the Creative Commons Attribution 4.0 License.
the Creative Commons Attribution 4.0 License.
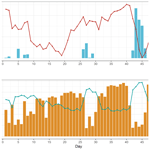
Effects of moss restoration on surface runoff and initial soil erosion in a temperate vineyard
Corinna Gall
Silvana Oldenburg
Martin Nebel
Thomas Scholten
Steffen Seitz
Soil erosion threatens soil fertility and food security worldwide, with agriculture being both a cause and a victim. Vineyards are particularly at risk due to the often steep slopes and detrimental management practices such as fallow interlines and bare soil under the vines. Therefore, the search for alternative management practices becomes vital, and vegetation covers, including mosses, have the potential to reduce soil erosion. However, research on moss restoration as an erosion control method is still in its infancy, and this form of erosion control has never been applied in vineyards. It is thus unclear whether moss restoration can be implemented in vineyards. In this study, the restoration of mosses was investigated by applying artificially cultivated moss mats in a temperate vineyard. The effects of moss restoration on surface runoff and sediment discharge were examined compared to bare soil and cover crops using rainfall simulation experiments (45 mm h−1 for 30 min) with small-scale runoff plots at three measurement times during 1 year (April, June, and October).
Mosses initially showed considerable desiccation in summer, whereupon their growth declined. In October, the mosses recovered and re-established themselves in the vineyard, showing a high level of resistance. Moss restoration significantly reduced surface runoff by 71.4 % and sediment discharge by 75.8 % compared with bare soils. While moss restoration reduced surface runoff slightly more and sediment discharge slightly less compared with cover crops (68.1 % and 87.7 %, respectively), these differences were not statistically significant. Sediment discharge varied seasonally for moss restoration, especially from April to June; this is most likely due to the decline in moss cover and the foliage of the vines in June, as concentrated canopy drip points form on the leaves and woody surfaces of the vines, increasing erosion. Overall, moss restoration proved to be an appropriate and low-maintenance alternative for erosion control, as it requires no mowing or application of herbicides. However, future research should address challenges such as preventing moss mats from drying out in summer; developing methods for large-scale application; and evaluating whether mosses significantly impact soil water content, potentially reducing water availability for vines.
- Article
(5761 KB) - Full-text XML
-
Supplement
(2403 KB) - BibTeX
- EndNote
Soil erosion poses a serious threat to global soil fertility and, consequently, to food security (Amundson et al., 2015). As one of the primary drivers of this issue, agricultural activities exacerbate soil degradation (Borrelli et al., 2017), resulting in soils that can no longer provide important ecosystem services, such as filtering and storing water, providing nutrients, storing carbon, providing habitat for biological activity, and producing biomass (Vogel et al., 2019; FAO and ITPS, 2015). With progression of land use changes and climate change, soil erosion will intensify in the future; thus, the rapid development of effective soil conservation strategies is required (Olsson et al., 2019; Borrelli et al., 2020).
Vineyards are particularly susceptible to soil erosion due to their typically steep slopes; fragile soils, characterised by an extremely basic or acidic pH, loamy or clayey textures, and low soil organic carbon contents; and specific management practices, such as fallow interlines (Rodrigo-Comino, 2018; Prosdocimi et al., 2016a; Rodrigo Comino et al., 2016). For instance, conventional farming in vineyards usually involves weed control practices, such as the application of herbicides and tillage, that leave the soil bare (Biddoccu et al., 2016), which is the most relevant anthropogenic factor for increased soil erosion in viticulture (Rodrigo-Comino et al., 2015, 2018). According to Costantini et al. (2018), who conducted a multidisciplinary study in 19 European and Turkish vineyards, the grape yield can decrease by up to 50 % as a result of soil erosion in viticulture. In addition, the aforementioned study emphasises that soil erosion has degraded essential parameters of soil fertility, the available water capacity, chemical fertility, total nitrogen, and cation exchange capacity, among others. Given the critical role of vineyards in agriculture and their vulnerability to erosion, it is imperative to explore alternative management practices that can effectively mitigate soil erosion.
Vegetation cover is a well-documented natural barrier against soil erosion due to its ability to stabilise the soil and reduce surface runoff (Morgan, 2005). In viticulture, organic management practices that cover the soil surface with vegetation are regularly used, and several studies have shown that these techniques substantially reduce surface runoff and soil erosion (Seeger et al., 2019; Kirchhoff et al., 2017; Biddoccu et al., 2017; Bagagiolo et al., 2018). These practices include allowing spontaneous vegetation to grow, seeding grasses and cover crops (Morvan et al., 2014; Kirchhoff et al., 2017), applying mulching techniques (Prosdocimi et al., 2016b), and planting aromatic herbs (Dittrich et al., 2021). In this way, vegetation cover not only prevents soil loss but also preserves soil organic matter (López-Vicente et al., 2020). Additionally, vegetation cover beneath the vines can positively influence soil fertility by increasing the soil organic carbon content (Fleishman et al., 2021; Marks et al., 2022), which can improve the aggregate structure, although the extent of this effect varies with further soil properties that control the mechanisms of aggregate formation (Bonifacio et al., 2024). In turn, these factors reduce soil erodibility, thereby supporting organic management practices in viticulture.
An argument against organic management practices in vineyards is that the soil-covering vegetation might compete with the vines for water and nutrients (Celette et al., 2009; Dittrich et al., 2021). For example, Celette et al. (2005) found that vine vigour was reduced in a vineyard intercropped with tall fescue grass compared with a conventional vineyard using chemical weed control, attributing this to the competition for not only water but also other soil resources, such as nutrients, or allelopathy effects. The extent of competition likely depends on the climatic conditions of the vineyard location and is probably more pronounced in arid regions compared with humid ones. Nevertheless, in their review on cover crop management and water conservation in vineyards, Novara et al. (2021) recommended the use of cover crops not only in humid but also in drier areas due to their numerous benefits, such as erosion control, increased organic matter, and improved soil fertility, while emphasising that the choice of cover crop species and the timing of termination should be adapted to the average rainfall in dry areas.
An alternative to cover crops to combat soil erosion is moss cover. As poikilohydric plants, mosses cannot actively regulate their water content, relying instead on ambient water availability (Green and Lange, 1994). Attributed in particular to their numerous capillary spaces, which differ depending on the respective species and its life form, mosses are capable of absorbing very high amounts of water, over 2000 % of their dry weight in some species (Proctor et al., 1998; Wang and Bader, 2018; Thielen et al., 2021). In this way, mosses can act as a runoff sink that delays surface runoff (Rodríguez-Caballero et al., 2012). Various studies have already shown that mosses reduce surface runoff (Tu et al., 2022) and effectively mitigate soil erosion (Gall et al., 2022a, 2024a; Juan et al., 2023). Additionally, some studies have demonstrated that mosses can enhance infiltration (Gall et al., 2024a), depending on rainfall intensity and moss species (Tu et al., 2022), and prevent soil evaporation (Thielen et al., 2021; Liu et al., 2022). However, there are also indications of opposite effects; for example, in some cases, mosses have prevented infiltration (Li et al., 2022), especially at low rainfall intensities (Tu et al., 2022), and have increased soil evaporation (Li et al., 2022). Due to its potential beneficial effects on the soil, moss restoration could be a promising new method for sustainable soil management in agricultural settings (Gall et al., 2022b).
However, moss restoration over large areas is demanding and constitutes a growing research field. In recent years, there have been successful efforts to establish mosses in the field under different environmental conditions (Antoninka et al., 2020). For instance, Bu et al. (2018) conducted a plot experiment (1 m × 1 m) in a warm temperate environment in China and achieved a moss cover of 85 % using two dispersal methods (broadcast and spray); this maximum cover was obtained after 30 d with spraying and after 60 d with broadcasting. With respect to moss growth, it was found to be beneficial to apply a nutrient solution, maintain the soil water content at 15 %–25 %, and provide moderate shade in summer. In comparison, Doherty et al. (2020b) developed a moss-colonised burlap fabric for restoration that was placed in the field and was able to establish itself when applied face-down despite drought during the observation period. In addition, there have also been some encouraging experiments on the application of moss restoration strategies in practice, for example, in agriculture (Doherty et al., 2020a) or for post-fire recovery of forests (Grover et al., 2019, 2022), although the moss cover remained low after restoration in all cases. This shows that there are still major challenges in the development of sustainable technologies for moss restoration; thus, these technologies should be the focus of restoration research so that application in practice over large areas becomes possible in the future.
