the Creative Commons Attribution 4.0 License.
the Creative Commons Attribution 4.0 License.
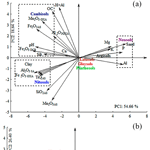
Physical, chemical, and mineralogical attributes of a representative group of soils from the eastern Amazon region in Brazil
Edna Santos de Souza
Antonio Rodrigues Fernandes
Anderson Martins De Souza Braz
Fábio Júnior de Oliveira
Luís Reynaldo Ferracciú Alleoni
Milton César Costa Campos
Amazonian soils are heterogeneous. However, few studies have been carried out in the Amazon, mainly because of its considerable size, which complicates the collection of data and the ability to plan for the sustainable use of natural resources. In this study, the physical, chemical, and mineralogical attributes of soils in the state of Pará, Brazil, were characterized by examining the particle size, fertility, silicon extracted by sodium hydroxide, iron, and aluminum, and manganese extracted by sulfuric acid, sodium citrate-bicarbonate-dithionite, and ammonium oxalate + oxalic acid. A descriptive analysis, multivariate principal component analysis, and cluster analysis were performed. The soils had low concentrations of bioavailable P, Ca2+, Mg2+, and K and had high concentrations of Al3+, Si, and Al oxide in the Cambisols. Concentrations of Fe and Mn oxides were higher in both the Cambisols and Nitosols, which are rich in oxidic minerals. The multivariate analysis indicated an association between the organic carbon content and pH, P, Ca, Mg, and K concentrations. An additional association was observed between clay, potential acidity, and the Fe and Al oxide concentrations.
- Article
(10220 KB) - Full-text XML
-
Supplement
(151 KB) - BibTeX
- EndNote
In the Amazon region, soils are heterogeneous due to the presence of different parent materials and bioclimatic conditions that affect pedogenetic processes (Quesada et al., 2009) and change the morphological, physical, chemical, and mineralogical attributes of the soil (Quesada et al., 2010). There is limited knowledge on the characteristics of the soils in this region, and existing knowledge is principally based on soil surveys with few details, which limits proper planning for land use practices (Moreira and Fageria, 2009).
Two physiographic environments are dominant in the Amazon region: (i) uplands, which represent approximately 80 % of the region (these areas are never flooded, and they consist of deep and highly weathered soils), and (ii) floodplains, which lie at the edges of the plains that border the rivers and are subjected to elevated water tables and periodic flooding (Moreira and Fageria, 2009).
The soils in the upland environments are acidic, have low fertility and low cation exchange capacity (CEC), and contain silicate minerals with low activity. Typically, the soils in lowland environments contain high levels of silt, clay, fine sand, CEC, and base saturation (V %), and contain highly active silicate minerals (Quesada et al., 2009). However, despite the differences between the two environments, the predominant clay mineralogy in both is kaolinitic and oxidic (Melo et al., 2006).
In the state of Pará, Brazil, the predominant soil types are Latosols and Argisols, which represent more than 74 % of the soils in the region. Plintosols, Nitosols, Neosols, Spodosols, and Cambisols (Quesada et al., 2010; Rodrigues et al., 2007) are also present but in smaller quantities. Detailed knowledge of the physical and chemical properties of these soils is essential to land management strategies that can prevent financial loss and environmental damage.
The main economic activities in the state of Pará and the Amazon include agriculture, cattle rearing, vegetable production, and mineral extraction (Bowman et al., 2012; Souza et al., 2013). When carried out without planning or any prior knowledge of the local bioclimatic and geomorphological characteristics, these activities contribute to increased deforestation and the loss of biodiversity (Godar et al., 2012). However, a natural balance can be maintained when soils are used sustainably and are thereby preserved. Thus, the physical, chemical, and biological attributes of soils should be considered for adequate soil management (Aquino et al., 2014).
The practice of forest burning in the Amazon has contributed to increased compaction and reduced soil fertility. Furthermore, carbon concentrations in tropical soils can be reduced by up to 50 % after a forest is burned (Aquino et al., 2014). Soil fertility can be reduced in forest areas after 3 years of continuous cultivation when rudimentary techniques are adopted in agriculture production, which highlights the fragility of the Amazon ecosystem (Melo et al., 2006). The low degree of fertility usually results in an abandonment of one area in favor of new forested areas, which further contributes to deforestation (Béliveau et al., 2009; Souza et al., 2013). One approach to the prevention of deforestation and soil degradation is to use management and conservation techniques that are appropriate in this region. These methods depend on the knowledge of soil attributes.
The diversity of the Amazon ecosystem and studies relating to soils in this region should be considered during the application of techniques that can prevent the exploitation of unsustainable natural resources. Information relating to soil attributes can serve as a basis for public policies that target agricultural planning and technologies that increase land use efficiency and conserve biodiversity. The aim of this study was to characterize the physical, chemical, and mineralogical attributes of the soil types in the state of Pará, Brazil.
2.1 Description of the study area
Soil samples were collected from 33 municipalities in the state of Pará, Brazil. When selecting the municipalities, the following factors were considered: (i) the human population, (ii) the level of mineral extraction activity, and (iii) the agricultural use of the land and whether the last two factors accounted for most of the economic activity. In addition, cities located along the Trans-Amazonian Highway (BR-230) (Fig. 1) were considered. This road crosses a border region that has received investment from the federal government since the 1970s and was earmarked for implementing large projects, such as hydroelectricity, mineral extraction, and agrarian reform settlements. These activities have contributed to land speculation and high rates of deforestation and soil degradation. The municipalities in Pará that were not selected for sampling were rejected for multiple reasons, such as a large number of indigenous areas, national parks, extractive reserves or conservation areas, and the difficulty of access across the territorial extension of the state of Pará, which covers more than 1.2 million km2 (Fig. 1).
The occupation and development plan for the state of Pará and the Amazon, in general, intensified after the construction of highways; most of the municipalities were created around it (Pacheco, 2009). Similarly, the exploitation of natural resources is concentrated along the highways, where deforestation is prevalent (Godar et al., 2012). Studies of soil attributes in preserved areas are fundamental to the public policy of the state and to rural settlements. Furthermore, the soil classes covered in this study include the main soil classes and the pedoenvironments that represent the state of Pará (Rodrigues et al., 2007) and have higher agricultural potential.
The soil samples covered 29 geological formations (Fig. 2) from the Archean, Precambrian, Ordovician, Carboniferous, Jurassic, Cretaceous, Tertiary, and Quaternary periods. Samples collected in the state's northeastern region are from the Barreiras Group and lateritic debris Pleistocene, which covered 18 % of the study area. In the south and southeast of Pará, soil samples were mostly from the Couto Magalhães Formation, Itapecuru Formation, Bacajá Complex, and Maloquinha Intrusive Suite. The southwest region of Pará has the greatest geological diversity among the samples and includes the Diabase Penatecaua Formation, Curuá Group, Maloquinha Intrusive Suite, and the Parauari Intrusive Suite. In the western region, the Alter do Chão Formation predominates. Sedimentary rock, shale, siltstone, sandstone, and, to a lesser extent, volcanic rock, such as basalt and diabase, are dominant in Pará. The broadest coverage of soil groups is the Argisols and Latosols, which comprise 80 % of the soil classes, and are present in 97 % of the geological formations included in this study. The Nitosols and Argisols on the Diabase Penatecaua Formation represent 9 % of the soil samples collected. In general, the soils in Pará are infertile and acidic, with kaolinitic and oxidic mineralogy (Falesi, 1986).
