the Creative Commons Attribution 4.0 License.
the Creative Commons Attribution 4.0 License.
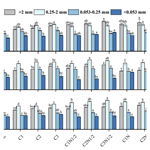
Biochar promotes soil aggregate stability and associated organic carbon sequestration and regulates microbial community structures in Mollisols from northeast China
Jing Sun
Xinrui Lu
Guoshuang Chen
Nana Luo
Qilin Zhang
Xiujun Li
Since the 1950s, heavy plowing of Mollisols, combined with a lack of organic matter intake, has resulted in severe soil degradation in northeast China. The use of biochar in combination with fertilizer is a sustainable method of improving soil quality. In this paper, we conducted field experiments to explore the response of the stability mechanism of the soil aggregate, the dynamic properties of organic carbon, and changes in the microbial community structure to biochar. The biochar input levels were C1, C2, and C3 (9.8, 19.6, and 29.4 Mg C ha−1, respectively), while the nitrogen (N) fertilizer rates were N1/2 (300 kg N ha−1) and N (600 kg N ha−1). Results indicated that biochar combined with N fertilizer effectively increases soil carbon storage and aggregates stability (P<0.05). And C2N treatment increased the aggregate contents of the >2 mm and 0.25–2 mm fractions by 56.59 % and 23.41 %, respectively. The phospholipid fatty acid (PLFA) analysis revealed that microbial community structure was effectively improved with biochar combined with N fertilizer application (P<0.05). The ratio increased by 25.22 % and the gram-positive (Gm+) to gram-negative (Gm−) ratio by 4.65 % under the C2N1/2 treatment. This study concluded that the response of Mollisols to biochar is primarily determined by the interplay of aggregate, organic carbon, and microorganisms. Therefore, the use of biochar combined with N fertilizer might mitigate soil degradation of Mollisols under an optimal application ratio, but the underlying mechanism still requires further exploration. This study will provide a scientific basis for the conservation and sustainable utilization of Mollisols resources.
- Article
(2813 KB) - Full-text XML
-
Supplement
(382 KB) - BibTeX
- EndNote
Mollisols, considered the world's high-yield soils, are typically found in the northern and southern hemispheres in mid-latitudes and constitute about 7 % of the world's soil resource base (Zhang et al., 2018; Eswaran et al., 2011). However, the Mollisols have been severely degraded by intensive continuous cultivation and soil erosion, which has led to the destruction of the agroecosystem and to soil infertility, with far-reaching effects on global climate change (He et al., 2021; Bonfante et al., 2019). Mollisols in China are mainly distributed in Heilongjiang and Jilin provinces, as one of the world's four major black soil regions, which is responsible for the mission of being the “granary” (Mei et al., 2021). The organic matter content of the Mollisols in northeast China decreased by 30 %–50 % from 1980 to 2011, which directly threatened the stability of the regional grain yields (Li et al., 2016). The principal manifestations of soil degradation were a significant decline of soil organic carbon (SOC), destruction in soil aggregation (Zhang et al., 2018), and deterioration of soil structure (Luo et al., 2020; Zhang et al., 2019). The climate (Bottinelli et al., 2017), tillage (Xue et al., 2019), microbial activities (Zhang et al., 2021), and C content all affect the size, number, and composition of soil aggregate (Yin et al., 2018). The SOC can promote the formation of large aggregates in soil; in turn, the increased soil aggregate stability promotes soil SOC sequestration (Zhang et al., 2018). The interaction between carbon sequestration and aggregate stability could reduce soil nutrient loss, improve effective water-holding capacity, increase crop yields, and mitigate global warming through lengthy soil carbon sequestration (He et al., 2021; Scow et al., 2017). It is critical to identify effective strategies to manage the soil in order to enhance its structure and increase its SOC content (Oksana et al., 2022; Plaza et al., 2016). The straw return has been demonstrated to be an effective approach for promoting SOC stabilization, improving soil aggregation, and influencing the structure of microbial communities by using the organic amendment to promote (Xiu et al., 2019). However, direct straw return frequently causes problems, such as creating an adverse soil environment for crop sowing and root elongation (Li et al., 2019) and increasing the number of disease-causing pests and weeds (Wang et al., 2011) during the subsequent growing season. This possibly resulted from the low decomposition rate of returning straw due to long soil freeze durations, especially at higher latitudes in cold Chinese Mollisols with straw decomposition durations of a quarter to 1 year (Wang et al., 2011). Therefore, developing proper straw returns that can increase soil productivity has been a major challenge in this context. Biochar is produced by pyrolyzing biomass at 400–700∘ C in an oxygen-depleted environment (Xiu et al., 2019; Kung et al., 2015). The method has been promoted as a win–win technology for recycling straw while also potentially improving agricultural soils (Islam et al., 2021). Biochar can enhance SOC storage, soil granular structure, and cation exchange capacity (Hu et al., 2020; Mete et al., 2015). For example, Wang et al. (2019) discovered that biochar improved the structural stability of Latosols in southern China. The aggregate mean weight diameter (MWD) and geometric mean diameter (GMD) were improved by 36.3 % and 28.3 %, respectively. Furthermore, Xiu et al. (2019) investigated the effect of corn stalk biochar application dose on Albic soils in northern China. They discovered that a high biochar application level reduced the bulk density of Albic soils by 9.93 % while increasing the pH value. Biochar was also found to significantly improve soil granular structure and organic carbon aggregation (Li et al., 2022). Thus, biochar had a favorable influence on soil quality and aggregation in these acidic soils, which could be attributed to the liming activity of biochar treatments on those acidic soils and the neutralization of the soil pH, which consequently had a significant effect on soil aggregation (Islam et al., 2021). However, there are still some researchers who believe that there is no significant effect (Zhang et al., 2015). Due to the low quantity of biochar minerals and inorganic nitrogen, several studies have indicated that only combination application with other fertilizers can improve soil fertility (Song et al., 2020). Chen et al. (2018b) proposed that an 8-year manure amendment could recover soil nitrogen supplying capacity of lightly eroded Mollisols to natural levels. Fungo et al. (2017) conducted a 2-year field trial in the impoverished Ultisol of western Kenya and found that biochar combined with urea increased MWD by 13 %, whereas biochar alone was less effective. Biochar combined with an organic/inorganic fertilizer has the potential to improve soil fertility (Li et al., 2020) and promote plant growth (Aneseyee et al., 2021; Mete et al., 2015) and carbon storage potential (Wang et al., 2019). Despite these benefits, a quantitative understanding is scarce of how combined effects between biochar and nitrogen fertilizer contribute to soil fertility by modifying microbe–soil interactions in the agroecosystem.