To date, some areas of application, such as viticulture, have not yet been considered for moss restoration, although the approach could be particularly promising for erosion control in vineyards. For example, unlike cover crops, mosses do not require mowing, thereby reducing maintenance efforts and costs. Furthermore, mosses may thrive under conditions in which vascular plants struggle, such as in low-pH soils, on steep slopes, or in managed soils (Gall et al., 2022b; Corbin and Thiet, 2020). However, the sunny, warm, and often dry conditions of vineyards provide an unusual and difficult environment for the establishment of mosses, which is also known from moss restorations studies in drylands (Antoninka et al., 2020). Therefore, it is unclear whether moss restoration will be successful in vineyards. This research gap emphasises the need for studies focusing on the establishment of moss restoration and the effectiveness of mosses with respect to reducing soil erosion in vineyards.
This study aims to address this research gap by investigating the restoration of mosses in a temperate vineyard and evaluating their impact on surface runoff and sediment discharge. The following two hypotheses are formulated:
-
Mosses will begin to establish themselves in the vineyard after being introduced into the field.
-
Moss restoration reduces surface runoff and sediment discharge compared with cover crops and bare soil.
With this research, we want to contribute to the understanding of mosses as a practicable erosion control measure and provide practical knowledge for the management of vineyards to prevent erosion.
2.1 Study site
The study took place in a vineyard south of Fellbach, southwestern Germany, approximately 10 km northeast of Stuttgart (Fig. 1). The vineyard produces the Lemberger grape variety, and the soil between the vines is continuously covered with cover crops such as Lolium perenne, Trifolium repens, Trisetum flavescens, and Achillea millefolium. The study site is located at an altitude of 324 m a.s.l. (metres above sea level) at the foot of the Kappelberg (469 m a.s.l.); comprises flat slopes of 5°; and is part of the Keuper Uplands (Keuperbergland), which consist of Triassic hills stratified by sandstones, marlstones, and claystones (Geyer et al., 2023). A Mollic Anthrosol (Relocatic), which is typically formed in vineyards by deep ploughing, was identified as a soil type (IUSS Working Group WRB, 2022). Mixed samples of the topsoil (0–5 cm) and subsoil (approx. 40 cm) were taken to describe general soil characteristics (Table 1). An agrometeorological station in the immediate vicinity of the study site (48.80158° N, 9.28113° E) revealed an average annual temperature of 11.5 °C between 2007 and 2023, while the average annual precipitation over the same period was 668.3 mm (Agrarmeteorologie Baden-Württemberg, 2024b).
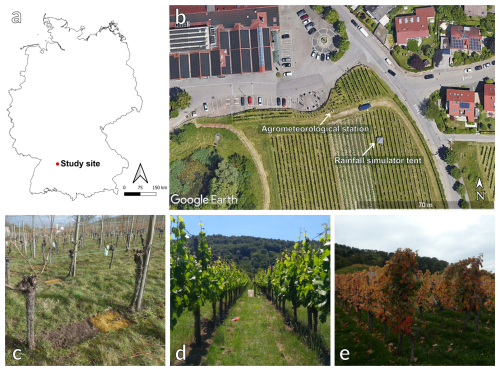
Figure 1Location map and overview of the study site during different seasons. (a) Location of the study site in southwestern Germany (© GeoBasis-DE/BKG 2024, data modified). (b) Google Earth aerial photo of the vineyard with the locations of the rainfall simulator tent and the agrometeorological station (© Google Earth 2022 Image Landsat/Copernicus). (c) Installation of the moss mats on 17 February 2022. (d) The vineyard during the second rainfall simulator experiment on 15 June 2022. (e) The vineyard during the third rainfall simulator experiment on 24 October 2022.
2.2 Field methods
2.2.1 Treatment preparation
The treatments were established on 17 February 2022, within the study site's vine rows. In total, there were three treatments, each with four replicates. The three treatments were moss restoration (moss), bare soil (bare), and cover crop (grass).
The bare treatment was set up by completely weeding the soil. Due to vegetation growth, this procedure had to be repeated before each rainfall simulation experiment, although the soil surface was kept intact to avoid influencing soil erosion processes. This regular weeding maintained a minimal vegetation cover (2 %–20 %), leaving only cut grass tufts and mosses.
The grass treatment utilised the existing planted cover crops without additional preparation, which comprised mainly grasses but also other vascular plants and a few moss species underneath. Common species included Lolium perenne, Trifolium repens, Trisetum flavescens, and Achillea millefolium (identified using Jäger and Werner, 2005).
The moss treatment used artificially grown moss mats with a mixture of mosses (Amblystegium serpens (Hedw.) Schimp., Brachythecium rutabulum (Hedw.) Schimp., Funaria hygrometrica Hedw., Homalothecium lutescens (Hedw.) Robins, Oxyrrhynchium hians (Hedw.) Loeske), produced by Reinhold Hummel GmbH+Co.KG, Stuttgart, Germany. Cultures of these moss species were propagated in hydraulic fluid in an in vitro environment and grown on jute fleece so that the moss mats could be easily rolled, transported, and spread in a similar way to rolled turf. The moss treatment was installed by weeding the area, cutting the moss mats to 40 cm × 40 cm sections, laying them on the bare soil, and securing them with a nail in each corner. Each moss mat was initially watered with 0.5 L of water and underwent periodic watering during dry, hot weather to ensure establishment.
2.2.2 Rainfall simulation experiments
To analyse the effect of moss restoration on initial soil erosion and surface runoff, three rainfall simulation experiments were conducted within 1 year, on 13–14 April, 14–15 June, and 24–25 October 2022 (referred to as measurement times). Each rainfall simulation experiment comprised 12 individual rainfall simulations, resulting in a total of 36 rainfall simulations in 1 year. The given dates were chosen to study initial soil erosion across seasons and to monitor the development of the moss mats. The first and second rainfall simulation experiments also assessed the impact of vine foliage on soil erosion: vines were leafless in April but had almost all of their foliage by June. Surface runoff and sediment discharge were measured using microscale runoff plots (ROPs, 40 cm × 40 cm; Seitz, 2015) for each treatment. The portable Tübingen rainfall simulator, modified with a pavilion for wind protection and an adjusted rainfall height of 2 m, was used (Fig. 2). It featured a Lechler 490.808.30.CE nozzle set to a rainfall intensity of 45 mm h−1 for 30 min. Runoff and sediment were collected in 1 L sample bottles. Soil water content was measured with biocrust wetness probes (BWPs) from UP GmbH, Cottbus, Germany, for each rainfall simulation experiment. Therefore, BWPs were placed in the upper 5 mm of the soil surface underneath the respective vegetation. To determine vegetation cover with a photogrammetric survey, perpendicular photos of all ROPs were taken with a digital compact camera (Panasonic DC-TZ91, Osaka, Japan) during each rainfall simulation experiment. Afterwards, the photos were analysed using the grid square method with a digital grid overlay with 100 subdivisions (Belnap et al., 2001). For each subdivision, bare soil and vegetation covers were separated by hue distinction.
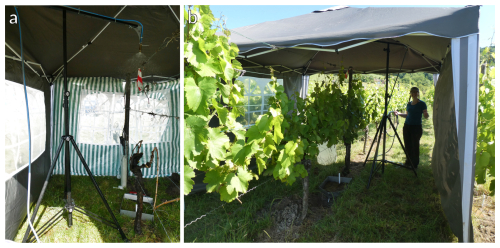
Figure 2Installation of the portable Tübingen rainfall simulator in the vineyard, with the runoff plots directly within the vine rows. (a) The Tübingen rainfall simulator during the rainfall simulation experiment in the vineyard without foliage in April. (b) The Tübingen rainfall simulator during the rainfall simulation experiment in the vineyard with foliage in June.