The climate is tropical rainy (Af, Am, and Aw types; Köppen classification), which is characterized by monthly temperatures that always have an average above 18 ∘C but vary from region to region across the state. Climate subtype Af has a dry season and annual rainfall between 2000 and 3000 mm. Climate subtype Am is a monsoon climate with rainfall between 1500 and 3000 mm. Climate subtype Aw has an average annual rainfall ranging from 1000 to 2000 mm (Fig. 3) and average annual temperatures ranging from 25 to 27 ∘C.
2.2 Sampling sites
The soils collected belong to the following soil orders: Latosols, Argisols, Nitosols, Plintosols, Neosols, Gleisols, and Cambisols, which are generally equivalent to Ferralsols, Acrisols, Nitisols, Plinthosols, Arenosols, Fluvisols, Cambisols, and Gleysols according to the FAO/WRB classification system (FAO, 2006) and Oxisols, Ultisols, Alfisols, Plintaqualfs, Entisols, Aquents, and Inceptisols according to the USDA Soil Taxonomy (Soil Survey Staff, 2014) (Supplement Table S1). These soil classes were mapped and characterized with reference to established criteria and were divided into the categories established by the Brazilian Soil Classification System (Embrapa, 2013). This process was achieved by superimposing maps of the soils found in the region, such as the Ecological-Economic Zoning map that covers the area around highways BR-163 (Cuiabá-Santarém) and BR-230 (Trans-Amazonian) in the state of Pará (Rodrigues et al., 2007) and the soils found on and around the Trans-Amazonian Highway (Falesi, 1986).
When selecting these areas, we ensured that the biogeoclimatic conditions were as homogeneous as possible. The samples were collected from areas of primary or secondary vegetation with over 20 years of regeneration without human disturbance, such as deforestation, agricultural use, and mining. Collection sites were approximately 100 m from the highway. Samples were collected from the top of the surface layer (0.0–0.2 m) and further down in the surface layer at depths that coincided with the diagnostic parameters of the soil classes as follows: at 0.8–1.0 m for Latosols, Argisols, Nitosols, Gleisols, and Plintosols and at 0.4–0.6 m for Neosols and Cambisols. Ten samples were collected at a spacing of approximately 70 m to obtain composite samples due to the homogeneity of the soils in terms of color, topography, drainage, and vegetation.
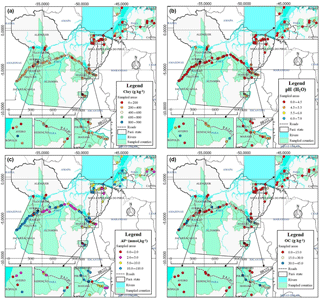
Figure 4Spatial distribution map of clay (a), pH (b), Al3+ (c), and organic carbon (OC) (d) of the soils of the state of Pará.
From each area, three composite samples were collected from the subsurface layers, making a total of 528 samples from 88 areas. In the other municipalities, collections were randomized according to the availability of the areas with primary or secondary vegetation in the final stage of regeneration (more than 20 years old). Samples were obtained using a Dutch stainless-steel soil auger to avoid contamination. The samples were air-dried, crushed, homogenized, and passed through a 2.0 mm mesh sieve to produce fine air-dried soil.
2.3 Physical and chemical analyses
Particle size analysis was carried out using a pipette method with a 0.1 M NaOH solution as the chemical dispersant under high-speed mechanical stirring for 10 min. The clay portion was separated by sedimentation and the sand by sieving, and the silt was calculated based on the pre- and post-difference (Embrapa, 2011).
Exchangeable calcium, magnesium, and aluminum contents were extracted by a 1 M KCl solution. Aluminum concentration was quantified by titrating with 0.025 M NaOH, and the Ca and Mg concentrations were quantified by atomic absorption spectrophotometry. K and P contents were extracted using a Mehlich-1 (0.05 M HCl + 0.0125 M H2SO4) solution. Next, the K concentration was determined by flame photometry, and the P concentration was determined by colorimetry (Embrapa, 2011). Based on these results, the sum of the bases, CEC, V %, and aluminum saturation (m %) were calculated. The pH was determined potentiometrically using a soil to water ratio of 1:2.5. Potential acidity (H + Al) was extracted using a 1 M buffered solution of calcium acetate at a pH of 7.0 and was determined by titration using NaOH in accordance with the methods used in Embrapa (2011). Organic carbon content was determined using the Walkley–Black method, in which organic matter (OM) was oxidized with potassium dichromate in a moist environment before the organic matter content was quantified by titration with 0.1 M ammonium iron sulfate (Nelson and Sommer, 1982).
Al, Fe, Mn, and Ti contents were extracted with 1:1 H2SO4, while the Si content was extracted with NaOH. The Fe, Al, and Mn concentrations were determined using atomic absorption spectrophotometry, and the Ti and Si concentrations were quantified gravimetrically using colorimetry, as described by Embrapa (2011). Ki and Kr indices were calculated to estimate the degree of soil weathering, where and Kr=1.7. Poorly crystalline (“amorphous”) Fe2O3 (Fe2O3OXA), Al2O3 (Al2O3OXA), and Mn2O3 (Mn2O3OXA) oxides were extracted using oxalic acid and ammonium oxalate, while the “free” iron or crystalline Fe2O3CBD oxides were extracted with sodium citrate-bicarbonate-dithionite (CBD) (Camargo et al., 1986) and were quantified using atomic absorption spectrophotometry.
2.4 Mineralogical analyses
For the mineralogical analysis by X-ray diffraction (XRD), the subsamples were first treated with hydrogen peroxide and then the CBD solution to eliminate the organic matter and iron oxides, respectively (Jackson, 1969). The clay subsamples were saturated with magnesium and glycol or potassium, were subjected to temperatures of 25, 300, and 500 ∘C, and were irradiated with an X-ray diffractometer that was equipped with a copper anode and a nickel filter (Whittig and Allardice, 1986).
2.5 Statistical analyses
The analytical results were evaluated descriptively by considering the mean, maximum, minimum, and coefficient of variation (CV). In addition, multivariate techniques, such as hierarchical cluster analysis (HCA) and principal component analysis (PCA) were used. To avoid interference from the units of measurement in the principal component and clustering analyses, the data were standardized to yield a mean of 0 and a variance of 1. Euclidean distance was adopted as a measure of similarity in the HCA. The set of variables used in the AAH and ACP were organic carbon (OC), pH, Ca, Mg, Al, Al + H, K, P, and oxides of Si, Al, Mn, Fe, and Ti. The variables adopted in the HCA and PCA analyses reached the minimum requirement in terms of Pearson's correlation (p 0.05) when explaining the variation in the data. The maps were made by Surfer 8.0 software (Golden Software, 2002).