Principal ecological activities in soil, including organic matter formation and decomposition, nutrient cycling, and soil aggregate size redistribution, are all controlled by soil microbial populations (Chen et al., 2022; Trivedi et al., 2017). Phospholipid fatty acids (PLFAs) are the main components of living cell membranes, which play an important role in maintaining cellular fluids, nutrient transportation, and elimination of metabolites, etc. Changes in their components can more accurately express the response of soil microbial biomass and community structure to environmental disturbances (Zhang et al., 2013). The structure of the microbial community is closely related to the change in soil function (Ng et al., 2014). The higher the ratio of soil fungal to bacterial fatty acids, the more sustainable and stable the soil ecosystem (Wang et al., 2017). High bacterial ratios facilitate soil organic carbon accumulation. Soil total nitrogen (TN) content is the main driver of variations in the community composition (Zhang et al., 2021). Wang et al. (2021) discovered that after using biochar in rice fields, the abundance of bacteria (B) and fungi (F) increased by 102 % and 178 %, respectively, which was likely related to an increase in soil total organic carbon (TOC), TN, and rice biomass. According to the study of Chen et al. (2018a), the improvement of microbial community structure by biochar was determined by the and in the paddy soil of central–southern China. In addition, Tian et al. (2016) investigated the mechanism of combined effects between biochar and fertilizer addition on microbial community and soil organic matter cycling in heavy loam soil. It was found that the addition of biochar alone did not significantly improve microbial community structure and that its effect on microbial community structure was dependent on fertilization. The ability of biochar and nitrogen fertilizer to stimulate microbial activity is regulated by the soil conditions and application rates (Palansooriya et al., 2019).
Soil organic carbon sequestration and microbial activity are critical for soil health and quality regulation. However, the beneficial effects of biochar on soil aggregate, associated SOC, and microbial activity have been observed primarily in nutrient-poor acidic soils (e.g., Ultisol and Albic soils), and relevant studies on Mollisols in northeast China have been limited. Furthermore, studies on the combined application of biochar and nitrogen fertilizer are insufficient, limiting the scope of production practice and theory. Therefore, for this study using the northeast Mollisols as a pilot, the objectives are to (1) explore the effects of three biochar gradients combined with N fertilizer on the size, proportion, stability, and carbon content of Mollisols aggregates; (2) explore the influence mechanism of biochar on microbial population structure and identify the major determinants for microbial community composition changes; and (3) develop scientific and effective field management measures for Mollisols by improving the structure of soil aggregates and microbial communities.
2.1 Site description
The field experimental site was located at the test base of the Northeast Institute of Geography and Agroecology, Jilin Province (43∘59′51′′ N, 125∘24′5′′ E). The annual average temperature is 4.6∘ C, the precipitation is 600–700 mm, and the frost-free period during the whole year is 140–150 d. For many years, continuous maize cropping has been carried out in conventional tillage patterns. The soil of the field was classified as Mollisols (Mei et al., 2021). The experimental soil pH was approximately 6.06, TN was 1.26 g kg−1, available phosphorus was 26.78 mg kg−1, available potassium was 133.54 mg kg−1, and organic matter was 26.72 g kg−1. The biochar was created by pyrolyzing corn straw at 400–500∘ C for 4 h under anaerobic conditions. The biochar had a mean particle diameter of 0.003–3.5 mm, a surface area per volume of 0.7 m2 g−1, and an ash concentration of 45 % (biochar particles need to pass through a 2 mm sieve before application). Also, the biochar had a pH of 9.16, a total carbon content of 62.64 %, and a ratio of 39.08. The fertilizer was high-quality urea that was produced by Erdos Yi Ding Ecological Agriculture Development Co. Ltd. The TN was ≥46 %, and the particle size range was 1.18–3.35 mm.
2.2 Field experimental design
A split-zone design was adopted for the field experiment, and three biochar input levels were set: 9.8 Mg ha−1 (C1), 19.6 Mg ha−1 (C2), and 29.4 Mg ha−1 (C3). Nitrogen was applied as a basal fertilizer at rates of 300 kg N ha−1 (N1/2) and 600 kg N ha−1 (N). The CK (no fertilizer) treatment was used as a control. In total, 10 treatments were studied: CK, C1, C2, C3, C1N1/2, C2N1/2, C3N1/2, C1N, C2N, and C3N. Each treatment was performed on a plot with the dimensions 3.9×6.5 m, and each treatment plot had a 1 m buffering zone. A randomized block design was used to conduct the three replicate plots. Biochar with N fertilizer was applied to the soil in April 2013 and 2021, and corn was sown in May 2013 and 2021.
2.3 Soil bulk density and water content
On 29 October 2021, after the corn harvest was complete, soil samples were obtained from each plot using the five-point sampling method, which involved taking 1 kg of soil samples from each plot. Undisturbed soil columns were collected using a soil drill and were placed into zip-locked bags after the removal of plant and animal residues. Some of the soil was promptly refrigerated at 4∘ C for PLFA measurement. A 5 mm mesh screen was used to remove the water-stable soil aggregates from the rest of the sample, which was then allowed to dry naturally. For the determination of the bulk TOC, subsamples of 2 mm soil particles were passed through a 0.15 mm filter after being air-dried. The TOC in the aggregate fractions was determined by K2Cr2O7 titration (Chen et al., 2018b). Next, the surface (0–10 cm) and bottom (10–20, 20–40 cm) soils were sampled with a cutting ring (V=100 cm3) and dried at 105 ∘C for 24 h to measure the soil bulk density and water content using the following formulae:
where X is the field water-holding capacity (%), ρb is the soil bulk density (g cm−3), m is the dry soil weight (g), v is the cutting ring volume (cm3), m2 is the total weight of the cutting ring and soil after 2 h on dry sand, and m1 is the total weight of the cutting ring and soil after drying.
2.4 Soil water-stable aggregate analysis and calculation
In this experiment, the soil aggregates were fractionated utilizing a modified version of the wet sieving method which was given by Zhang et al. (2018). The dry soil sample (100 g) was uniformly coated on automatic vibrating sleeve screens of 2, 0.25, and 0.053 mm in diameter.
The formula for calculating the mass fraction of the water-stable aggregates is as follows:
where Wt is the percentage of the component weight of the ith-sized aggregate.
The MWD and GMD represent the size distribution of the soil aggregates. The larger the values, the more stable the aggregates. The formulas are as follows:
where j is the aggregate size, Xj is the average diameter of the particle size, Wj is the ratio of the aggregate sample weight of each particle size on the screen, Xi is the average diameter of a size i aggregate, Mi is the weight of a size i aggregate, and Mt is the total weight of all the aggregates. The aggregate content was determined as follows:
where R0.25 is the aggregate content (%) with an aggregate size of >0.25 mm, Mr>0.25 is the weight of the soil aggregate that is >0.25 mm, and Mt is the total weight of all the aggregate fractions.