2.3 Weather conditions after treatment preparation
To evaluate the progress of moss restoration, the weather conditions from the preparation of the treatments to the first rainfall simulation experiment must be taken into account, as shown in Fig. 3 (which was created based on data from the agrometeorological station in Fellbach; Agrarmeteorologie Baden-Württemberg, 2024a, b). A total of 51.1 mm of precipitation and an average air temperature of 6.65 ± 0.15 °C were recorded for the period 48 d from the start of the moss restoration until 1 week before the first rainfall simulation experiment (17 February–5 April 2022). In February and March 2022, precipitation sums were especially low compared with the respective monthly long-term averages of the region (1961–1990, climate station in Waiblingen; February 2022: 34.3 mm; long-term average for February: 48.8 mm; March 2022: 20.3 mm; long-term average for March: 48.8 mm), while the average air temperature was especially high (February 2022: 6.4 °C; long-term average for February: 1.5 °C; March 2022: 7.2 °C; long-term average for March: 5.1 °C). Figure 3 also shows that high daily sums of global radiation were achieved on some days, which is also reflected in the increased hours of sunshine compared with the long-term average (February 2022: 85 h; long-term average for February: 80 h; March 2022: 199 h; long-term average for March: 124 h). For this reason, the average values for relative humidity were below 50 % on some days.
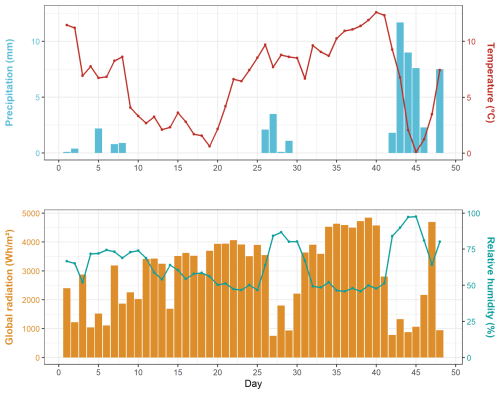
Figure 3Weather diagram for Fellbach with the daily sum of precipitation (mm), average air temperature (°C), daily sum of global radiation (W h m−2), and average relative humidity (%). Displayed are the 48 d from the start of the moss restoration until 1 week before the first rainfall simulation experiment from 17 February to 5 April 2022 (Agrarmeteorologie Baden-Württemberg, 2024a, b).
In addition, the weather conditions for the entire observation period from the beginning of February to the end of October 2022 are presented in Fig. S1 of the Supplement. This information is intended to provide a better understanding of the development of moss restoration over the course of the year.
2.4 Laboratory analysis
After the rainfall simulation experiments, the amount of surface runoff was determined using the sample bottle scales. Surface runoff samples were then evaporated at 40 °C in a compartment drier to weigh the eroded sediment. The following basic soil properties were determined using the mixed soil sample collected prior to the first rainfall simulation experiment: grain size distribution with an X-ray particle size analyser (SediGraph III, Micromeritics, Norcross, GA, USA), soil pH in a 0.01 M CaCl2 solution with a pH meter with SenTix 81 electrodes (WTW, Weilheim in Oberbayern, Germany), soil organic carbon with an elemental analyser (Vario EL II, Elementar Analysensysteme GmbH, Hanau, Germany), and soil bulk density in 100 cm3 core samples using the mass-per-volume method (Blake and Hartge, 1986).
2.5 Data analysis
Data analysis was conducted using R software version 4.0.4 (R Core Team, 2021). Normality was tested with the Shapiro–Wilk test prior to all statistical tests, while homoscedasticity was verified with Levene's test. As our data were not normally distributed and not homoscedastic, the Kruskal–Wallis test was used to screen for significant differences. Dunn's test was applied as a post hoc test, as it allows one to check for significant differences with a small sample size. Significant differences were postulated in all cases at p<0.05. For all mean values described, the standard error was also given (mean ± standard error). The colours selected for all figures are from the “wesanderson” R package (Karthik et al., 2018).
3.1 Development of moss restoration
The percentage vegetation cover per ROP (Fig. 4) was determined for each measurement time and is summarised in Table 2. The bare treatment had the lowest vegetation cover for all measurement times; for this treatment the remaining vegetation was characterised by cut grass tufts in April and June, while some mosses could not be removed without damaging the soil surface in October. For the grass treatment, the vegetation cover was 100 % for all measurement times, although a noticeably lower growth height of the grasses can be seen in April compared with June and October. The moss treatments dried out considerably in April and June, and the jute fleece under the mosses was still clearly visible at both measurement times. Additionally, the moss cover noticeably decreased from April to June. In October, the jute fleece under the mosses had completely decomposed, the moss cover had increased again, and the mosses appeared green and vital.
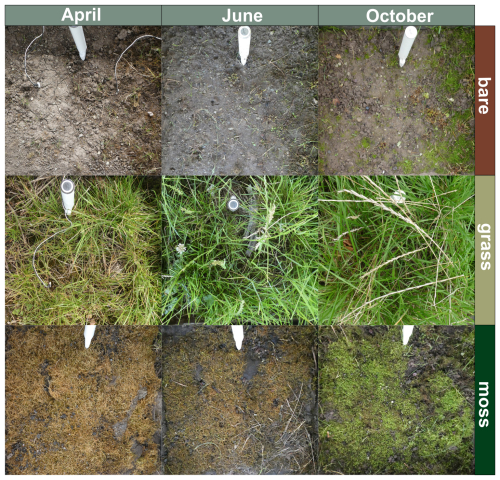
Figure 4Exemplary development of vegetation cover over time in one runoff plot for the three respective treatments.
3.2 Effect of moss restoration on surface runoff
Taking the mean for all measurement times, it can be observed that both the moss and the grass treatment significantly reduced surface runoff (moss: 6.27 ± 1.92 L m−2, p<0.01; grass: 6.99 ± 2.27 L m−2, p<0.01) compared with the bare treatment (21.92 ± 2.52 L m−2), resulting in a decrease in surface runoff of 71.4 % and 68.1 %, respectively. Even though the moss treatment had a slightly lower mean surface runoff than the grass treatment, no significant difference was detected between the two treatments. A separate consideration of the measurement times shows that the surface runoff was influenced by seasonality (Fig. 5). Especially for the moss treatment, there was a significant increase in surface runoff between April (0.91 ± 0.20 L m−2) and October (10.39 ± 4.12 L m−2, p<0.05). Additionally, surface runoff for the moss treatment was significantly lower than for the bare treatment in April, while the reduction in surface runoff was only significant for the grass treatment in June. In October, no significant difference in surface runoff was observed between the three treatments.
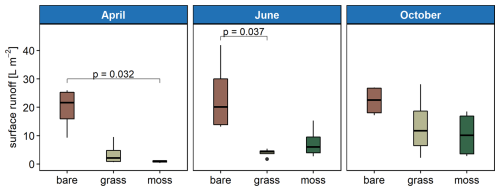
Figure 5Surface runoff (in L m−2) for three treatments and three measurement times (n=4). Lines within the box plots represent median values, while the bottom and top of the box plot show the first and third quartiles, respectively. Whiskers extend up to 1.5 times the interquartile range (IQR) of the data. Outliers are defined as more than 1.5 times the IQR and are displayed as points. The p values presented indicate significant differences between treatments and are based on Dunn's test.
3.3 Effect of moss restoration on sediment discharge
On average, for all measurement times, sediment discharge was highest for bare treatments (139.49 ± 34.57 g m−2), with a significant reduction in the grass treatment to 17.21 ± 3.91 g m−2 (p<0.001) and the moss treatment to 33.74 ± 13.08 g m−2 (p<0.01), corresponding to a decrease in sediment discharge of 87.7 % and 75.8 %, respectively. However, there was no significant difference in sediment discharge between the grass and moss treatments. As for surface runoff, the influence of seasonality was also visible in the sediment discharge separated by measurement time (Fig. 6). In all treatments, there was an increase in sediment discharge between April and June, followed by a reduction in October. The significant increase in sediment discharge in the moss treatment between April (1.31 ± 0.73 g m−2) and June (83.25 ± 24.12 g m−2) (p<0.01) is particularly noteworthy. In April, the moss treatment led to a significant reduction in sediment discharge compared with the bare treatment, while the grass treatment produced significantly lower sediment discharge in June and October compared with the bare treatment but not compared with the moss treatment.
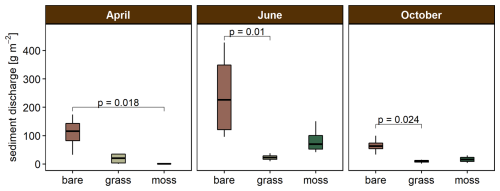
Figure 6Sediment discharge (in g m−2) for three treatments and three measurement times (n=4). Lines within the box plots represent median values, while the bottom and top of the box plot show the first and third quartiles, respectively. Whiskers extend up to 1.5 times the interquartile range (IQR) of the data. Outliers are defined as more than 1.5 times the IQR and are displayed as points. The p values presented indicate significant differences between treatments and are based on Dunn's test.