3.1 Soil characteristics
In general, the soil samples mostly contained sand, regardless of depth, with the exception of the Nitosols, which predominantly contained clay, due to the heterogeneity of the parent material (Fig. 4a, Tables 1 and S1). Of the 528 soil samples from the 88 sampled areas, 53 % had a clay content that ranged between 200 and 400 g kg−1 (Fig. 4a).
The spatial variability of the pH showed that 71 % of the samples had a pH range between 3 and 4, while 36 % had a pH range between 4 and 5 (Fig. 4b). Aluminum had low variation between the soil types and the geological material (Fig. 4c), which revealed the soils are highly weathered and acidic across the state. In 30 % of the study area, aluminum saturation was above 50 %.
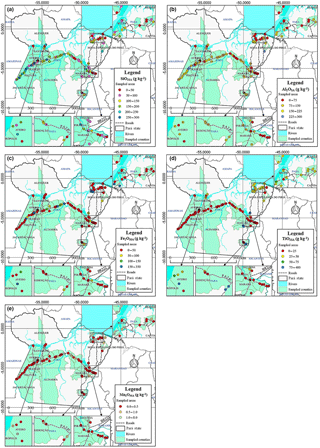
Figure 6Spatial distribution map of Si (a), Al (b), Fe (c), Ti (d), and Mn (e) oxides of the soils of the state of Pará.
Contents of OC ranged from 4 to 45 g kg−1 in the surface layer and was 3 to 50 g kg−1 in the subsurface layer (Fig. 4d). Available P concentration in the surface layer ranged from 2 to 16 mg kg−1 (Table 2) and in the subsurface layer 1 to 3 mg kg−1 (Table S2 and Fig. 5a). In 61 % of the soils, P concentrations were less than 6.6 mg kg−1, which is very low according to Venegas et al. (1999). K, Ca, and Mg concentrations were higher in the surface layer than in the subsurface layer (Table 1), indicating the importance of nutrient cycling for soil fertility in the Amazon due to the low concentration of basic cations in the parent material. The spatial variability in K+ concentration was low in all the samples: in 84 % of the samples (Fig. 5b), Ca2+ was below 12 mmolc kg−1, while 85 % (Fig. 5c) of the samples had a Mg2+ concentration below 4.5 mmolc kg−1; both are considered low (Fig. 5d) (Venegas et al., 1999).
Table 1Physical and chemical properties of the surface layer of soil in the state of Pará.
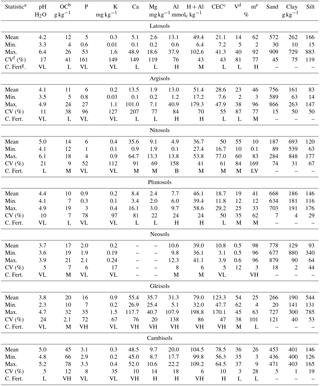
a Descriptive statistic. b Organic carbon. c Cation exchange capacity. d Base saturation ∑ (K, Ca, Mg) ∕ (CEC) ∕ 100. e Aluminum saturation (100 × Al3) ∕ CEC). f Coefficients of variation. g Classes of soil fertility for the complex cation exchange according to Venegas et al. (1999): VL – very low, L – low, M – medium, H – high, VH – very high.
3.2 Soil oxide compositions
The SiO2 and Al2O3 concentrations were distributed similarly (Fig. 6a and b), with higher levels in the Nitosols and Latosols and lower levels in the Plintosols, Neosols, and Gleisols (Tables 2 and S3). The spatial distribution of Fe and Ti oxides paralleled the spatial distribution of clay (Fig. 6c and d). In 84 % of the soils, Fe and Ti oxide concentration ranged from 1 to 400 and from 1 to 350 g kg−1, respectively, while 16 % of the samples had a concentration above 200 g kg−1. Manganese oxide concentration was low in all the study areas and, similar to Ti oxides, the manganese oxides did not accumulate in accordance with the geological formations (Fig. 6e).
The concentrations of poorly crystalline Fe and Al oxides were highest in the most superficial layer and were uniform across the soil classes, with lower values in the Neosols and Plintosols. The levels of free iron (Fe2O3CBD) were high relative to the poorly crystallized concentration in all soil classes, except for in the Cambisols and Neosols, which had a free iron concentration of less than 30 %. Higher Fe2O3CBD oxide concentration was observed in the Latosols and Nitosols, which represented 52 % and 65 % of the free iron relative to the total iron content, respectively.
The Ki and Kr ratios were low in all soil classes, except for Gleisols. Furthermore, Ki values > 1 suggest a predominance of kaolinite, Ki values < 1 suggest the dominance of oxides, and Ki values > 2 indicate the presence of equal amounts of kaolinite and 2:1 minerals (Soares et al., 2005). The Feo ∕ Fed ratio decreased in the subsurface layer relative to the surface layer.
Table 2Descriptive statistic of the levels of oxides SiO2, Fe2O3, Al2O3, Mn2O3, and TiO2 extracted by alkaline and sulfuric acid attack, of the poorly crystallized forms of Mn2O3, Fe2O3, and free oxides Fe2O3, and the relationships between them in the soil surface layer in the state of Pará.
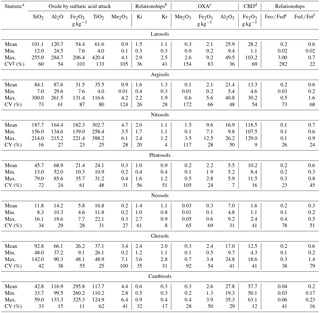
a Descriptive statistic. b Weathering index. c Low crystalline forms extracted by ammonium oxalate acid. d Crystalline Fe extracted by citrate-bicarbonate-dithionite. e Relationships between amorphous and crystalline Fe. f Relationship between levels of citrate-bicarbonate-dithionite Fe and Fe by sulfuric acid attack. g Coefficients of variation.
3.3 Mineralogy of the clay fractions
The mineralogical composition of the clay portion was similar among soil types and at different depths. Thus, only the results for the surface layer of certain soil samples that are representative of each class are presented. The predominant mineral soils from the Amazon were kaolinite, gibbsite, illite, chlorite, and muscovite (Figs. 7, 8, and 9). The silicate mineral in the clay portion of the representative soils was kaolinite, which indicates a high degree of organization because of its structure and range of reactions. Kaolinite was the dominant mineral in the Latosols and Argisols. More diverse mineralogy was identified in the Gleisols (kaolinite, illite, and muscovite), while chlorite was dominant in the Plintosols.