The formula for the soil carbon contribution rate of each aggregate grain size is as follows:
where Cc represents the contribution rate of each particle size aggregate to the carbon level in the soil sample, wi is the weight percent (%) of the i-sized aggregate component, Ci is the organic carbon content of the soil aggregate at size i, and Cs represents the soil TOC content.
2.5 Phospholipid fatty acid analyses
The PLFA extraction method used in this study was described by Luo et al. (2017). The nonadecanoic acid methyl ester (19:0) was employed as an endogenous control. The identified PLFAs were classified into specific microbiota: bacteria (i15:0, a15:0, 15:0, i16:0, 16:1 ω 5, 16:1 ω 9, i17:0, 17:0, a17:0, cy17:0, and cy19:0), fungi (18:2 ω 6c and 18:3 ω 6c), actinomycetes (16:1 ω 7c, 17:1 ω 8c, and 18:1 ω 7c), Gm+ bacteria (i14:0, a15:0, i15:0, i16:0, a17:0, and i17:0), and Gm− bacteria (16:1 ω 7c, 16:1 ω 9c, cy17:0, 17:1 ω 8c, 18:1 ω 7c, and cy19:0) (Luo et al., 2017).
The concentration of the target PLFAs in the sample was calculated as follows:
where CPLFA is the concentration of the target PLFA (nmol g−1), FPLFA is the peak area for the PLFAs, FIS is the area of the internal standard peak, CIS is the internal standard concentration (25 ng µl−1), MPLFA is the molecular weight of the target PLFA, V is the sample dissolution volume (120 µl−1), and m is the soil weight (4 g).
2.6 Statistical analyses
IBM Statistics SPSS 22.0 software was used to test the data normality and homogeneity and principal component analysis (PCA). Significant differences among treatments were calculated by Duncan's multiple range test, with P<0.05. If the data did not meet the criteria, a nonparametric Kruskal–Wallis test was performed to determine the statistical significance. Two-way ANOVA was conducted on a subset of treatments to examine the effects of biochar and organic fertilizer on bulk density, soil water content, soil aggregates, C storage, and microbial community abundance. Canoco 5 (Windows Release 5.02 trial version) software was used for redundancy analysis (RDA).
3.1 Soil physical properties
The biochar had a substantial impact on the soil (0–10 cm) bulk density (P<0.05; Fig. 1), but its coupling effect with N fertilizer was not significant. Also, soil bulk density showed distinct regularities in all profiles and increased with soil depth. The C2N1/2 treatment had the greatest improvement effect of all treatments, and the soil bulk densities of the 0–10, 10–20, and 20–40 cm layers decreased by 13.00 %, 8.62 %, and 2.94 %, respectively. The surface soil (0–10 cm) had the highest moisture content under the CK treatment, while the 10–20 cm soil had the lowest water content. Additionally, there was a substantial positive relationship between biochar application amount and the soil water content in the profile (P<0.01; Fig. 1), with the C3 treatment improving the most when compared to the CK. Furthermore, the soil moisture content increased by 15.12 %–35.39 %. The two-factor ANOVA (Table S1 in the Supplement) showed that biochar significantly improved soil water content (P<0.01) and that biochar contributed significantly to soil bulk density and water content.
3.2 Soil aggregation
The proportions of soil aggregates in descending order were as follows: microaggregates (0.053–0.25 mm), small aggregates (0.25–2 mm), silt and clay (<0.053 mm), and large aggregates (>2 mm; Fig. 2). First, the number of large aggregates fractions was lower in the 20–40 cm soil layer than in the 0–10 cm soil layer. Second, the biochar considerably increased the percentage of large aggregates (11.59 %–50.40 %) while decreasing the percentage of <0.053 mm aggregates (5.12 %–38.66 %). Third, biochar combined with N fertilizer had a synergistic effect, and the proportion of large aggregates continued to increase (38.98 %–56.59 %) before stabilizing.
According to the interactive analyses, N fertilizer had a greater effect on the fraction of large aggregates in the profile (Table S2). The C2N treatment increased the >2 and 0.25–2 mm fractions of soil aggregates by 56.59 % and 23.41 %, respectively. Furthermore, the proportions of aggregates 0.053–0.025 and <0.053 mm decreased by 4.09 % and 43.64 %, respectively. The C2N treatment had the highest growth rate of large aggregates within the 0–10 cm layer, which was 3.66 % and 20.16 % higher than that of the C2N1/2 and C2 treatments, respectively. Furthermore, as soil depth increased, the water-stable aggregates were gradually replaced with aggregates of the size 0.053–0.25 mm (35.95 %–46.42 %).
The MWD, GMD, and R0.25 values increased significantly as the biochar addition ratios increased (Fig. 3). The increasing trend in the stability index was more noticeable after the application of biochar together with fertilizer. Additionally, the R0.25 values of the 0–10, 10–20, and 20–40 cm soil layers increased by 30.33 %, 57.90 %, and 17.70 %, respectively, and the MWD increased by 28.22 %, 50.37 %, and 46.01 %, respectively, in this treatment. The GMD then increased by 18.32 %, 29.43 %, and 17.71 %, respectively.
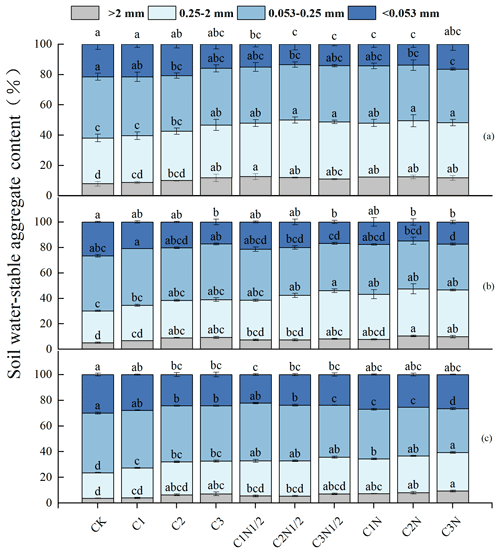
Figure 2The size distribution of the soil aggregates at 0–10 cm (a), 10–20 cm (b), and 20–40 cm (c). The letters indicate significant differences among various treatments (P<0.05). The bars indicate the standard error.