4.1 Development of moss restoration
The moss mats established themselves more slowly in the vineyard than we originally expected. This can be attributed primarily to the atypical weather conditions observed during the restoration period (Fig. 3). The composition of the moss mats included species that thrive in a variety of habitats, from shaded forest floors to open grasslands (Nebel et al., 2000; Atherton et al., 2010). Moss species growing in these environments can generally tolerate occasional dry periods, but they are not known to be particularly desiccation-tolerant (Proctor et al., 2007). Especially for the initial growing and acclimatisation of the mosses in the vineyard, a high water requirement was expected, and we assumed, based on historical weather data, that March would provide sufficient rainfall for moss establishment (Agrarmeteorologie Baden-Württemberg, 2024b). Instead, the mosses experienced substantial stress due to the unusually dry and warm weather, leading to desiccation and a subsequent decline in moss cover during the summer months. Similar findings from other studies emphasise that water availability is a critical factor for the success of moss restoration efforts (Grover et al., 2022; Doherty et al., 2020b). Although there were extended dry periods in July and August after the second rainfall simulation experiment (Fig. S1), the great resistance of the moss species involved led to a final establishment success. Even though soil protection was less effective in the summer months, a vital and healthy moss cover was re-established from October onwards and fulfilled the expected ecosystem functions. This can be seen as an advantage of moss mats in changing extreme weather situations.
There have already been promising approaches to moss restoration that employ adaptive management strategies to account for weather variability. Bu et al. (2018) showed, for example, that the rapid restoration of moss worked well with sufficient irrigation (70 L per 1 m × 1 m plot in 75 d, in addition to natural rainfall) and shading. Applying this strategy in vineyards would require an adaptation of irrigation practices to ensure adequate water supply during the establishment phase, especially in regions with limited rainfall. While shading is beneficial for moss establishment, it poses a challenge in vineyards, as the vines require sunlight. A simple transfer of these approaches of moss restoration is, therefore, not possible without additional adaptations to the conditions and requirements specific to vineyards.
Additionally, besides sufficient water supply and temperature, many other factors, such as soil pH, nutrients, calcium carbonate content, or soil texture, play an essential role in moss growth (Glime, 2021). This suggests that it may be necessary to develop species-specific solutions for moss restoration in vineyards, taking into account the major constraints on the species involved (Adessi et al., 2021). One promising species is the extremotolerant moss Syntrichia caninervis (Mitt.) Broth., which is known to survive and adapt to extreme conditions, such as severe desiccation and high radiation, including conditions simulated for Mars (Li et al., 2024). S. caninervis is also suitable for moss restoration, as Liu et al. (2021) showed that efficient regeneration is possible for various fragments of gametophytes (leaves, stems, and rhizoids) using peat pellets as substrates. In summary, future research should focus on the development of moss restoration approaches adapted to vineyard conditions, considering alternative restoration techniques and the selection of moss species adapted to the particular challenges posed by these environments.
4.2 Effect of moss restoration on surface runoff
Overall, surface runoff was strongly reduced by moss restoration and cover crops compared with bare soil, although the reduction in runoff was slightly higher for moss restoration, albeit not significantly. Several prior studies, also using rainfall simulation experiments, have shown that organic management practices in vineyards, such as planting grasses as cover crops, can reduce surface runoff compared with bare soils in conventional vineyards (Rodrigo Comino et al., 2016; Seeger et al., 2019). In some cases, however, grass cover had no significant influence on the amount of surface runoff, which was in the same range as for tilled vineyard soils (Telak et al., 2021; Dugan et al., 2023). Morvan et al. (2014) also reported a high variability in surface runoff in vineyard soils covered with grass; this could not be explained by soil type, soil moisture, slope, or agricultural practices, but it could be rationalised by the density of the grass cover. This emphasises the importance of maintaining a dense and consistent grass cover to effectively reduce runoff.
However, according to Dugan et al. (2023), the season also has a significant effect on the hydrological response of vineyard soils, and this was confirmed across all treatments studied, including tilled soils, grass cover, and straw mulch. Similarly, our study found that the reduction in runoff varied seasonally. This phenomenon was also demonstrated in vineyards in Croatia using rainfall simulator experiments, where surface runoff in the wet season (in May) was significantly higher in both tilled and grass-covered treatments compared with the dry season in September (Telak et al., 2021). Biddoccu et al. (2017) also observed this seasonal effect during a 2-year monitoring experiment with natural rainfall in an Italian vineyard. They concluded that runoff primarily occurred in the grass cover treatment due to topsoil saturation, while total annual runoff reduction reached approximately 63 %. Our measurements of the topsoil water content during rainfall simulation experiments also show seasonal differences in water content (Fig. S3), which partly explains the seasonal variation in surface runoff.
The seasonal variation in surface runoff is particularly noticeable with regard to the restoration of moss, which steadily increased the surface runoff from April to October. This can be attributed to the decline in moss cover, on the one hand, and to the delayed decomposition of the jute fleece, on the other hand. We originally assumed that the surface runoff would decrease once the mosses had established themselves at the site. However, on average, the highest surface runoff was measured in October. One possible explanation for this is that, despite the full establishment of mosses in October, soil coverage was still lower compared with April. In addition, it is possible that the jute material itself contributed substantially to runoff reduction, as jute nets are also often used as a geotextile for soil protection, and their runoff and erosion-reducing effect has been demonstrated in several studies (Bhattacharyya et al., 2010; Mitchell et al., 2003). However, Kertész et al. (2007) tested the use of jute mats for erosion control in vineyards and found that surface runoff increased when jute mats were applied. In summary, it is challenging to disentangle the surface runoff effects of the moss and the underlying jute fleece. Therefore, it would be important for future research to specifically investigate the effects of jute fleece alone.
The runoff-reducing effect of mosses has already been confirmed in several studies (Xiao et al., 2015; Tu et al., 2022). However, to the best of our knowledge, no comparable data are available for vineyards, as mosses have not yet been applied for erosion control in this context. The extent of the surface runoff reduction by mosses varies widely, from a 28.8 % reduction in Juan et al. (2023) to a 91 % reduction compared with bare soil in Gall et al. (2024a). However, Gall et al. (2024a) could show that runoff reduction was also strongly influenced by desiccation cracks. In contrast to our results, Bu et al. (2015) measured a runoff reduction of 37.3 % by moss-dominated biocrusts compared with bare soils, while two different grass species alone (Stipa bungeana Trin. and Caragana korshinskii Kom.) reduced surface runoff even more (58.5 % and 90.1 %, respectively). A combination of mosses and the two grasses increased the runoff reduction by just 7.4 % and 5.7 %, respectively. This wide range of runoff reduction also shows that, in addition to moss cover, many other factors influence surface runoff, such as antecedent soil moisture, aggregate structure, soil texture, and many more (Le Bissonnais and Singer, 1993; Le Bissonnais et al., 1995; Knapen et al., 2007).
4.3 Effect of moss restoration on sediment discharge
Moss restoration markedly reduced sediment discharge in the vineyard, but cover crops appeared to reduce sediment discharge to an even greater extent, although the difference was not significant. Similarly, the study by Bu et al. (2015) showed that two different grass species reduced sediment discharge more compared with bare soils (Stipa bungeana Trin. by 95.9 % and Caragana korshinskii Kom. by 99.5 %) than moss-dominated biocrusts (81.0 % erosion reduction). In contrast, Gall et al. (2022a) found that moss-dominated runoff plots reduced sediment discharge by 77 %, while runoff plots dominated by vascular vegetation only mitigated sediment discharge by 59 %, although the difference was not significant. However, it is important to distinguish between moss-dominated biocrusts and moss-covered soils (Weber et al., 2022), as these two types of mosses can likely have different effects on runoff and erosion control due to their very different structure. While biocrusts form in the upper millimetres of the soil and create an encrusted surface, with only a small part of their biomass protruding above the soil surface, mature moss covers grow mainly on top of the soil surface and, depending on the species, are not even attached to the soil and create thick mats or lawns (Weber et al., 2022). For instance, in a soil flume experiment combined with rainfall simulations, Juan et al. (2023) showed that mature moss covers, produced by cultivation, can reduce sediment discharge by 64.87 % compared with bare soils. Due to the diverse life forms of mosses (Bates, 1998), it is also possible that the impact on runoff formation and sediment discharge varies from species to species (Tu et al., 2022; Gall et al., 2024a; Thielen et al., 2021).