3.4 Multivariate analysis of soil properties
The HCA indicated the formation of four clusters in the surface layer and six clusters in the subsurface layer (Fig. 10a and b). The Neosols, Cambisols, and Nitosols did not form independent clusters at either depth. The chemical and physical attributes of these soils had a low similarity value (Tables 2 and S3). For the subsurface samples, clusters were formed only between the Argisols and Latosols (Fig. 10b). Both soils are dystrophic, with Al predominating in the exchange complex (Tables 1 and S2), have low clay activities, and have a kaolinitic and oxidic mineralogy (Figs. 7, 8, and 9 and Tables 2 and S3).
The PCA results complemented the HCA results for the two depths studied. For the surface layer, the principal components, the first (PC1) and second component (PC2), explained 74 % of the variability in the soil attributes (Fig. 11a). Specifically, PC1 explained 55 % of the total variance, while PC2 explained 19 %. For the subsurface layer, the first two components explained 64 % of the total variability (37 % was explained by the first component and 26 % by the second) (Fig. 11b).
In the subsurface layers, the pH and sand were negatively correlated with the first component, but K, Ca, Mg, and the exchangeable Al and Al2O3OXA were positively correlated. The second component was negatively correlated with Si, Al, Fe, Ti, and Mn oxide concentrations.
4.1 Factors affecting the development and properties of the studied soils
Nitosols that were derived from basalt and diabase had a clay-like texture, which may be related to the parent material because basic rocks form soils with a clay-like texture. Soils with a clay content below 200 g kg−1 are related to the Alter do Chão Formation, Barreiras Group, Maloquinha Intrusive Suite, and Couto Magalhães Formation, all of which are predominantly covered by sedimentary, sandstone, and mudstone rock, with a coarse texture that is rich in quartz (Mendes et al., 2012). Soils that were derived from the same geological formation were found with a sandy or sandy clay texture. These results can be explained by the formation of sandstone and siltstone with a high concentration of Si oxides and coarse granulometry (Camargo et al., 2015). This variation is more related to the characteristics of the parent material than to climate. In areas under the same climatic conditions, pH ranged up to three units, as in the Nitosols and Latosols in the western region of the state.
Low pH values prevailed in soils that were derived from crystalline rocks (argillite and sandstone) with a low concentration of basic cations, such as the Latosols, Argisols, and Plintosols. A pH above 5.0 was prevalent in soils that are developed on basic rocks (basalt and diabase), such as Fluvic Neosols and Gleisols, and anthropogenic soils with an A horizon with relatively restrictive leaching. Soils that are derived from basic rocks, such as basalt and diabase, have a higher concentration of basic cations with less H+ availability when compared with soils derived from crystalline rocks, such as Latosols. The Gleisols and the Fluvic Neosols that develop in alluvium are less acidic due to the deposition of sediments that are rich in OM (Fabian et al., 2014) and in Ca and Mg (Moreira and Fageria, 2009).
Soils with aluminum concentration less than 4 mmolc kg−1 and a pH above 5.5 are considered ideal for the development of most crops, but these conditions are met in only 9 % of the study area. In the states of the Amazonas, Moreira and Fageria (2009) reported that 77 % of the soils had m % values above 50 %. In addition, these authors attributed the low saturation by base in the soil to the high degree of weathering.
The lowest concentrations were found in the Latosols, Argisols, Neosols, and Plintosols as a consequence of climatic conditions (heavy rainfall and high temperatures) that prevail in the Amazon (Fig. 3). High pluvial precipitation and high temperatures contribute to the rapid decomposition of the organic fraction. Clay minerals form complexes with the organic matter to stabilize the organic particles and reduce the rate of mineralization. Low OC content was reported by Steffeens et al. (2011) for the sand fraction in relation to the silt and the clay fraction under different management systems and at different depths.
An OC content above 23 g kg−1 is considered high by Venegas et al. (1999), and this was found in 16 % of the soil samples that were anthropogenic A horizon, Gleisols, Cambisols, and Nitosols. The hydromorphic conditions contributed to the deposition of organic residues, and the high content of clay and oxides protected the OC from microbial decomposition, which leads to the accumulation of carbon on the surface. Another factor contributing to the high OC content in the Gleisols was the strong affinity with organic particles of the amorphous Fe and Al oxides (Fajardo et al., 2009; Quesada et al., 2009).
These values were low in all of the soils but the Gleisols had a high P concentration (16 mg kg−1). The Nitosols and Cambisols had a low available P concentration despite their development on basalt and diabase. Soils developed from basalt usually have a high total P concentration but low available P because of the clay material and the high concentration of Fe and Al oxides. Low levels of available P, Fe, and Al oxides exhibit a high capacity for P immobilization (Hartmann et al., 2014). The high P concentration in the Gleisols, and some of the soil samples with an anthropogenic horizon, may have resulted from the high OC content because the parent materials of these soils are P-poor. In 61 % of the soils, P concentrations were less than 6.6 mg kg−1, which is very low according to Venegas et al. (1999). These concentrations are typical of soils with P deficiencies. The low P concentration could be related to the paucity of P in the parent material combined with pedogenesis. As weathering progresses, P is released from primary minerals, such as apatite, which can be lost by erosion or leached, and thus form secondary phosphates that are bound to Fe and Al oxides with low solubility (Comte et al., 2012).
The low concentrations of K, Ca, and Mg reflect the conditions of soil derived from acidic rocks and from being subjected to intense weathering conditions. Conversely, the high concentrations of K, Ca, and Mg in the lowland soil confer the importance of these soils to food production in the area. The high concentrations of Ca and Mg are related to the samples collected from soils derived from the Diabase Penatecaua Formation and soils in the floodplain areas of the Maecuru Formation and lateritic debris Paleogene covering. The floodplain soils in the Amazon occupy about 200 000 km2 and their fertility is related to the periodic deposition of sediments that is a result of the annual flooding of the rivers in the region (Fajardo et al., 2009).
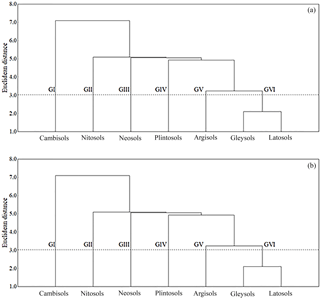
Figure 10Cluster analysis of the classes of soils represented in the state of Pará, in the surface layer (a) and subsurface layer (b).
The higher levels in the Nitosols and Latosols can be explained by the formation of sandstone and siltstone with a high concentration of Si oxides (Camargo et al., 2015). High concentrations of Si and Al were found in the state's western, southern, and northeastern regions, but they were predominantly low in soils of the Barreiras Group (≤200 g kg−1) (Fig. 6a and b). Low concentrations were found in regions with high rainfall. Low oxide contents in the Plintosols and Gleisols were potentially related to the hydromorphism in these environments, which may have led to the removal of these elements from the profile (Camargo et al., 2014). In turn, Al2O3 concentration was highest in the Latosols. The Nitosols and Argisols of Penatecaua Formation had oxidic characteristics because of the advanced degree of weathering. SiO2 concentration was lower than the Al2O3 concentration, which was reflected in the low Ki values. The high solubility and the loss of Si in the soil profile favors the formation of secondary minerals, such as kaolinite and Fe oxides (Darunsontaya et al., 2012).
