. Bull., 54, 272–279, https://doi.org/10.1007/s11434-008-0442-y, 2009.
Lu, Y., Gao, Y., and Yang, T.: A review of mass flux monitoring and estimation methods for biogeochemical interface processes in watersheds, J. Geogr. Sci., 30, 881–907, https://doi.org/10.1007/s11442-020-1760-5, 2020.
Lv, C., Zhang, Q., Hao, Y., Chen, Y., and Yang, Y.: Influence of short-term warming on the composition of stable carbon and nitrogen isotopes in Cunninghamia lanceolata in subtropical region of China, Forest Res., 31, 27–32, https://doi.org/10.13275/j.cnki.lykxyj.2018.05.004, 2018. (in Chinese)
Mayor, J., Bahram, M., Henkel, T., Buegger, F., Pritsch, K., and Tedersoo, L.: Ectomycorrhizal impacts on plant nitrogen nutrition: emerging isotopic patterns, latitudinal variation and hidden mechanisms, Ecol. Lett., 18, 96–107, https://doi.org/10.1111/ele.12377, 2015.
McLay, C., Dragten, R., Sparling, G., and Selvarajah, N.: Predicting groundwater nitrate concentrations in a region of mixed agricultural land use, a comparison of three approaches, Environ. Pollut., 115, 191–204, https://doi.org/10.1016/S0269-7491(01)00111-7, 2001.
Munir, T. M., Khadka, B., Xu, B., and Strack, M.: Mineral nitrogen and phosphorus pools affected by water table lowering and warming in a boreal forested peatland, Ecology, 10, e1893, https://doi.org/10.1002/eco.1893, 2017.
Natali, S. M., Schuur, E. A. G., and Rubin, R. L.: Increased plant productivity in Alaskan tundra as a result of experimental warming of soil and permafrost, J. Ecol., 100, 488–498, https://doi.org/10.1111/j.1365-2745.2011.01925.x, 2012.
Pardo, L. H., Templer, P. H., Goodale, C. L., Duke, S., Groffman, P. M., Adams, M. B., Boeckx, P., Boggs, J., Campbell, J., Colman, B., Compton, J., Emmett, B., Gundersen, P., Kjønaas, J., Lovett, G., Mack, M., Magill, A., Mbila, M., Mitchell, M. J., McGee, G., McNulty, S., Nadelhoffer, K., Ollinger, S., Ross, D., Rueth, H., Rustad, L., Schaberg, P., Schiff, S., Schleppi, P., Spoelstra, J., and Wessel, W.: Regional assessment of N saturation using foliar and root δ15N, Biogeochemistry, 80, 143–171, https://doi.org/10.1007/s10533-006-9015-9, 2006.
Peng, A.: Effects of artificial warming on alpine meadow in the permafrost region of Qinghai-Tibet Plateau. Master dissertation, The University of Chinese Academy of Sciences, 2017 (in Chinese).
Rastetter, E. B., Kling, G. W., Shaver, G. R., Crump, B. C., Gough, L., and Griffin, K. L.: Ecosystem recovery from disturbance is constrained by N cycle openness, vegetation-soil N distribution, form of N losses, and the balance between vegetation and soil-microbial processes, Ecosystems, 24, 667–685, https://doi.org/10.1007/s10021-020-00542-3, 2021.
Robinson, D.: δ15N as an integrator of the nitrogen cycle, Trends Ecol. Evol. (Amst.), 16, 153–162, https://doi.org/10.1016/S0169-5347(00)02098-X, 2001.
Rosenberg, M., Adams, D., and Gurevitch, J.: MetaWin: Statistical Software for Meta-Analysis, Sinauer Associates, Sunderland, MA, USA, 128 pp., 2000.
Rousk, K. and Michelsen, A.: Ecosystem nitrogen fixation throughout the snow-free period in subarctic tundra: Effects of willow and birch litter addition and warming, Global Change Biol., 23, 1552–1563, https://doi.org/10.1111/gcb.13418, 2017.
Rousk, K., Sorensen, P. L., and Michelsen, A.: What drives biological nitrogen fixation in high arctic tundra: Moisture or temperature?, Ecosphere, 9, e02117, https://doi.org/10.1002/ecs2.2117, 2018.
Rui, Y., Wang, S., Xu, Z., Wang, Y., Chen, C., Zhou, X., Kang, X., Lu, S., Hu, Y., Lin, Q., and Luo, C.: Warming and grazing affect soil labile carbon and nitrogen pools differently in an alpine meadow of the Qinghai–Tibet Plateau in China, J. Soils Sediment., 11, 903–914, https://doi.org/10.1007/s11368-011-0388-6, 2011.
Salmon, V. G., Soucy, P., Mauritz, M., Celis, G., Natali, S. M., Mack, M. C., and Schuur, E. A. G.: Nitrogen availability increases in a tundra ecosystem during five years of experimental permafrost thaw, Global Change Biol., 22, 1927–1941, https://doi.org/10.1111/gcb.13204, 2016.
Schaeffer, S. M., Sharp, E., Schimel, J. A. P., and Welker, J. M.: Soil–plant N processes in a High Arctic ecosystem, NW Greenland are altered by long-term experimental warming and higher rainfall, Global Change Biol., 19, 3529–3539, https://doi.org/10.1111/gcb.12318, 2013.
Schindlbacher, A., Zechmeister-Boltenstern, S., and Jandl, R.: Carbon losses due to soil warming: do autotrophic and heterotrophic soil respiration respond equally?, Global Change Biol., 15, 901–913, https://doi.org/10.1111/j.1365-2486.2008.01757.x, 2009.