Our findings, along with other studies using rainfall simulator experiments, consistently demonstrate that vegetation covers, such as grasses, reduce sediment discharge in vineyards (Rodrigo Comino et al., 2016; Dugan et al., 2023; Seeger et al., 2019; Kirchhoff et al., 2017). While seasonal differences in sediment discharge were observed, grass covers consistently reduced sediment discharge across both dry and wet seasons (Telak et al., 2021). A critical consideration for these organic management strategies is that grasses can compete with vines for water and nutrients, which can negatively impact vineyard productivity (Celette et al., 2005; Ruiz-Colmenero et al., 2011). In comparison, mosses can also absorb a very high amount of water (Thielen et al., 2021; Wang and Bader, 2018), but most species are not able to extract water from the soil, as they do not have roots but, rather, rhizoids that are not designed for water absorption or nutrient uptake (Glime, 2021). This could lead to less competition with the vines. Future research should focus on evaluating the water consumption of mosses in vineyard environments to assess their feasibility as an erosion control strategy.
The seasonal fluctuations in sediment discharge between April and June in the moss restoration can be attributed to the fact that the moss cover decreased significantly during this period and that the vines had almost all of their foliage in June, which was not the case in April. To date, only a few studies have examined the impact of leaves and species-specific plant traits on soil erosion. For example, in a young subtropical forest in China, Seitz et al. (2016) found that trees influence soil erosion based on the species present and their respective functional traits, whereby a particularly high crown cover and leaf area index significantly controlled soil erosion. Investigating species' functional traits is crucial, as they greatly affect throughfall kinetic energy, consequently affecting splash erosion (Seitz et al., 2016; Goebes et al., 2016, 2015).
However, the effect of individual trees or tall plants, such as vines, on soil erosion is still unclear, as (to our knowledge) no existing work has dealt with the effect of vine leaves on soil erosion. This is presumably due to the fact that a large part of the studies using rainfall simulator experiments in vineyards are carried out between vine rows instead of within vine rows (Telak et al., 2021; Rodrigo Comino et al., 2016), where the effect of the leaves is probably smaller. For instance, Neumann et al. (2022) observed that the presence of vines and their canopy interception influenced results in a rainfall simulation experiment in vineyards in the Czech Republic. Using two different-sized rainfall simulators, they measured 1.5 times higher soil loss with the larger simulator, despite 30 %–50 % less runoff, highlighting the complex interplay of factors, including the vines. In our study, the leaf blades of the vines are pointed, which may lead to the formation of particularly large droplets that result in a higher splash effect. For instance, Nanko et al. (2013) showed that leaf geometry is, among other things, decisive for leaf drip drop size distribution. Additionally, a further splash effect became visible on bare soils, as we found drop impact holes on the soil surface after the rainfall simulation experiment. We suspect that large drops have repeatedly formed at structurally mediated woody surface drip points, leading to this severe form of erosion, which was recently reported by Katayama et al. (2023), who described these concentrated points as hotspots of soil erosion in forests.
This study investigated moss restoration in a temperate vineyard, evaluating its impact on surface runoff and sediment discharge. The moss mats were able to establish themselves in a temperate vineyard despite challenging environmental conditions. Due to unexpected dry weather, the mosses initially dried out after restoration in February and recovered in October, albeit with less cover. Therefore, future moss restoration projects should incorporate flexible planning, such as selecting more desiccation-tolerant species or providing additional irrigation during critical periods, to address weather fluctuations. Developing species-specific solutions considering major constraints may be also necessary. The strongest reduction in surface runoff was achieved by moss restoration (71.4 %) and was slightly higher than the reduction by cover crops (68.1 %). Moss restoration also significantly reduced sediment discharge by 75.8 % compared with bare soil, but cover crops reduced sediment discharge more (by 87.7 %).
This study demonstrated that moss restoration can reduce sediment discharge and surface runoff. With improved application methods, mosses could effectively limit soil erosion under vine rows, particularly in steep vineyards or those with challenging parent material that is difficult for vascular plants to colonise. Additionally, mosses require minimal maintenance once established, as they do not need mowing. This characteristic makes them particularly suitable as ground cover under vines, where mowing is impractical and herbicides are commonly used. Consequently, successful moss restoration in viticulture has the potential to reduce the environmentally harmful application of herbicides, although further research is necessary to realise this potential.
The codes used in this study are available from the authors upon request.
The dataset compiled and analysed in this study is available on figshare: https://doi.org/10.6084/m9.figshare.26510614.v1 (Gall et al., 2024b).
The supplement related to this article is available online at https://doi.org/10.5194/soil-11-199-2025-supplement.
CG, StS, and MN designed the experiment. SO, CG, and StS carried out field measurements. SO was responsible for laboratory analyses. SO and CG conducted data analyses. CG prepared the manuscript with contributions from all other co-authors.
The contact author has declared that none of the authors has any competing interests.
Publisher's note: Copernicus Publications remains neutral with regard to jurisdictional claims made in the text, published maps, institutional affiliations, or any other geographical representation in this paper. While Copernicus Publications makes every effort to include appropriate place names, the final responsibility lies with the authors.
This research would not have been possible without the provision of the experimental fields by Marc Jäger, the owner of the vineyard, and we would like to thank him for his great contribution and support. We also thank Gert Joachim Aldinger and the Fellbacher Weingärtner eG for the opportunity to use their facilities during rainfall simulation experiments. Furthermore, we are sincerely grateful to Carla L. Webber, Julia Dartsch, Nicolás Riveras-Muñoz, Larissa Werner, Caspar Hollmann, and all students of the GEO 51 course during the 2022–2023 winter semester for their help with fieldwork and lab work. We also appreciate Sabine Flaiz, Rita Mögenburg, and Peter Kühn for their lab work support. We are grateful to Jesús Rodrigo-Comino and the anonymous reviewer for a very constructive and helpful review. During the preparation of this work, ChatGPT-4 by OpenAI was used to refine the language and grammar of the manuscript in individual cases.
This research has been funded by the Deutsche Forschungsgemeinschaft (DFG SE 2767/2-1, “MesiCrust”) and the German Federal Ministry of Food and Agriculture and the German Federal Ministry for the Environment, Nature Conservation, Nuclear Safety and Consumer Protection via Fachagentur Nachwachsende Rohstoffe e.V. (FNR 2220WK67A4, “AnKliMoos”).
This open-access publication was funded by the Open Access Publication Fund of the University of Tübingen.
This paper was edited by Marta Dondini and reviewed by Jesús Rodrigo-Comino and one anonymous referee.
Adessi, A., De Philippis, R., and Rossi, F.: Drought-tolerant cyanobacteria and mosses as biotechnological tools to attain land degradation neutrality, Web Ecol., 21, 65–78, https://doi.org/10.5194/we-21-65-2021, 2021.
Agrarmeteorologie Baden-Württemberg: Stundenwerte der Globalstrahlung und der relativen Luftfeuchtigkeit an der Wetterstation in Fellbach, Agrarmeteorologie Baden-Württemberg [data set], https://www.wetter-bw.de/Internet/AM/NotesBwAM.nsf/bwweb/353153acec539eb8c1257ca7003c1860?OpenDocument (last access: 3 December 2024), 2024a.
Agrarmeteorologie Baden-Württemberg: Stundenwerte für Lufttemperatur (2 m) und Niederschlag an der Wetterstation in Fellbach, Agrarmeteorologie Baden-Württemberg [data set], https://www.wetter-bw.de/Internet/AM/NotesBwAM.nsf/bwweb/353153acec539eb8c1257ca7003c1860?OpenDocument (last access: 3 December 2024), 2024b.
Amundson, R., Berhe, A. A., Hopmans, J. W., Olson, C., Sztein, A. E., and Sparks, D. L.: Soil and human security in the 21st century, Science, 348, 1261071, https://doi.org/10.1126/science.1261071, 2015.
Antoninka, A., Faist, A., Rodriguez-Caballero, E., Young, K. E., Chaudhary, V. B., Condon, L. A., and Pyke, D. A.: Biological soil crusts in ecological restoration: emerging research and perspectives, Restor. Ecol., 28, 150–159, https://doi.org/10.1111/rec.13201, 2020.
Atherton, I., Bosanquet, S., and Lawley, M.: Mosses and Liverworts of Britain and Ireland – A field guide, 1, British Bryological Society, Plymouth, ISBN 978-0-9561310-1-0, 2010.