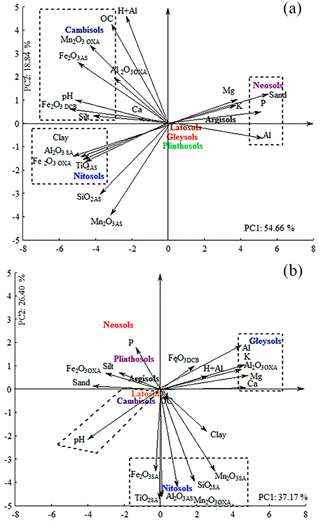
Figure 11Principal component analysis (PC1 and PC2) of the physical, chemical, and mineralogical attributes of the samples from the surface layers (0–0.2 m) (a) and the subsurface layers – diagnostic horizon (b) of the soils of the state of Pará.
In the clay fraction of highly weathered soils, Fe, Ti, and Al oxides had a great influence on CEC and soil acidity (Chandrasekaran and Ravisankar, 2015). Generally, the distribution of Fe oxides was dependent on the parent material and the environmental conditions. The high levels of Fe oxides found in the Nitosols are related to the Diabase Penatecaua Formation that has felsic and mafic rocks, such as basalt and diabase with Fe oxide concentrations higher than that of Si and Al oxides (Dublet et al., 2015). The Nitosols and Cambisols had high Fe concentrations. Ti oxides do not accumulate in specific geological formations, and this may be related to the low levels of Ti in the parent material and the isomorphic substitution of Ti during pedogenesis (Silva et al., 2013).
The high concentration of Mn oxides is related to soils being collected in marsh deposits and Holocene mangroves. These soils are developed in the Guamá and Tapajós river basins. The oxidation-reduction process and the deposition of sediment explain the high concentration of these elements in the floodplain soils when compared to the soils that are developed in the upland regions and on sedimentary rocks (Carvalho Filho et al., 2011). Mn oxide concentration in the floodplain areas was up to 25 times higher than the Mn oxide concentration found in the soils that were developed in well-drained conditions that suffer little influence from a hydrological regime and that naturally have low concentrations of Mn oxides (Thanachit et al., 2006).
The higher concentrations of crystalline Fe and Al oxides in the surface layer were associated with greater amounts of OC in this layer (Table 1) because organic materials inhibit the crystallization of oxides (Camargo et al., 2014). The highest levels of free iron were observed in the Latosols and Nitosols that were developed from basalt, while the lowest levels were in the Cambisols and Neosols and originated from sedimentary rocks.
The low Ki and Kr ratios indicate an advanced state of weathering and the presence of low activity of silicate minerals. The lower Ki values in the soil were due to their oxidic mineralogy (Tables 2 and S3). In the Gleisols, the Ki values were higher, and the mineralogy was dominated by kaolinite, muscovite, and illite (Fig. 9). These results are consistent with those presented by Falesi (1986) and Soares et al. (2005).
The lower Feo / Fed ratio in the subsurface layer was due to high weathering that promotes the crystallization of iron oxides (Paisani et al., 2013) and results in low ratios between the amorphous and crystalline forms. The higher values in the topsoil layer may be related to the contributions of OC and the high exchangeability of the Al, which results in Fe complexation, inhibition of crystallization (Schiavo et al., 2012), and the favoring of the formation of low crystalline oxides.
The small group of minerals that make up the soils reflect the similarities between the parent material that is associated with the bioclimatic conditions (Jiang et al., 2010), temperature, and high precipitation. The mineralogy is consistent with the results of Ki < 2.0, pH < 5.0, and the low FeOXA ∕ FeCBD ratio, which is typical of the mineralogy associated with highly weathered soils (Mareschal et al., 2011), such as those found in the Amazon.
The predominance of kaolinite in the Latosols and Argisols could be related to similar geochemical conditions, including similar parent material with a higher proportion of Al relative to Si, the low mobility of Al in the soil, low pH, good drainage, and heavy rainfall, which promotes the removal of basic cations (Darunsontaya et al., 2012; Paisani et al., 2013) and silica. In this region, mudstone, siltstone, sandstone, and shale are dominant and are mainly derived from the Alter do Chão, Trombetas and Maecuru formations. These geological units support the formation of the Latosols and Argisols throughout the state (Rodrigues et al., 2007).
The occurrence of kaolinite, illite, and muscovite in the Gleisols can be attributed to sediment that is transported to and deposited in the floodplains of the Amazon basin. Poor drainage and the constant deposition of sediment reduces the effects of weathering when compared to well-drained areas that are derived from older sediments and crystalline rocks (Silva et al., 2013). Conversely, the presence of kaolinite in the Gleisols is most likely the result of allochthonous formations. In environments with heavy rainfall, silicate minerals are often distributed to adjacent areas, via the water system, throughout the plains (Furquim et al., 2010).
In the Plintosols, chlorite was dominant and the occurrence in soils of the western Amazonia was reported by Marques et al. (2002). In pedogenetic environments, chlorite is unstable and is present in small quantities (Mareschal et al., 2011). The formation of chlorite is common in soils derived from ultrabasic rocks, even in humid tropical regions where climatic conditions are favorable for weathering and transformation into 1:1 minerals. Although the Plintosols soils are a source of Mg (Lessovaia et al., 2012), the reserves of other basic cations in the Plintosols are limited.
4.2 Multivariate analysis of soil properties
In the surface layer, the Plintosols, Gleisols, Latosols, and Argisols showed poor heterogeneity between the chemical and physical parameters. The ages of the soils, with the origin of the materials, and the different geomorphologies allowed us to explain the chemical variability and the physical attributes of the Neosols, Cambisols, and Nitosols. The Neosols and Cambisols soils are young and dominated by the sand fraction, whereas the Nitosols are old soils (Quesada et al., 2010).
The first component in the surface layer was characterized by the following variables: P, Al, pH, Fe2O3OXA, Mn2O3OXA, Fe2O3CBD, sand, silt, clay, Al, Ti, and Fe oxides. In contrast, the second component was related to the MO, H+, Al, and MnOSA concentrations. The concentration of P and exchangeable Al and the sand contents had positive values in the first component, while the pH, Fe2O3OXA, Mn2O3OXA, Fe2O3CBD, silt, clay, and oxides of Al, Ti, and Fe had negative values. The inverse relationship between the P concentration and the oxide concentration corresponded with the basic principles of soil chemistry because P in tropical soils is strongly adsorbed to oxides and clay minerals, which reduces its availability (Wang et al., 2012).