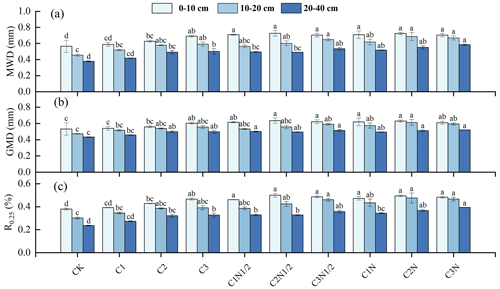
Figure 3The aggregate content with an aggregate size of >0.25 mm (R0.25), mean weight diameter (MWD), and geometric mean diameter (GMD) of the soil aggregates under different treatments. The letters indicate significant differences between the various treatments (P<0.05). The bars indicate the standard error.
3.3 Total organic carbon distribution in the bulk soil and aggregate fractions
The average TOC content of the surface layer was 20.26 % higher than that of the 20–40 cm soil layer (Fig. 4). The TOC content was significantly correlated with the application rates of the biochar and nitrogen fertilizer (P<0.01). Among all the treatments, the C3N treatment in comparison to the CK resulted in the greatest increase in organic carbon content, and the TOC increased by 35.59 %, 30.62 %, and 29.53 % in the soil profile from surface to bottom.
The TOC was significantly associated with aggregate fractions of >2 and 0.25–2 mm but inversely associated with fractions of 0.25–0.053 mm and 0.053 mm aggregates (Fig. 5). We also compared the TOC of the particle size components of the various aggregates under different biochar treatments (Fig. 6a, b, and c) and found that large aggregates had higher carbon content than microaggregates. The C3N1/2 treatment increased the TOC content in the >2 mm, 2–0.25 mm, 0.25–0.053 mm, and <0.053 mm fractions by 36.89 %, 20.39 %, 15.41 %, and 16.14 %, respectively (P<0.05). Furthermore, the 0.25–2 mm aggregate fractions contributed the most to TOC, followed by the >2 mm fractions (Fig. 6d, e, and f). There was no significant change in the contribution of biochar with full N fertilizer (N) to TOC compared to biochar with reduced N fertilizer (N1/2) treatment.
3.4 Microbial community structure
The PLFAs of microorganisms (i.e., bacteria, fungi, actinomycetes, Gm+ bacteria, and Gm− bacteria) in the soil were identified (Fig. 7). The biochar treatment resulted in the highest increases in and proportions of 28.17 % and 7.91 %, respectively (Fig. 7g and h). Also, the two-factor ANOVA (Table S3) showed that N fertilizer effectively altered the abundance of microorganisms, except for fungi and Gm− bacteria (P<0.05). The abundances of the bacteria, fungi, actinomycetes, Gm+, and Gm− in the C2N1/2 treatment increased by 41.89 %, 61.75 %, 75.40 %, 25.46 %, and 19.74 %, respectively. The total PLFAs increased by 52.34 %.
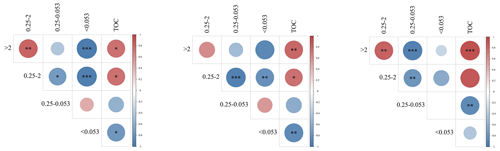
Figure 5The correlation between the total organic carbon (TOC) and the aggregate contents of the different particle sizes in the soil profile (from left to right: 0–10 cm, 10–20 cm, and 20–40 cm). (P<0.001). (P<0.01). ∗ (P<0.05).
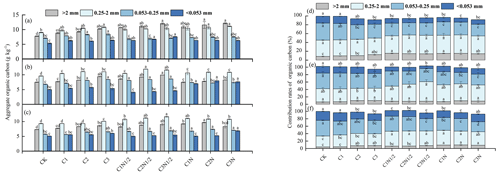
Figure 6The total organic carbon (TOC) levels of the four aggregate fractions: (a) 0–10 cm, (b) 10–20 cm, and (c) 20–40 cm. The contribution rates of the aggregate fractions to the TOC: (d) 0–10 cm, (e) 10–20 cm, and (f) 20–40 cm. The letters indicate significant differences among various treatments (P<0.05) for a given aggregate fraction. The bars indicate the standard error.
The RDA was performed to determine the relationship between soil environmental change and the PLFA response variables (Fig. 8). The two RDA axes were significant (P<0.05), accounting for 94.12 % of the overall variation in the soil microbial characteristics. Soil bulk density was the most significant variable, accounting for 62.61 % of the microbial community characteristics, followed by MWD, soil moisture, TOC, R0.25, and GMD, all of which were significantly correlated with the microbial community composition and explained 15.90 %, 13.42 %, 4.01 %, 2.83 %, and 1.28 % of the various rates of microbial PLFAs, respectively.
The PCA was used to evaluate the effects of various treatments on the soil traits in northeast China (Tables 1, S4). The results showed that the first three principal components (F1−F3) explained 90.13 % of the total variance. The higher the F value, the better the improvement effect, and the C2N1/2 treatment was optimal. The expression of the principal component is as follows:
where X1–X14 represent the bacteria PLFAs, fungi PLFAs, actinomycetes PLFAs, total PLFAs, , Gm+, Gm−, , TOC, R0.25, MWD, GMD, B, and soil moisture, respectively.
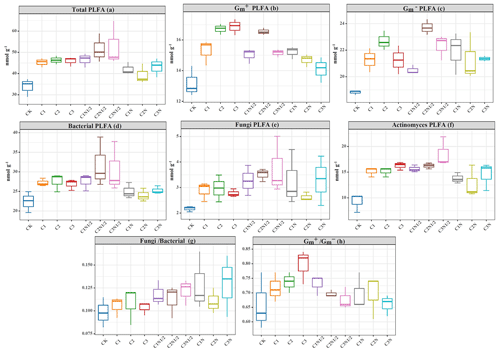
Figure 7The concentration of the (a) total phospholipid fatty acids (PLFAs; nmol g−1), (b) gram-positive bacteria (Gm+) PLFAs, (c) gram-negative bacteria (Gm−) PLFAs, (d) bacteria PLFAs, (e) fungi PLFAs, ((f) actinomycetes PLFAs, (g) ratio of the bacteria PLFAs fungi PLFAs (), and (h) ratio of the Gm+ to Gm− bacteria of the microbial community in the soils under treatment.