Bagagiolo, G., Biddoccu, M., Rabino, D., and Cavallo, E.: Effects of rows arrangement, soil management, and rainfall characteristics on water and soil losses in Italian sloping vineyards, Environ. Res., 166, 690–704, https://doi.org/10.1016/j.envres.2018.06.048, 2018.
Bates, J. W.: Is “Life-Form” a Useful Concept in Bryophyte Ecology?, Oikos, 82, 223–237, https://www.jstor.org/stable/3546962 (last access: 3 December 2024), 1998.
Belnap, J., Kaltenecker, J. H., Rosentreter, R., Williams, J., Leonard, S., and Eldridge, D.: Biological soil crusts: ecology and management, US Department of the Interior, Bureau of Land Management, Denver, Colorado, https://www.ars.usda.gov/ARSUserFiles/6112/biologicalSoilCrusts2.pdf (last access: 8 August 2024), 2001.
Bhattacharyya, R., Smets, T., Fullen, M. A., Poesen, J., and Booth, C. A.: Effectiveness of geotextiles in reducing runoff and soil loss: A synthesis, Catena, 81, 184–195, https://doi.org/10.1016/j.catena.2010.03.003, 2010.
Biddoccu, M., Ferraris, S., Opsi, F., and Cavallo, E.: Long-term monitoring of soil management effects on runoff and soil erosion in sloping vineyards in Alto Monferrato (North–West Italy), Soil Till. Res., 155, 176–189, https://doi.org/10.1016/j.still.2015.07.005, 2016.
Biddoccu, M., Ferraris, S., Pitacco, A., and Cavallo, E.: Temporal variability of soil management effects on soil hydrological properties, runoff and erosion at the field scale in a hillslope vineyard, North-West Italy, Soil Till. Res., 165, 46–58, https://doi.org/10.1016/j.still.2016.07.017, 2017.
Blake, G. R. and Hartge, K. H.: Bulk density, in: Methods of soil analysis, Part 1: physical and mineralogical methods, 2nd Edn., edited by: Arnold, K., American Society of Agronomy, Inc. and Soil Science Society of America, Inc., Madison, 363–375, https://doi.org/10.2136/sssabookser5.1.2ed.c13, 1986.
Bonifacio, E., Said-Pullicino, D., Stanchi, S., Potenza, M., Belmonte, S. A., and Celi, L.: Soil and management effects on aggregation and organic matter dynamics in vineyards, Soil Till. Res., 240, 106077, https://doi.org/10.1016/j.still.2024.106077, 2024.
Borrelli, P., Robinson, D. A., Fleischer, L. R., Lugato, E., Ballabio, C., Alewell, C., Meusburger, K., Modugno, S., Schütt, B., Ferro, V., Bagarello, V., Oost, K. V., Montanarella, L., and Panagos, P.: An assessment of the global impact of 21st century land use change on soil erosion, Nat. Commun., 8, 2013, https://doi.org/10.1038/s41467-017-02142-7, 2017.
Borrelli, P., Robinson, D. A., Panagos, P., Lugato, E., Yang, J. E., Alewell, C., Wuepper, D., Montanarella, L., and Ballabio, C.: Land use and climate change impacts on global soil erosion by water (2015–2070), P. Natl. Acad. Sci., 117, 21994–22001, https://doi.org/10.1073/pnas.2001403117, 2020.
Bu, C., Wu, S., Han, F., Yang, Y., and Meng, J.: The combined effects of moss-dominated biocrusts and vegetation on erosion and soil moisture and implications for disturbance on the Loess Plateau, China, PLOS ONE, 10, e0127394, https://doi.org/10.1371/journal.pone.0127394, 2015.
Bu, C., Li, R., Wang, C., and Bowker, M. A.: Successful field cultivation of moss biocrusts on disturbed soil surfaces in the short term, Plant Soil, 429, 227–240, https://doi.org/10.1007/s11104-017-3453-0, 2018.
Celette, F., Findeling, A., and Gary, C.: Competition for nitrogen in an unfertilized intercropping system: The case of an association of grapevine and grass cover in a Mediterranean climate, Eur. J. Agron., 30, 41–51, https://doi.org/10.1016/j.eja.2008.07.003, 2009.
Celette, F., Wery, J., Chantelot, E., Celette, J., and Gary, C.: Belowground interactions in a vine (Vitis vinifera L.)-tall fescue (Festuca arundinacea Shreb.) intercropping system: water relations and growth, Plant Soil, 276, 205–217, https://doi.org/10.1007/s11104-005-4415-5, 2005.
Corbin, J. D. and Thiet, R. K.: Temperate biocrusts: mesic counterparts to their better-known dryland cousins, Front. Ecol. Environ., 18, 456–464, https://doi.org/10.1002/fee.2234, 2020.
Costantini, E. A. C., Castaldini, M., Diago, M. P., Giffard, B., Lagomarsino, A., Schroers, H.-J., Priori, S., Valboa, G., Agnelli, A. E., Akça, E., D'Avino, L., Fulchin, E., Gagnarli, E., Kiraz, M. E., Knapič, M., Pelengić, R., Pellegrini, S., Perria, R., Puccioni, S., Simoni, S., Tangolar, S., Tardaguila, J., Vignozzi, N., and Zombardo, A.: Effects of soil erosion on agro-ecosystem services and soil functions: A multidisciplinary study in nineteen organically farmed European and Turkish vineyards, J. Environ. Manage., 223, 614–624, https://doi.org/10.1016/j.jenvman.2018.06.065, 2018.
Dittrich, F., Iserloh, T., Treseler, C.-H., Hüppi, R., Ogan, S., Seeger, M., and Thiele-Bruhn, S.: Crop diversification in viticulture with aromatic plants: effects of intercropping on grapevine productivity in a steep-slope vineyard in the Mosel area, Germany, Agriculture, 11, 95, https://doi.org/10.3390/agriculture11020095, 2021.
Doherty, K., Bowker, M. A., Durham, R. A., Antoninka, A., Ramsey, P., and Mummey, D.: Adapting mechanized vascular plant seed dispersal technologies to biocrust moss restoration, Restor. Ecol., 28, 25–31, https://doi.org/10.1111/rec.12998, 2020a.
Doherty, K. D., Grover, H. S., Bowker, M. A., Durham, R. A., Antoninka, A. J., and Ramsey, P. W.: Producing moss-colonized burlap fabric in a fog chamber for restoration of biocrust, Ecol. Eng., 158, 106019, https://doi.org/10.1016/j.ecoleng.2020.106019, 2020b.
Dugan, I., Bogunovic, I., and Pereira, P.: Soil management and seasonality impact on soil properties and soil erosion in steep vineyards of north-western Croatia, J. Hydrol. Hydromech., 71, 91–99, https://doi.org/10.2478/johh-2022-0038, 2023.
FAO and ITPS: Status of the World's Soil Resources (SWSR) – Main Report, Food and Agriculture Organization of the United Nations and Intergovernmental Technical Panel on Soils, Rome, Italy, https://www.fao.org/3/i5199e/i5199e.pdf (last access: 8 August 2024), 2015.
Fleishman, S. M., Bock, H. W., Eissenstat, D. M., and Centinari, M.: Undervine groundcover substantially increases shallow but not deep soil carbon in a temperate vineyard, Agr. Ecosyst. Environ., 313, 107362, https://doi.org/10.1016/j.agee.2021.107362, 2021.
Gall, C., Nebel, M., Quandt, D., Scholten, T., and Seitz, S.: Pioneer biocrust communities prevent soil erosion in temperate forests after disturbances, Biogeosciences, 19, 3225–3245, https://doi.org/10.5194/bg-19-3225-2022, 2022a.
Gall, C., Ohan, J., Glaser, K., Karsten, U., Schloter, M., Scholten, T., Schulz, S., Seitz, S., and Kurth, J. K.: Biocrusts: Overlooked hotspots of managed soils in mesic environments, J. Plant Nutr. Soil Sc., 185, 745–751, https://doi.org/10.1002/jpln.202200252, 2022b.
Gall, C., Nebel, M., Scholten, T., Thielen, S. M., and Seitz, S.: Water's path from moss to soil Vol. 2: how soil-moss combinations affect soil water fluxes and soil loss in a temperate forest, Biologia, online first, https://doi.org/10.1007/s11756-024-01666-w, 2024a.