The predominance of 1:1 minerals (Figs. 7, 8, and 9) contributes to the inverse behavior between acidity and nutrient availability. Acidic soils (Tables 2 and S3) favor the mobility of monovalent and divalent cations that are electrostatically bound to clay minerals, oxides, and the OC (Marques et al., 2004). Climatic conditions in the Amazon (intense rainfall) facilitate the leaching of bases, especially in sandy soils with low CEC.
The PCA produced three separate groups in the surface and subsurface layers that were correlated with the soil classes and attributes. In the surface layer, the P, sand, and exchangeable Al variables were grouped with the Neosols. The P concentration and exchangeable Al concentration is altered by the sand content, and the sand content varies according to the soil class and the depth of the soil profile. The relationships between the P concentration and the sand fraction potentially correspond to the presence of apatite in the sand fraction (Thanachit et al., 2006). This group contained samples from Fluvic Neosols. The transport and deposition of organic matter and sediments rich in apatite (those found in the Amazon basin) contribute to high P concentration in Fluvic Neosols.
The OC, Mn2O3OXA, Fe2O3SA, pH, Fe2O3CBD, and silt contents were correlated with each other in the Cambisols. The pH in humid tropical regions is strongly influenced by the OC content. Organic acids can move, and complex H+ and Al is adsorbed on mineral surfaces, allowing for the occupation of these sites by basic cations to prevent leaching (Fernández-Pazos et al., 2013).
In the Nitosols, the clay, Al2O3SA, Fe2O3OXA, and TiO2SA levels were strongly correlated. The clay fraction of highly weathered soils is predominantly oxidic and contains, for the main part, iron oxides (Ferreira et al., 2003). The aluminum, iron, and titanium oxide concentrations, which had the next highest eigenvalues, indicated a close relationship between these oxides that are potentially derived from a common group of minerals. In addition to the Al2O3SA, Fe2O3OXA, and TiO2SA concentrations in the subsurface, a correlation was found between Mn2O3SA and SiO2SA in the Nitosols. The Nitosols in the state of Pará are derived mainly from basalt and diabase, which is rich in ferromagnesian minerals (Moreira et al., 2009).
No correlations were observed between the attributes of the Latosols, Argisols, Gleisols, and Plintosols in the surface layers (Fig. 11a) due to the different natures of these soils and the different pedogenesis conditions that were found in their surface layers. The absence of correlations between the attributes of these soils may be related to the heterogeneity of the samples, which results in scattered data. A subsurface group was formed with K, Ca, Mg, Al, and Al2O3OXA in the Gleisols (Fig. 11b). The relief of the sample area favors the penetration of water, and basic cations are removed from the profile (surface layer) by natural drainage (Fajardo et al., 2009). These processes explain the high levels of bases in the subsurface. In addition, the allochthonous origin of the parent material and less intense weathering contributed to high levels of bases in the Gleisols (Quesada et al., 2010). In the Amazon plain, organic materials and sediments originating from the Andes, with high concentrations of basic elements, are transported and deposited (Fageria and Moreira, 2009), which favor the accumulation of basic cations in the Gleisols and Fluvic Neosols.
The soils in the state of Pará have low fertility with a predominance of aluminum in the exchange complex, except for the eutrophic Fluvic Neosols and Red Nitosols. Low soil fertility is directly related to bioclimatic and biogeochemical conditions in the region. The parent material (predominantly sedimentary rocks), high temperatures, and intense precipitation in this region contribute to low soil fertility. The mineralogy is predominantly kaolinite, but micaceous minerals are found in poorly drained environments. The deposition of sediments from the Andes region contributes to the neoformation of minerals and to the preservation of primary minerals in soils along the Amazon basin, as in the Plintosols, Gleisols, and Fluvic Neosols. From the PCA it was possible to identify the relationship between several soil attributes and the variation of attributes among the soil classes. Based on the PCA, it was possible to identify the relationship between several soil attributes and the variation of other attributes among the soil classes. The low concentrations of Ca, Mg, K, P, and organic matter, associated with the predominant 1:1 silicates and high contents of Fe and Al oxides, may make intensive agriculture in the state of Pará difficult. The adoption of management agricultural techniques that maintain soil cover and include the addition of vegetable residues can be a strategy to increase agricultural yield in the Amazon without decreasing soil quality and to reduce deforestation in the region.
The underlying research can be accessed by Edna Santos de Souza and Anderson Martins de Souza Braz.
The supplement related to this article is available online at: https://doi.org/10.5194/soil-4-195-2018-supplement.
EdSS, ARF, LRFA, and AMdSB performed collection and analysis of soils. EdSS, MCCC, and FJdO conducted data tabulation and statistical analysis. EdSS prepared the manuscript with contributions from all co-authors.
The authors declare that they have no conflict of interest.
Brazil's National Counsel of Technological and Scientific Development
(CNPq) is acknowledged for financial support (481187/2010-3 and 481802/2013-4). The
Brazilian Federal Agency for Support and Evaluation of Graduate Education
(CAPES) is also acknowledged for financial support (PROCAD NF 1551/2007).
Edited by: David Dunkerley
Reviewed by: Colin
Pain and one anonymous referee
Venegas, V. H. A., Novais, R. F., Barros, N. F., Catarutti, R. B., and Lopes, A. S.: Interpretation of soil analysis results, in: Recommendations for use of amendments and fertilizers in Minas Gerais, edited by: Ribeiro, A. C., Guimarães, P. T. G., and Alvarez Venegas, V. H., 5th Edn., Brazil, 25–32, 1999.
Aquino, R. E., Campos, M. C. C., Marques Júnior, J., Oliveira, I. A., Mantovaneli, B. C., and Soares, M. D. R.: Geostatistics in assessment of physical properties in a latossolo (oxisol) under native forest and grassland in Manicoré, Amazonas, Brazil, R. Bras. Ci. Solo., 38, 397–406, https://doi.org/10.1590/S0100-06832014000200004, 2014.
Béliveau, A., Lucotte, M., Davidson, R., Lopes, L. O. C., and Paquet, S.: Early Hg mobility in cultivated tropical soils one year after slash-and-burn of the primary forest, in the Brazilian Amazon, Sci. Total Environ., 407, 4480–4489, https://doi.org/10.1016/j.scitotenv.2009.04.012, 2009.
Bowman, M. S., Soares-Filho, B. S., Merry, F. D., Nepstad, D. C., Rodrigues, H., and Almeida, O. T.: Persistence of cattle ranching in the Brazilian Amazon: A spatial analysis of the rationale for beef production, Land Use Policy, 29, 558–568, https://doi.org/10.1016/j.landusepol.2011.09.009, 2012.
Camargo, L. A., Marques Júnior, J., Pereira, G. T., and Bahia, A. S. R. S.: Clay mineralogy and magnetic susceptibility of Latosols in geomorphic surfaces, Sci. Agric., 71, 244–256, https://doi.org/10.1590/S0103-90162014000300010, 2014.