4.1 The effects of the biochar and nitrogen fertilizer treatments on soil physical properties
Soil compaction often causes soil structure to be destroyed and soil to be degraded, which is not conducive to the transport of water, air, and solutes in the soil and the propagation of soil microorganisms (Gaia et al., 2020). Our results demonstrated that soil bulk density had a negative correlation with biochar application rate. The C3 treatment reduced soil bulk density by up to 12.69 % (Fig. 1). The bottom soil bulk density was on average 18.88 % higher than that of the surface soil by the biochar amendment, though the improvement in the bottom soil bulk density was not significant (P>0.05), consistent with Xiu et al. (2019). This trend might be due to the unique properties of biochar, such as complex microporous structure, large specific surface area, and light texture (Zhang et al., 2015). The biochar had a slow and gradual effect on the soil improvement. According to Chaganti et al. (2015), the biochar in the soil will gradually migrate to the lower soil over time due to natural factors and human activities. Also, Luo et al. (2020) concluded that biochar was often applied to the surface layer, resulting in a greater decline in the bulk density of the surface soil than the underlying soil. This suggests that biochar has a great benefit in ameliorating soil compaction problems in modern agriculture. Our study also found a considerably strong correlation between the soil water content of the Mollisols and the amount of biochar applied, particularly in the surface soil. The soil water content gradually increased with increasing rate of biochar application. This improvement was the largest during the single application of biochar, with an average increase of 18.07 %. The two-factor ANOVA showed that the increase in soil water content was mainly attributed to biochar, though there was also a synergistic effect of biochar and nitrogen fertilizer on the increase in soil moisture content (Table S1). An et al. (2022) explained this phenomenon using CT scanning techniques, where the addition of biochar reduced soil porosity, reduced pore size, and increased water retention, meaning that water was stored in the smaller pores of the soil and drainage was delayed. The porosity, hydrophilic domains, and huge specific surface area of biochar may be favorable for the improvement of water retention (Leonard et al., 2014). However, some studies contradicted this study, and found either reduced water retention capacity (Madari et al., 2017) or no effect (Baiamonte et al., 2015) after biochar application. The variation in the actions may be attributed to biochar properties, soil texture type, climate change, and experimental design and duration. The input of biochar combined with N fertilizer actively participates in the formation of soil large aggregates, which enhances the soil water-holding capacity and alleviates soil erosion by improving the soil aggregate structure (Gaia et al., 2020; Islam et al., 2021).
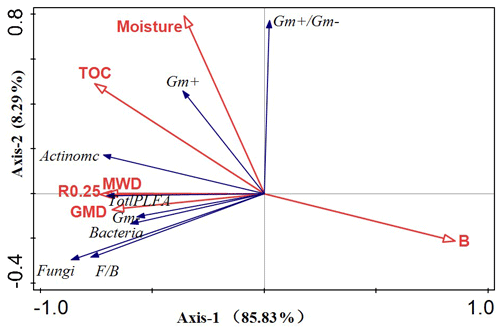
Figure 8A redundancy analysis was used to clarify the relationship between the soil parameter variables and microbial communities. The red arrows represent the explanatory variables (soil physicochemical properties), and the blue vectors represent the response variables (phospholipid fatty acid biomass).
4.2 The effects of biochar and nitrogen fertilizer on soil aggregate distribution and stability
Soil aggregate is essential for the performance of soil functions and is primarily responsible for the formation of the soil structure (Zhang et al., 2018). In this study, biochar increased the formation of large aggregates (>0.25 mm), especially small aggregates (0.25–2 mm), but decreased the number of microaggregates in Mollisols. Grunwald et al. (2016) also confirmed this point by treating Haplic Phaeozem and Gleyic Luvisol with biochar in field experiments. Our findings showed that when biochar was combined with N fertilizer, the fraction of large aggregates steadily increased, while the content of the microaggregates and clay particles decreased (Fig. 2). This suggests that surface hydrophobic–hydrophilic interactions between clay minerals and biochar particles, as well as the biochar ability to integrate with the soil biota and labile carbon, may all contribute to soil aggregation (Joseph et al., 2010).
Furthermore, surface area, microporous structure, and ratio are key biochar features for binding to organo-mineral complexes, an initial stage in aggregate formation and stability (Du et al., 2017). According to the findings of this study, the soil aggregate stability increased by 10.9 %–23.49 %, which is consistent with the findings of a meta-analysis (Peng et al., 2015). The initial low TOC level (26.72 g kg−1) and protracted field experiments (8 years) could explain this. In a laboratory incubation experiment, biochar application increased soil aggregate stability by 2.33 %–6.55 % in Albic soil soils of northeast China (Xiu et al., 2019). According to the MWD (Fig. 3), increased TOC and microbial biomass (Fig. 7) were responsible for the significant increase in aggregation caused by biochar addition. This was also found to be the case in other studies, which found that biochar served as a cementing material, assisting more microaggregates, silt, and clay components to cement together into large aggregates (Xu et al., 2019). The relationship between N input and aggregation can be explained by three possible aspects. Firstly, N fertilizer increased macro-aggregates, and MWD stemmed from N stimulation of root growth (Bai et al., 2021). Roots are a major driver of soil aggregation as they not only constitute the primary source of SOC but also function as physical binding agents for aggregation (Sokol and Bradford, 2019). Second, the N increase of plant photosynthesis generally allows plants to allocate more photosynthates to the roots and their associated mycorrhizal fungi, which may physically facilitate the formation of soil macroaggregate through fine root and hyphal enmeshment of microaggregates (Miller and Jastrow, 1990; Bai et al., 2021). Third, the active functional groups in the N fertilizer adsorb soil Ca2+ and then tend to combine with clay minerals to form a clay–humus complex (Gao et al., 2019). Thus, biochar and N fertilizer application has a longer-term positive influence on aggregate stability. At the same time, it provides a theoretical basis for preventing the thinning of the humus layer and reducing surface runoff and soil erosion (Grunwald et al., 2016). Our findings were in contrast with those of Zhou et al. (2019), who discovered neutral or even antagonistic effects on soil aggregate formation and stabilization due to fewer binding agents produced during the decomposition of recalcitrant biochar. Therefore, the response of soil aggregates to biochar is influenced by the initial TOC, clay content, biochar attributes, and application rate, etc. (Peng et al., 2015). As a result, the evaluation results should be thoroughly examined, considering these factors as well as the effect of time in the field.
According to a two-factor ANOVA (Table S2), there was a synergistic effect of biochar blend with nitrogen fertilizer on increasing the proportion of soil large aggregates, which suggests that biochar with organic fertilizer is beneficial for the stability of soil aggregates. Previous research proposed that biochar combined with N fertilizer promotes crop root growth and improves crop root fungi reproductive capacity. Fungal hyphae and root secretions promote soil aggregation by binding and entangling mineral particles to form large aggregate structures (Islam et al., 2021). Consistently, our data suggest that the increased stability of aggregates results from increased root activity and the significant role of exogenous carbon as a binder for soil particles (Wang et al., 2019).