Gall, C., Oldenburg, S., Nebel, M., Scholten, T., and Seitz, S.: Effects of moss restoration on soil erosion and soil water content in a temperate vineyard, Figshare [data set], https://doi.org/10.6084/m9.figshare.26510614.v1, 2024b.
Geyer, M., Nitsch, E., and Simon, T.: Geologie von Baden-Württemberg, E. Schweizerbart'sche Verlagsbuchhandlung (Nägele u. Obermiller), Stuttgart, Germany, ISBN 978-3-510-65526-7, 2023.
Glime, J. M.: Volume 1: Physiological Ecology, edited by: Glime, J. M., Michigan Technological University, Michigan, https://digitalcommons.mtu.edu/oabooks/4 (last access: 3 December 2024), 2021.
Goebes, P., Bruelheide, H., Härdtle, W., Kröber, W., Kühn, P., Li, Y., Seitz, S., Oheimb, G. v., and Scholten, T.: Species-specific effects on throughfall kinetic energy in subtropical forest plantations are related to leaf traits and tree architecture, PLOS ONE, 10, e0128084, https://doi.org/10.1371/journal.pone.0128084, 2015.
Goebes, P., Schmidt, K., Härdtle, W., Seitz, S., Stumpf, F., Oheimb, G. v., and Scholten, T.: Rule-based analysis of throughfall kinetic energy to evaluate biotic and abiotic factor thresholds to mitigate erosive power, Prog. Phys. Geogr., 40, 431–449, https://doi.org/10.1177/0309133315624642, 2016.
Green, T. G. A. and Lange, O. L.: Photosynthesis in poikilohydric plants: A comparison of lichens and bryophytes, in: Ecophysiology of photosynthesis, edited by: Schulze, E.-D. and Caldwell, M. M., Springer, New York, 319–341, https://doi.org/10.1007/978-3-642-79354-7_16, 1994.
Grover, H. S., Bowker, M. A., and Fulé, P. Z.: Improved, scalable techniques to cultivate fire mosses for rehabilitation, Restor. Ecol., 28, 1–7, https://doi.org/10.1111/rec.12982, 2019.
Grover, H. S., Bowker, M. A., Fulé, P. Z., Sieg, C. H., and Antoninka, A. J.: Pelletized inoculation of fire mosses in severely burned conifer forests overcomes initial barriers to Bryum argenteum establishment but does not increase cover, Ecol. Eng., 176, 106513, https://doi.org/10.1016/j.ecoleng.2021.106513, 2022.
IUSS Working Group WRB (Ed.): World Reference Base for Soil Resources, International soil classification system for naming soils and creating legends for soil maps, International Union of Soil Sciences, Vienna, Austria, https://wrb.isric.org/files/WRB_fourth_edition_2022-12-18_errata_correction_2024-09-24.pdf (last access: 19 November 2024), 2022.
Jäger, J. E. and Werner, K.: Rothmaler – Exkursionsflora von Deutschland, Band 4: Kritischer Band, Spektrum Akademischer Verlag, München, ISBN 978-3-8274-1496-0, 2005.
Juan, J., Dongdong, L., YuanHang, F., and Pu, L.: Combined effects of moss colonization and rock fragment coverage on sediment losses, flow hydraulics and surface microtopography of carbonate-derived laterite from karst mountainous lands, Catena, 229, 107202, https://doi.org/10.1016/j.catena.2023.107202, 2023.
Karthik, R., Wickham, H., Richards, C., and Bagget, A.: wesanderson: A Wes Anderson palette generator (R package version 0.3.7), CRAN [code], https://cran.r-project.org/web/packages/wesanderson/index.html (last access: 8 August 2024), 2018.
Katayama, A., Nanko, K., Jeong, S., Kume, T., Shinohara, Y., and Seitz, S.: Short communication: Concentrated impacts by tree canopy drips – hotspots of soil erosion in forests, Earth Surf. Dynam., 11, 1275–1282, https://doi.org/10.5194/esurf-11-1275-2023, 2023.
Kertész, Á., Tóth, A., Szalai, Z., Jakab, G., Kozma, K., Booth, C. A., Fullen, M. A., and Davies, K.: Geotextile as a tool against soil erosion in vineyards and orchards, Sustainable Development and Planning III, 102, 9, https://doi.org/10.2495/sdp070592, 2007.
Kirchhoff, M., Rodrigo-Comino, J., Seeger, M., and Ries, J. B.: Soil erosion in sloping vineyards under conventional and organic land use managements (Saar-Mosel Valley, Germany), Cuadernos de Investigación Geográfica, 43, 119–140, https://doi.org/10.18172/cig.3161, 2017.
Knapen, A., Poesen, J., Govers, G., Gyssels, G., and Nachtergaele, J.: Resistance of soils to concentrated flow erosion: A review, Earth-Sci. Rev., 80, 75–109, https://doi.org/10.1016/j.earscirev.2006.08.001, 2007.
Le Bissonnais, Y. and Singer, M. J.: Seal formation, runoff, and interrill erosion from seventeen California soils, Soil Sci. Soc. Am. J., 57, 224–229, https://doi.org/10.2136/sssaj1993.03615995005700010039x, 1993.
Le Bissonnais, Y., Renaux, B., and Delouche, H.: Interactions between soil properties and moisture content in crust formation, runoff and interrill erosion from tilled loess soils, Catena, 25, 33–46, https://doi.org/10.1016/0341-8162(94)00040-L, 1995.
Li, S., Bowker, M. A., and Xiao, B.: Impacts of moss-dominated biocrusts on rainwater infiltration, vertical water flow, and surface soil evaporation in drylands, J. Hydrol., 612, 128176, https://doi.org/10.1016/j.jhydrol.2022.128176, 2022.
Li, X., Bai, W., Yang, Q., Yin, B., Zhang, Z., Zhao, B., Kuang, T., Zhang, Y., and Zhang, D.: The extremotolerant desert moss Syntrichia caninervis is a promising pioneer plant for colonizing extraterrestrial environments, Innovation, 5, 100657, https://doi.org/10.1016/j.xinn.2024.100657, 2024.
Liu, X., Zhou, P., Li, X., and Zhang, D.: Propagation of desert moss Syntrichia caninervis in peat pellet: a method for rapidly obtaining large numbers of cloned gametophytes, Plant Methods, 17, 42, https://doi.org/10.1186/s13007-021-00740-7, 2021.
Liu, Z., Chen, R., Qi, J., Dang, Z., Han, C., and Yang, Y.: Control of mosses on water flux in an alpine shrub site on the Qilian Mountains, Northwest China, Plants, 11, 3111, https://doi.org/10.3390/plants11223111, 2022.
López-Vicente, M., Calvo-Seas, E., Álvarez, S., and Cerdà, A.: Effectiveness of cover crops to reduce loss of soil organic matter in a rainfed vineyard, Land, 9, 230, https://doi.org/10.3390/land9070230 2020.
Marks, J. N. J., Lines, T. E. P., Penfold, C., and Cavagnaro, T. R.: Cover crops and carbon stocks: how under-vine management influences SOC inputs and turnover in two vineyards, Sci. Total Environ., 831, 154800, https://doi.org/10.1016/j.scitotenv.2022.154800, 2022.
Mitchell, D. J., Barton, A. P., Fullen, M. A., Hocking, T. J., Zhi, W. B., and Yi, Z.: Field studies of the effects of jute geotextiles on runoff and erosion in Shropshire, UK, Soil Use Manage., 19, 182–184, https://doi.org/10.1111/j.1475-2743.2003.tb00301.x, 2003.
Morgan, R. P. C.: Soil Erosion and Conservation, 3, Blackwell Publishing, Oxford, ISBN 140514467X, 2005.
Morvan, X., Naisse, C., Malam Issa, O., Desprats, J. F., Combaud, A., and Cerdan, O.: Effect of ground-cover type on surface runoff and subsequent soil erosion in Champagne vineyards in France, Soil Use Manage., 30, 372–381, https://doi.org/10.1111/sum.12129, 2014.
Nanko, K., Watanabe, A., Hotta, N., and Suzuki, M.: Physical interpretation of the difference in drop size distributions of leaf drips among tree species, Agr. Forest Meteorol., 169, 74–84, https://doi.org/10.1016/j.agrformet.2012.09.018, 2013.
Nebel, M., Philippi, G., Ahrens, M., Holz, I., Sauer, M., and Schoepe, G.: Die Moose Baden-Württembergs, Band 1: Bryophytina I, Andreaeales bis Funariales, Eugen Ulmer Verlag, Stuttgart, ISBN 9783800135271, 2000.