Camargo, L. A., Marques, J., Barrón, V., Alleoni, L. R. F., Barbosa, R. S., and Pereira, G. T.: Mapping of clay, iron oxide and adsorbed phosphate in Latosols using diffuse reflectance spectroscopy, Geoderma, 251/252, 124–132, https://doi.org/10.1016/j.geoderma.2015.03.027, 2015.
Camargo, O. A., Moniz, A. C., Jorge, J. A., and Valadares, J. M. A. S.: Methods of chemical, physical and mineralogical analysis of soils from the Agronomic Institute of Campinas, Campinas (IAC, Technical Bulletin), 94 pp., 1986.
Carvalho Filho, A., Curi, N., Marques, J. J. G. S. M., Shinzato, E., Freitas, D. A. F., Alvarenga, E., and Massahud, R. T. L. R.: Manganese oxides an iron ore province soils, Minas Gerais, Brazil, R. Bras. Ci. Solo., 35, 793–804, 2011.
Chandrasekaran, A. and Ravisankar, R.: Spatial distribution of physico-chemical properties and function of heavy metals in soils of Yelagiri hills, Tamilnadu by energy dispersive X-ray florescence spectroscopy (EDXRF) with statistical approach, Spectrochim. Acta A, 150, 586–601, https://doi.org/10.1016/j.saa.2015.05.083, 2015.
Comte, I., Davidson, R., Lucotte, M., Carvalho, C. J. R., Oliveira, F. A., Silva, B. P., and Rousseau, G. X.: Physicochemical properties of soils in the Brazilian Amazon following fire-free land preparation and slash-and-burn practices, Agr. Ecosyst. Environ., 156, 108–115, https://doi.org/10.1016/j.agee.2012.05.004, 2012.
Darunsontaya, T., Suddhiprakarn, A., Kheoruenromne, I., Prakongkep, N., and Gilkes, R. J.: The forms and availability to plants of soil potassium as related to mineralogy for upland Latosols and Argisols from Thailand, Geoderma, 170, 11–24, https://doi.org/10.1016/j.geoderma.2011.10.002, 2012.
Dublet, G., Juillot, F., Morin, G., Fritsch, E., Fandeur, D., and Brown Jr., G. E.: Goethite aging explains Ni depletion in upper units of ultramafic lateritic ores from New Caledonia, Geochim. Cosmochim. Ac., 160, 1–15, https://doi.org/10.1016/j.gca.2015.03.015, 2015.
Embrapa: Empresa Brasileira de Pesquisa Agropecuária: Manual of chemical analysis of soils, plants and fertilizers, 2nd Edn., National Research Center of Soil, Rio de Janeiro, Brazil, 2011.
Embrapa: Empresa Brasileira de Pesquisa Agropecuária, Brazilian system of soil classification, 3rd Edn., National Research Center of Soil, Brasília, DF, 353 pp., 2013.
Fabian, C., Reimann, C., Fabian, K., Birke, M., Baritz, R., and Haslinger, E.: GEMAS: Spatial distribution of the pH of European agricultural and grazing land soil, Appl. Geochem., 48, 207–216, https://doi.org/10.1016/j.apgeochem.2014.07.017, 2014.
Fajardo, J. D. V., Souza, L. A. G., and Alfaia, S. S.: Chemical characteristics of floodplain soils in different of land uses system in the Solimões/Amazonas river channel, Acta Amazon., 39, 731–740, https://doi.org/10.1590/S0044-59672009000400001, 2009.
Falesi, I. C.: Soils of the Trans-Amazonian Highway, Bulletin technical, IMPEA, Belém, No. 55, 196 pp., 1986.
FAO: World reference base for soil resources.: a framework for international classification, correlation and communication, 2nd Edn., Roma, IT, FA, IUSS, ISRIC, 128 pp., 2006.
Fernández-Pazos, M. T., Garrido-Rodriguez, B., Nóvoa-Muñoz, J. C., Arias-Estévez, M., Fernández-Sanjurjo, M. J., Núñez-Delgado, A., and Álvarez, E.: Cr (VI) Adsorption and Desorption on Soils and Biosorbents, Water Air Soil Poll., 224, 1366, https://doi.org/10.1007/s11270-012-1366-3, 2013.
Ferreira, B. A., Fabris, J. D., Santana, D. P., and Curi, N.: Iron oxides of sand and silt in a Nitossolo developed from basalt, R. Bras. Ci. Solo., 27, 405–413, https://doi.org/10.1590/S0100-06832003000300002, 2003.
Furquim, S. A. C., Barbiéro, L., Graham, R. C., Queiroz Neto, J. P., Ferreira, R. P. D., and Furian, S.: Neoformation of micas in soils surrounding an alkaline-saline lake of Pantanal wetland, Brazil, Geoderma, 158, 331–342, https://doi.org/10.1016/j.geoderma.2010.05.015, 2010.
Godar, J., Tizado, E. J., and Pokorny, B.: Who is responsible for deforestation in the Amazon? A spatially explicit analysis along the Transamazon Highway in Brazil, Forest Ecol. Manag., 267, 58–73, https://doi.org/10.1016/j.foreco.2011.11.046, 2012.
Golden Software INC: Surfer for windows: realese 8.0, contouring and 3D surface mapping for scientist's engineers user's guide, New York, 714 pp., 2002.
Hartmann, J., Moosdorf, N., Lauerwald, R., Hinderer, M., and West, A. J.: Global chemical weathering and associated P-release – The role of lithology, temperature and soil properties, Chem. Geol., 363, 145–163, https://doi.org/10.1016/j.chemgeo.2013.10.025, 2014.
Jackson, M. L.: Soil chemical analysis: advanced course, Madison, University of Wisconsin, 991 pp., 1969.
Jiang J., Xu, R. K., and Zhao, A. Z.: Comparison of the surface chemical properties of four soils derived from Quaternary red earth as related to soil evolution, Catena, 80, 154–161, https://doi.org/10.1016/j.catena.2009.11.002, 2010.
Lessovaia, S., Dultz, S., Polekhovsky, Y., Krupskaya, V., Vigasina, M., and Melchakova, L.: Rock control of pedogenic clay mineral formation in a shallow soil from serpentinous dunite in the Polar Urals, Russia, Appl. Clay Sci., 64, 4–11, https://doi.org/10.1016/j.clay.2011.11.002, 2012.
Mareschal, L., Nzila, J. D. D., Turpault, M. P., ThongoM'bou, A., Mazoumbou, J. C., Bouillet, J. P., Ranger, J., and Laclau, J. P.: Mineralogical and physico-chemical properties of Ferralic Arenosols derived from unconsolidated Plio-Pleistocenic deposits in the coastal plains of Congo, Geoderma, 162, 159–170, https://doi.org/10.1016/j.geoderma.2011.01.017, 2011.
Marques, J. J., Schulze, D. G., Curi, N., and Mertzman, S. A.: Trace element geochemistry in Brazilian Cerrado soils, Geoderma, 121, 31–43, https://doi.org/10.1016/j.geoderma.2003.10.003, 2004.