4.3 The effects of biochar combined with nitrogen fertilizer on the total organic carbon
In this investigation, the TOC level of the Mollisols increased significantly following biochar application, which is consistent with the results of Dong et al. (2016). More recently, Shi et al. (2020) proposed that the combined application of biochar and nitrogen fertilizer was conducive to soil carbon sequestration, with the cumulative mineralization rate of TOC decreasing by 0.6 %–1.1 % when compared to the CK treatment. These findings can be interpreted in three ways. First, the use of biochar increased soil microbial activity (Fig. 7) and crop yields (Lin et al., 2020), thereby promoting further degradation and transformation of the plant residues and increasing TOC. Second, when added to the soil, biochar with a high organic carbon concentration (34.9 %) directly improved the soil's organic matter content. Xiu et al. (2019) found similar results in Albic soil. Third, the enrichment of the organic carbon occluded within the large aggregates and small aggregates (Figs. 5, 6) was higher than that in the microaggregates. Increasing the proportion of large aggregates promoted soil aggregate carbon fixation (Zhang et al., 2018). The fourth explanation is that biochar has a high inert carbon content, which increased the (Fig. 7) in the decomposition of persistent and complex substrates, indicating that carbon accumulation was greater than carbon decomposition (Dong et al., 2020). Thus, biochar effectively prevented the bulk TOC in the Mollisols from decreasing.
In this study, the TOC concentration was positively correlated with the proportion of large aggregate size (Fig. 5), which is consistent with the aggregate hierarchy model proposed by Tisdall (1982). Figure 6 shows that the fractions >0.053 mm had a much higher carbon content than silt and clay, especially in the 0.25–2 mm fraction. Our findings confirmed those of Du et al. (2017) and Dong et al. (2016).
These results showed that the combined application of biochar and a half amount of N fertilizer was more economically efficient. Under the treatment, the carbon of the aggregates <0.053 mm in the 0–20 and 20–40 cm soil layers decreased significantly, which could be explained by the finding of Ying (2018) that N fertilization promoted the mineralization rates of primary organic carbon by affecting the soil microbial community. Overall, there was no advantage in increasing the organic carbon content of the soil aggregates from the increased N fertilizer ratio. This could be due to the high N content, which caused an imbalance in the soil ratio, affecting the breakdown and turnover of soil organic matter (Kimetu et al., 2010). Therefore, biochar, in combination with nitrogen fertilizer as amendments, effectively improves the soil aggregates and carbon sequestration.
4.4 The effects of biochar combined with nitrogen fertilizer on microbial community biomass and structure
Biochar can alleviate the negative effects of soil structure and function degradation on soil microbial activities, particularly when applied in conjunction with nitrogen fertilizer (Oksana et al., 2022). According to published research, biochar addition alone did not change the microbial community structure in spring maize fields or rice paddy fields, but when combined with fertilizer, the structure was changed (Luo et al., 2017; Tian et al., 2016). These findings are consistent with our experimental results. Soil and total PLFA contents were significantly increased following biochar and N fertilizer treatments, which may be accompanied by increased C and N cycling and mineralization rates (Khadem et al., 2021). The higher the ratio of PLFA of soil fungi to bacteria, the more stable the soil ecosystem (Thiet et al., 2006). Compared to Gm− bacteria, Gm+ bacteria generally possess a greater proportion of peptidoglycan, which is a relatively decay-resistant soil organic matter (Zhang et al., 2013). The high bacteria ratio means that SOC accumulation is higher than mineralization (Wang et al., 2017). Therefore, the effect of biochar and organic fertilizer application on microbial community structure may be more inclined to the retention of easily decomposed organic carbon in northeast Mollisols (Jiang et al., 2016). In this study, C2N1/2 treatment had the best improvement effect on microbial community structure. However, when N fertilizer application exceeds crop absorption capacity and soil retention capacity, the excess N may be leached to deep soil and pollute groundwater. Meanwhile, the unbalanced ratio also became the main factor for the significant decline of soil biodiversity (Yuan et al., 2017).
The RDA showed that the biomass of fungi, bacteria, actinomycetes, Gm+, and Gm− was positively related to the fraction of large aggregates and negatively linked to the soil bulk density. The increased fungal abundance has been proposed as an important biological factor in soil aggregate formation (Yuan et al., 2015; Zheng et al., 2020). Previous research has shown that aggregate stability and carbon storage are important prerequisites for enhancing microbial communities (Zhang et al., 2021). Our results showed that the mutual effects of biochar and reduced N fertilizer could effectively affect the abundance of microorganisms, which is attributed to the increased soil content as a result of the applied N fertilizer providing more N sources for microbial decomposition and organic matter utilization (Jia et al., 2020). These findings were consistent with those of Zhang et al. (2021), who discovered that combining biochar with fertilizer significantly increased microbial abundance in the soil sample, implying that the addition of inorganic fertilizer reduced crop N limitation and microbial N immobilization. Furthermore, the TOC and affected the fungal community composition, most likely because fungi were the primary decomposers of TOC (Chen et al., 2013). This conclusion is further confirmed by Sekaran et al. (2019), who found that the amount of soil microbial PLFAs and the ratio of soil carbon to nitrogen were strongly and positively correlated, but biochar and a full dose of N fertilizer had little effect. Based on the sequestration of SOC and the sustainability and stability of the ecosystem, we selected C2N1/2 as the most reasonable biochar ratio.
The field experiments showed that the porous structure of biochar and its carbon source can effectively improve soil structure and carbon storage. The proportion of soil large aggregates and the stability of aggregates were significantly increased. Biochar combined with N fertilizer provided an abundance of living space and nutrients for soil microorganisms, but microbial activity and abundance were limited by carbon input and soil nitrogen availability. The effect of excessive N application was unsatisfactory, which affects the further improvement of soil microbial abundance. This study highlighted that biochar combined with N fertilizer application could be a potential option for the mitigation of soil degradation, reasonable application of N fertilizer, and enhancement of soil carbon storage, which would support sustainable use of Mollisols. In the future, we will further investigate the long-term effects of biochar application on soil C and N cycles in the agroecosystem.
Original data are available upon request.
The supplement related to this article is available online at: https://doi.org/10.5194/soil-9-261-2023-supplement.
JS: investigation, experimentation, data collection and analysis, and manuscript writing. XLu and GC: revision of the manuscript. XLi: concept and design, project administration, and funding acquisition. NL and QZ: investigation, material preparation, and experimentation. The final paper was read and approved by all authors.
The contact author has declared that none of the authors has any competing interests.
Publisher's note: Copernicus Publications remains neutral with regard to jurisdictional claims in published maps and institutional affiliations.