Schnecker, J., Borken, W., Schindlbacher, A., and Wanek, W.: Little effects on soil organic matter chemistry of density fractions after seven years of forest soil warming, Soil Biol. Biochem., 103, 300–307, https://doi.org/10.1016/j.soilbio.2016.09.003, 2016.
Schrijver, A. D., Verheyen, K., Mertens, J., Staelens, J., Wuyts, K., and Muys, B.: Nitrogen saturation and net ecosystem production. Nature, 451, E1, https://doi.org/10.1038/nature06578, 2008.
Sheng, W., Yu, G., Fang, H., Liu, Y., Wang, Q., Chen, Z., and Zhang, L.: Regional patterns of 15N natural abundance in forest ecosystems along a large transect in eastern China, Sci. Rep., 4, 4249, https://doi.org/10.1038/srep04249, 2014.
Simek, M., and Cooper, J. E.: The influence of soil pH on denitrification: progress towards the understanding of this interaction over the last 50 years, Eur. J. Soil Sci., 53, 345–354, https://doi.org/10.1046/j.1365-2389.2002.00461.x, 2002.
Soper, F. M., Richards, A. E., Siddique, I., Aidar, M. P. M., Cook, G. D., Hutley, L. B., Robinson, N., and Schmidt, S.: Natural abundance (δ15N) indicates shifts in nitrogen relations of woody taxa along a savanna-woodland continental rainfall gradient, Oecologia, 178, 297–308, https://doi.org/10.1007/s00442-014-3176-3, 2015.
Sorensen, P. L. and Michelsen, A.: Long-term warming and litter addition affects nitrogen fixation in a subarctic heath, Global Change Biol., 17, 528–537, https://doi.org/10.1111/j.1365-2486.2010.02234.x, 2011.
Swap, R. J., Aranibar, J. N., Dowty, P. R., Gilhooly, W. P., and Macko, S. A.: Natural abundance of 13C and 15N in C3 and C4 vegetation of southern Africa: patterns and implications, Global Change Biol., 10, 350–358, https://doi.org/10.1111/j.1365-2486.2003.00702.x, 2004.
Wang, C., Wang, X., Liu, D., Wu, H., Lv, X., Fang, Y., Cheng, W., Luo, W., Jiang, P., Shi, J., Yin, H., Zhou, J., Han, X., and Bai, E.: Aridity threshold in controlling ecosystem nitrogen cycling in arid and semi-arid grasslands, Nat. Commun., 5, 4799, https://doi.org/10.1038/ncomms5799, 2014.
Wang, Q., Nian, J., Xie, X., Yu, H., Zhang, J., Bai, J., Dong, G., Hu, J., Bai, B., Chen, L., Xie, Q., Feng, J., Yang, X., Peng, J., Chen, F., Qian, Q., Li, J., and Zuo, J.: Genetic variations in ARE1 mediate grain field by modulating nitrogen utilization in rice, Nat. Commun., 9, 735, https://doi.org/10.1038/s41467-017-02781-w, 2018.
Wang, X., Jiang, Y., Ren, H., Yu, F., and Li, M.: Leaf and soil δ15N patterns along elevational gradients at both treelines and shrublines in three different climate zones, Forests, 10, 557, https://doi.org/10.3390/f10070557, 2019.
Webster, C. P., Belford, R. K., and Cannell, R. Q.: Crop uptake and leaching losses of 15N labelled fertilizer nitrogen in relation to waterlogging of clay and sandy loam soils, Plant Soil, 92, 89–101, 1986.
Wu, J., Song, M., Ma, W., Zhang, X., Shen, Z., Tarolli, P., Wurst, S., Shi, P., Ratzmann, G., Feng, Y., Li, M., Wang, X., and Tietjen, B.: Plant and soil's δ15N are regulated by climate, soil nutrients, and species diversity in alpine grasslands on the northern Tibetan Plateau, Agr. Ecosyst. Environ., 281, 111–123, https://doi.org/10.1016/j.agee.2019.05.011, 2019.
Yang, Y. H., Ji, C. J., Robinson, D., Zhu, B., Fang, H. J., Shen, H. H., and Fang, J. Y.: Vegetation and soil 15N natural abundance in alpine grasslands on the tibetan plateau: patterns and implications, Ecosystems, 16, 1013–1024, https://doi.org/10.1007/s10021-013-9664-1, 2013.
Zhang, Q., Zhou, J., Li, X., Yang, Z., Zheng, Y., Wang, J., Lin, W., Xie, J., Chen, Y., and Yang, Y.: Are the combined effects of warming and drought on foliar C:N:P:K stoichiometry in a subtropical forest greater than their individual effects?, For. Ecol. Manage., 448, 256–266, https://doi.org/10.1016/j.foreco.2019.06.021, 2019.
Zhao, Y., Xu, L., Yao, B., Ma, Z., Zhang, C., Wang, F., and Zhou, H.: Influence of simulated warming to the carbon, nitrogen and their stability isotope-(δ13C, δ15N) contents in alpine meadow plant leaves, Acta Bot. Boreal Occident Sin., 36, 777–783, https://doi.org/10.7606/j.issn.1000-4025.2016.04.0777, 2016 (in Chinese).
Zhu, Q., Castellano, M. J., and Yang, G. S.: Coupling soil water processes and the nitrogen cycle across spatial scales: Potentials, bottlenecks and solutions, Earth-Sci. Rev., 187, 248–258, https://doi.org/10.1016/j.earscirev.2018.10.005, 2018.