Marques, J. J. G. S. M., Teixeira, W. G., Schulze, D. G., and Curi, N.: Mineralogy of soils with unusually high exchangeable Al from the western Amazon Region, Clay Miner., 37, 651–661, https://doi.org/10.1590/S0100-06832006000100007, 2002.
Melo, V. F., Schaefer, C. E. G. R., Fontes, L. E. F., Chagas, A. C., Lemos Júnior, J. B., and Andrade, R.: Physical chemical and mineralogical characteristics of soils from the agricultural colony of Apiaú (Roraima, Amazonia), under different land uses and after burning, R. Bras. Ci. Solo., 30, 1039–1050, https://doi.org/10.1590/S0100-06832006000600013, 2006.
Mendes, A. C., Truckenbrod, W., and Afonso, C. R. N.: Faciological analysis of Alter do Chão Formation (Cretaceous, Amazon basin), near the town of Óbidos, Pará, Brazil, Rev. Bras. Geociênc., 42, 39–57, 2012.
Moreira, A. and Fageria N. K.: Soil Chemical Attributes of Amazonas State, Brazil, Comm. Soil Sci. Plant Anal., 40, 2912–2925, https://doi.org/10.1080/00103620903175371, 2009.
Moreira, F. M. S., Nóbrega, R. S. A., Jesus, E. C., Ferreira, D. F., and Pérez, D. V.: Differentiation in the fertility of Cambisols as related to land use in the upper Solimões river region, western Amazon, Sci. Total Environ., 408, 349–355, https://doi.org/10.1016/j.scitotenv.2009.09.007, 2009.
Nelson, D. W. and Sommers, L. E.: Total carbon, organic carbon and organic matter, in: Methods of Soil Analysis: Chemical Methods, edited by: Sparks, D. L., Part 3. Soil Science Society of America, Madison, 961–1010, 1996.
Pacheco, P.: Agrarian Reform in the Brazilian Amazon: Its Implications for Land Distribution and Deforestation, World Dev., 8, 1337–1347, https://doi.org/10.1016/j.worlddev.2008.08.019, 2009.
Paisani, J. C., Pontelli, M. E., Corrêa, A. C. B., and Rodrigues, R. A. R.: Pedogeochemistry and micromorphology of Latosols – A basis for understanding etchplanation in the Araucárias Plateau (Southern Brazil) in the Late Quaternary, J. South Am. Earth Sci. 48, 1–12, https://doi.org/10.1016/j.jsames.2013.07.011, 2013.
Quesada, C. A., Loyd, J., Anderson, L. O., Fyllas, N. M., Schwarz, M., and Czimczik, E. C. I.: Soils of Amazonia with particular reference to the rainfor sites, Biogeosciences, 6, 3851–3921, https://doi.org/10.5194/bg-8-1415-2011, 2009.
Quesada, C. A., Lloyd, J., Schwarz, M., Patino, S., Baker, T. R., Czimczik, C., Fyllas, N. M., Martinelli, L., Nardoto, G. B., Schmerler, J., Santos, A. J. B., Hodnett, M. G., Herrera, R., Luizão, F. J., Arneth, A., Lloyd, G., Dezzeo, N., Hilke, I., Kuhlmann, I., Raessler, M., Brand, W. A., Geilmann, H., Moraes Filho, J. O., Carvalho, F. P., Araujo Filho, R. N., Chaves, J. E., Cruz Junior, O. F., Pimentel, T. P., and Paiva, R.: Variations in chemical and physical properties of Amazon forest soils in relation to their genesis, Biogeosciences, 7, 1515–1541, https://doi.org/10.5194/bg-7-1515-2010, 2010.
Rodrigues, T. E., Silva, R. C., Silva, B. N. R., Silva, J. M. L., Valente, M. A., Dariva, T. A., Souto de Jesus, A. A., and Venturieri, A.: Characterization, mapping and classification of soils of the area of influence of the BR-163 (Santarém-Cuiabá) and BR-230 (Trans-Amazonian Highway), in the Pará state, Brazil, in: Zoning Ecological-Economic of the area of influence of the BR-163 (Cuiabá-Santarém), edited by: Embrapa, 403–571, 2007.
Schiavo, J. A., Dias Neto, A. H., Pereira, M. G., Rosset, J. S., Secretti, M. L., and Pessenda, L. C. R.: Characterization and classification of soils in the Taquari River basin – Pantanal region, state of Mato Grosso do Sul, Brazil, R. Bras. Ci. Solo., 36, 697–707, https://doi.org/10.1590/S0100-06832012000300002, 2012.
Silva, A. C., Bispo, F. H. A., De Souza, S., Ardisson, J. D., Viana, A. J. S., Pereira, M. C., Costa, F. R., Murad, A., and Fabris, J. D.: Iron mineralogy of a grey Oxisol from the Jequitinhonha River Basin, Minas Gerais, Brazil, Clay Miner., 48, 713–723, https://doi.org/10.1180/claymin.2013.048.5.04, 2013.
Soares, M. R., Alleoni, L. R. F., Vidal-Torrado, P., and Cooper, M.: Mineralogy and ion exchange properties of the particle size fractions of some Brazilian soils in tropical humid areas, Geoderma, 125, 355–367, https://doi.org/10.1016/j.geoderma.2004.09.008, 2005.
Soil Survey Staff: Keys to Soil Taxonomy, 12th Edn., USDA-Natural Resources Conservation Service, Washington, DC.
Souza, R. A., Miziara, F., and Marco Junior, P.: Spatial variation of deforestation rates in the Brazilian Amazon: A complex theater for agrarian technology, agrarian structure and governance by surveillance, Land Use Policy, 30, 915–924, https://doi.org/10.1016/j.landusepol.2012.07.001, 2013.
Steffens, M., Kölbl, A., Schörk, E., Gschrey, B., and Kögel-Knabner, I.: Distribution of soil organic matter between fractions and aggregate size classes in grazed semiarid steppe soil profiles, Plant Soil, 338, 63–81, https://doi.org/10.1007/s11104-010-0594-9, 2011.
Thanachit, S., Suddhiprakarn, A., Kheoruenromne, I., and Gilkes, R. J.: The geochemistry of soils on a catena on basalt at Khon Buri, northeast Thailand, Geoderma, 135, 81–96, https://doi.org/10.1016/j.geoderma.2005.10.010, 2006.
Wang, X., L. Zhang, L., Zhang, H., Wu, X., and Mei, D.: Phosphorus adsorption characteristics at the sediment–water interface and relationship with sediment properties in Fushi reservoir, China, Environ. Earth Sci., 67, 15–22, https://doi.org/10.1007/s12665-011-1476-z, 2012.
Whittig, L. D. and Allardice, W. R.: X-ray diffraction techniques, in: Methods of soil analysis, Part 1 – Physical and mineralogical methods, edited by: Klute, A., 2nd Edn., Madison, Soil Science Society of America, American Society of Agronomy, 331–382, 1986.