We thank Ezemaduka Anastasia Ngozi (Northeast Institute of Geography and Agroecology, Chinese Academy of Sciences) for her editorial assistance. This work was supported by research grants from the Strategic Priority Research Program of the Chinese Academy of Sciences (grant nos. XDA28020401 and XDA23070500), the National Science Foundation of China (grant no. 41877024), and the Key Laboratory Foundation of Mollisols Agroecology (grant no. 2020ZKHT-05).
This research has been supported by the National Natural Science Foundation of China (grant no. 41877024).
This paper was edited by Raúl Zornoza and reviewed by two anonymous referees.
An, N., Zhang, L., Liu, Y., Shen, S., Li N., Wu, Z., Yang, J., Han, W., and Han, X.: Biochar application with reduced chemical fertilizers improves soil pore structure and rice productivity, Chemosphere, 298, 134304, https://doi.org/10.1016/j.chemosphere.2022.134304, 2022.
Aneseyee, A. B. and Wolde, T.: Effect of biochar and inorganic fertilizer on the soil properties and growth and yield of onion (Allium cepa) in tropical Ethiopia, Sci. World J., 9, 5582697, https://doi.org/10.1155/2021/5582697, 2021.
Bai, T., Wang, P., Ye, C., and Hu, S.: Form of nitrogen input dominates n effects on root growth and soil aggregation: a meta-analysis, Soil Biol. Biochem., 157, https://doi.org/10.1016/j.soilbio.2021.108251, 2021.
Baiamonte, G., Pasquale, C. D., Marsala, V., Cimò, G., Alonzo, G., and Crescimanno, G.: Structure alteration of a sandy-clay soil by biochar amendments, J. Soil. Sed., 15, 816–824, https://doi.org/10.1007/s11368-014-0960-y, 2015.
Bonfante, A., Terribile, F., and Bouma, J.: Refining physical aspects of soil quality and soil health when exploring the effects of soil degradation and climate change on biomass production: an Italian case study, SOIL, 5, 1–14, https://doi.org/10.5194/soil-5-1-2019, 2019.
Bottinelli, N., Angers, D. A., Hallaire, V., Michot, D., Guillou, C. L., and Cluzeau, D.: Tillage and fertilization practices affect soil aggregate stability in a humic cambisol of northwest France, Soil Till. Res., 170, 14–17, https://doi.org/10.1016/j.still.2017.02.008, 2017.
Chaganti, V. N. and Crohn, D. M.: Evaluating the relative contribution of physicochemical and biological factors in ameliorating a saline-sodic soil amended with composts and biochar and leached with reclaimed water, Geoderma, 259, 45–55, https://doi.org/10.1016/j.geoderma.2015.05.005, 2015.
Chen, J., Liu, X., Zheng, J., Zhang, B., Yu, X.: Biochar soil amendment increased bacterial but decreased fungal gene abundance with shifts in community structure in a slightly acid rice paddy from southwest China, Appl. Soil Ecol., 71, 33–44, https://doi.org/10.1016/j.apsoil.2013.05.003, 2013.
Chen, J., Chen, D., Xu, Q., Fuhrmann, J. J., and Sun, X.: Organic carbon quality, the composition of main microbial groups, enzyme activities, and temperature sensitivity of soil respiration of an acid paddy soil treated with biochar, Biol. Fert. Soil., 55, https://doi.org/10.1007/s00374-018-1333-2, 2018a.
Chen, J., Sun, X., Zheng, J., Zhang, X., Liu, X., and Bian, R.: Biochar amendment changes the temperature sensitivity of soil respiration and composition of microbial communities 3 years after incorporation in an organic carbon-poor dry cropland soil, Biol. Fert. Soil., 54, 175–188, https://doi.org/10.1007/s00374-017-1253-6, 2018b.
Chen, L., Li, X., Peng, Y., Xiang, P., Zhou, Y., and Yao, B.: Co-application of biochar and organic fertilizer promotes the yield and quality of red pitaya (Hylocereus polyrhizus) by improving soil properties, Chemosphere, 294, 133619, https://doi.org/10.1016/j.chemosphere.2022.133619, 2022.
Chen, Y., Xu, X., Jiao X., Sui, Y., Liu, X., Zhang, J., Zhou, K., and Zhang, J.: Responses of labile organic nitrogen fractions and enzyme activities in eroded mollisols after 8-year manure amendment, Sci. Rep., 8, 14179, https://doi.org/10.1038/s41598-018-32649-y, 2018.
Dong, D., Wang, C., Zwieten, L. V., Wang, H., and Wu, W.: An effective biochar-based slow-release fertilizer for reducing nitrogen loss in paddy fields, J. Soil. Sed., 20, 3027–3040, https://doi.org/10.1007/s11368-019-02401-8, 2020.
Dong, X., Guan, T., Li, G., Lin Q., and Zhao, X.: Long-term effects of biochar amount on the content and composition of organic matter in soil aggregates under field conditions, J. Soil. Sed., 16, 1481–1497, https://doi.org/10.1007/s11368-015-1338-5, 2016.
Du, Z. L., Zhao, J. K., and Wang, Y. D.: Biochar addition drives soil aggregation and carbon sequestration in aggregate fractions from an intensive agricultural system, J. Soil. Sed., 17, 1–9, https://doi.org/10.1007/s11368-015-1349-2, 2017.
Eswaran, H., Reich, P., and Padmanabhan, E.: World soil resources: opportunities and challenges. CRC Press, Taylor and Francis Group, 29–52, 2011.
Fungo, B., Lehmann, J., Kalbitz, K., Thion, O. M., Okeyo, I., and Tenywa, M.: Aggregate size distribution in a biochar-amended tropical ultisol under conventional hand-hoe tillage, Soil Till. Res., 165, 190–197, https://doi.org/10.1016/j.still.2016.08.012, 2017.
Gaia, P., Elisa P., Maria, C., and Laura E.: Long-term conservation tillage and nitrogen fertilization effects on soil aggregate distribution, nutrient stocks and enzymatic activities in bulk soil and occluded microaggregates - sciencedirect, Soil Till. Res., 196, 104482, https://doi.org/10.1016/j.still.2019.104482, 2020.
Gao, Y., Xue, S. A., Kl, B., Tl, A., Wz, A., and Yong, W. C.: Mixture of controlled-release and conventional urea fertilizer application changed soil aggregate stability, soil humic acid molecular composition, and nitrogen uptake, Sci. Total Environ., 789, 147778, https://doi.org/10.1016/j.scitotenv.2021.147778, 2021.