Neumann, M., Kavka, P., Devátý, J., Stašek, J., Strouhal, L., Tejkl, A., Kubínová, R., and Rodrigo-Comino, J.: Effect of plot size and precipitation magnitudes on the activation of soil erosion processes using simulated rainfall experiments in vineyards, Front. Environ. Sci., 10, 949774, https://doi.org/10.3389/fenvs.2022.949774, 2022.
Novara, A., Cerda, A., Barone, E., and Gristina, L.: Cover crop management and water conservation in vineyard and olive orchards, Soil Till. Res., 208, 104896, https://doi.org/10.1016/j.still.2020.104896, 2021.
Olsson, L., Barbosa, H., Bhadwal, S., Cowie, A., Delusca, K., Flores-Renteria, D., Hermans, K., Jobbagy, E., Kurz, W., Li, D., Sonwa, D. J., and Stringer, L.: Land degradation, in: Climate Change and Land: An IPCC special report on climate change, desertification, land degradation, sustainable land management, food security, and greenhouse gas fluxes in terrestrial ecosystems, edited by: Shukla, P. R., Skea, J., Calvo Buendia, E., Masson-Delmotte, V., Pörtner, H.-O., Roberts, D. C., Zhai, P., Slade, R., Connors, S., van Diemen, R., Ferrat, M., Haughey, E., Luz, S., Neogi, S., Pathak, M., Petzold, J., Portugal Pereira, J., Vyas, P., Huntley, E., Kissik, K., Belkameci, M., and Malley, J., Intergovernmental Panel on Climate Change (IPCC), Cambridge University Press, https://doi.org/10.1017/9781009157988.006, 2019.
Proctor, M. C. F., Nagy, Z., Csintalan, Z., and Takács, Z.: Water-content components in bryophytes: Analysis of pressure-volume relationships, J. Exp. Bot., 49, 1845–1854, https://doi.org/10.1093/jxb/49.328.1845, 1998.
Proctor, M. C. F., Oliver, M., Wood, A., Alpert, P., Stark, L., Cleavitt, N., and Mishler, B.: Desiccation-tolerance in bryophytes: A review, Bryologist, 110, 595–621, https://doi.org/10.1639/0007-2745(2007)110[595:DIBAR]2.0.CO;2, 2007.
Prosdocimi, M., Cerdà, A., and Tarolli, P.: Soil water erosion on Mediterranean vineyards: A review, Catena, 141, 1–21, https://doi.org/10.1016/j.catena.2016.02.010, 2016a.
Prosdocimi, M., Jordán, A., Tarolli, P., Keesstra, S., Novara, A., and Cerdà, A.: The immediate effectiveness of barley straw mulch in reducing soil erodibility and surface runoff generation in Mediterranean vineyards, Sci. Total Environ., 547, 323–330, https://doi.org/10.1016/j.scitotenv.2015.12.076, 2016b.
R Core Team: R: A language and environment for statistical computing, R Foundation for Statistical Computing [code], https://www.R-project.org/ (last access: 8 August 2024), 2021.
Rodríguez-Caballero, E., Cantón, Y., Chamizo, S., Afana, A., and Solé-Benet, A.: Effects of biological soil crusts on surface roughness and implications for runoff and erosion, Geomorphology, 145, 81–89, https://doi.org/10.1016/j.geomorph.2011.12.042, 2012.
Rodrigo-Comino, J.: Five decades of soil erosion research in “terroir”. The State-of-the-Art, Earth-Sci. Rev., 179, 436–447, https://doi.org/10.1016/j.earscirev.2018.02.014, 2018.
Rodrigo Comino, J., Brings, C., Lassu, T., Iserloh, T., Senciales, J. M., Martínez Murillo, J. F., Ruiz Sinoga, J. D., Seeger, M., and Ries, J. B.: Rainfall and human activity impacts on soil losses and rill erosion in vineyards (Ruwer Valley, Germany), Solid Earth, 6, 823–837, https://doi.org/10.5194/se-6-823-2015, 2015.
Rodrigo Comino, J., Iserloh, T., Lassu, T., Cerdà, A., Keestra, S. D., Prosdocimi, M., Brings, C., Marzen, M., Ramos, M. C., Senciales, J. M., Ruiz Sinoga, J. D., Seeger, M., and Ries, J. B.: Quantitative comparison of initial soil erosion processes and runoff generation in Spanish and German vineyards, Sci. Total Environ., 565, 1165–1174, https://doi.org/10.1016/j.scitotenv.2016.05.163, 2016.
Rodrigo-Comino, J., Novara, A., Gyasi-Agyei, Y., Terol, E., and Cerdà, A.: Effects of parent material on soil erosion within Mediterranean new vineyard plantations, Eng. Geol., 246, 255–261, https://doi.org/10.1016/j.enggeo.2018.10.006, 2018.
Ruiz-Colmenero, M., Bienes, R., and Marques, M. J.: Soil and water conservation dilemmas associated with the use of green cover in steep vineyards, Soil Till. Res., 117, 211–223, https://doi.org/10.1016/j.still.2011.10.004, 2011.
Seeger, M., Rodrigo-Comino, J., Iserloh, T., Brings, C., and Ries, J. B.: Dynamics of runoff and soil erosion on abandoned steep vineyards in the Mosel Area, Germany, Water, 11, 2596, https://doi.org/10.3390/w11122596, 2019.
Seitz, S.: Mechanisms of soil erosion in subtropical chinese forests – Effects of species diversity, species identity, functional traits and soil fauna on sediment discharge, Dissertation, Universitätsbibliothek Tübingen, https://doi.org/10.15496/publikation-8010, 2015.
Seitz, S., Goebes, P., Song, Z., Bruelheide, H., Härdtle, W., Kühn, P., Li, Y., and Scholten, T.: Tree species and functional traits but not species richness affect interrill erosion processes in young subtropical forests, SOIL, 2, 49–61, https://doi.org/10.5194/soil-2-49-2016, 2016.
Telak, L. J., Pereira, P., and Bogunovic, I.: Management and seasonal impacts on vineyard soil properties and the hydrological response in continental Croatia, Catena, 202, 105267, https://doi.org/10.1016/j.catena.2021.105267, 2021.
Thielen, S. M., Gall, C., Ebner, M., Nebel, M., Scholten, T., and Seitz, S.: Water's path from moss to soil: A multi-methodological study on water absorption and evaporation of soil-moss combinations, J. Hydrol. Hydromech., 69, 421–435, https://doi.org/10.2478/johh-2021-0021, 2021.
Tu, N., Dai, Q., Yan, Y., Peng, X., Meng, W., and Cen, L.: Effects of moss overlay on soil patch infiltration and runoff in karst rocky desertification slope land, Water, 14, 3429, https://doi.org/10.3390/w14213429, 2022.
Vogel, H.-J., Eberhardt, E., Franko, U., Lang, B., Ließ, M., Weller, U., Wiesmeier, M., and Wollschläger, U.: Quantitative evaluation of soil functions: Potential and state, Front. Environ. Sci., 7, 164, https://doi.org/10.3389/fenvs.2019.00164, 2019.
Wang, Z. and Bader, M. Y.: Associations between shoot-level water relations and photosynthetic responses to water and light in 12 moss species, AoB Plants, 10, ply034, https://doi.org/10.1093/aobpla/ply034, 2018.
Weber, B., Belnap, J., Büdel, B., Antoninka, A. J., Barger, N. N., Chaudhary, V. B., Darrouzet-Nardi, A., Eldridge, D. J., Faist, A. M., Ferrenberg, S., Havrilla, C. A., Huber-Sannwald, E., Malam Issa, O., Maestre, F. T., Reed, S. C., Rodriguez-Caballero, E., Tucker, C., Young, K. E., Zhang, Y., Zhao, Y., Zhou, X., and Bowker, M. A.: What is a biocrust? A refined, contemporary definition for a broadening research community, Biol. Rev., 97, 1768–1785, https://doi.org/10.1111/brv.12862, 2022.
Xiao, B., Zhao, Y., Wang, Q., and Li, C.: Development of artificial moss-dominated biological soil crusts and their effects on runoff and soil water content in a semi-arid environment, J. Arid Environ., 117, 75–83, https://doi.org/10.1016/j.jaridenv.2015.02.017, 2015.