Grunwald, D., Kaiser, M., and Ludwig, B.: Effect of biochar and organic fertilizers on C mineralization and macro-aggregate dynamics under different incubation temperatures, Soil Till. Res., 3612, https://doi.org/10.1016/j.still.2016.01.00, 2016.
He, M., Xiong, X., and Wang, L.: A critical review on performance indicators for evaluating soil biota and soil health of biochar-amended soils, J. Hazard. Mat., 414, 125378, https://doi.org/10.1016/j.jhazmat.2021.125378, 2021.
Hu, L., Li, S., Li, K., Huang, H., Wan, W., and Huang, Q.: Effects of Two Types of Straw Biochar on the Mineralization of Soil Organic Carbon in Farmland, Sustainability, 12, 10586, https://doi.org/10.3390/su122410586, 2020.
Islam, M. U., Jiang, F., Guo, Z., and Peng, X.: Does biochar application improve soil aggregation? A meta-analysis, Soil Till. Res., 209, 104926, https://doi.org/10.1016/j.still.2020.104926, 2021.
Jia, X., Zhong, Y., Liu, J., Zhu, G., Shang, G. Z., and Yan, W.: Effects of nitrogen enrichment on soil microbial characteristics: from biomass to enzyme activities, Geoderma, 366, 114256, https://doi.org/10.1016/j.geoderma.2020.114256, 2020.
Jiang, X. Y., Karolien, Denef., Catherine, E., Stewart, M., and Francesca, C.: Controls and dynamics of biochar decomposition and soil microbial abundance, composition, and carbon use efficiency during long-term biochar-amended soil incubations, Biol. Fertil. Soil., 52, 1–14, https://doi.org/10.1007/s00374-015-1047-7, 2016.
Joseph, S. D., Camps-Arbestain, M., Lin, Y., Munroe, P., and Chiaa, C. H.: An investigation into the reactions of biochar in soil, Soil Res., 48, 501–515, https://doi.org/10.1071/SR10009, 2010.
Khadem, A., Raiesi, F., Besharati, H., and Khalaj, M. A.: The effects of biochar on soil nutrients status, microbial activity, and carbon sequestration potential in two calcareous soils, Biochar, 3, 105–116, https://doi.org/10.1007/s42773-020-00076-w, 2021.
Kimetu, J. M. and Lehmann, J.: Stability and stabilization of biochar and green manure in soil with different organic carbon contents, Soil Res., 48, 577–585, https://doi.org/10.1071/SR10036, 2010.
Kung, C. C., Kong, F., and Choi, Y.: Pyrolysis and biochar potential using crop residues and agricultural wastes in China, Ecol. Ind., 51, 139–145, https://doi.org/10.1016/j.ecolind.2014.06.043, 2015.
Leonard, G.: Effect of biochar application rate on soil physical and hydraulic properties of a sandy loam, Arch. Agron. Soil Sci., 60, 457–470, https://10.1080/03650340.2013.821698, 2014.
Li, C. Z., Zhang, H., Yao, W. J., Xu, C., Wu, D., Wang, J. D., Ai, Y. C., and Zhang, Y. C.: Effects of biochar application combined with nitrogen fertilizer on soil physicochemical properties and winter wheat yield in the typical ancient region of the yellow river, China, Chin. J. Appl. Ecol., 31, 3424–3432, https://doi.org/10.13287/j.1001-9332.202010.028, 2020.
Li, F., Qiu, P., Shen, B., and Shen, Q.: Soil aggregate size modifies the impacts of fertilization on microbial communities, Geoderma, 343, 205–214, https://doi.org/10.1016/j.geoderma.2019.02.039, 2019.
Li, L. C., Yang, M. Y., Li, J. C., Bol, R., Du, Z. L., and Wu, D.: Potential denitrification activity response to long-term nitrogen fertilization – A global meta-analysis, J. Clean. Prod., 336, 130451, https://doi.org/10.1016/j.jclepro.2022.130451, 2022.
Li, L.J., Burger, M., Du, S. L., Zou, W. X., You, M. Y., Hao, X. X., Lu, X. C., Zheng, L., and Han, X. Z.: Change in soil organic carbon between 1981 and 2011 in croplands of Heilongjiang Province, northeast China, J. Sci. Food Agr., 96, 1275–1283, 2016.
Lin, H., Zhou, M., Zhang, B., Li, Z., and Zhu, B.: Effects of long-term application of biochar and straw on soil aggregate organic carbon in purple slope farmland, Chin. J. Eco-Agr., 28, https://doi.org/10.13930/j.cnki.cjea.190614, 2020.
Luo, C., Yang, J., Chen, W., and Han, F.: Effect of biochar on soil properties on the Loess Plateau: Results from field experiments, Geoderma, 369, 114323, https://doi.org/10.1016/j.geoderma.2020.114323, 2020.
Luo, S., Wang, S., Tian, L., Li, S., Li, X., Shen, Y., and Tian, C.: Long-term biochar application influences soil microbial community and its potential roles in semiarid farmland, Appl. Soil Ecol., 117, 10–15, https://doi.org/10.1016/j.apsoil.2017.04.024, 2017.
Madari, B. E., Silva, M., Carvalho, M., Maia, A., Petter, F. A., Santos, J., Tsaid, S., Leala, W., and Zeviani W.: Properties of a sandy clay loam haplic ferralsol and soybean grain yield in a five-year field trial as affected by biochar amendment, Geoderma, 305, 100–112, https://doi.org/10.1016/j.geoderma.2017.05.029, 2017.
Mei, N., Zhang, X., Wang, X., Peng, C., and Gu, Y.: Effects of 40 years of applications of inorganic and organic fertilization on soil bacterial community in a maize agroecosystem in northeast china, Europ. J. Agron., 130, 126332, https://doi.org/10.1016/j.eja.2021.126332, 2021.
Mete, F. Z., Mia, S., Dijkstra, F. A., Abuyusuf, M., Hossain, A., and Agronomy, D. O.: Synergistic Effects of Biochar and NPK Fertilizer on Soybean Yield in an Alkaline Soil, Pedosphere, 25, 713–719, https://doi.org/10.1016/S1002-0160(15)30052-7, 2015.
Miller, R. M. and Jastrow, J. D.: Hierarchy of root and mycorrhizal interactions with soil aggregation soil biol biochem, Soil Biol. Biochem., 22, 579–584, https://doi.org/10.1016/0038-0717(90)90001-G, 1990.
Ng, E. L., Patti, A. F., Rose, M. T., Schefe, C. R., Wilkinson, K., Smernik, R. J., and Cavagnaro, T.: Does the chemical nature of soil carbon drive the structure and functioning of soil microbial communities?, Soil Biol. Biochem., 70 54–61, https://doi.org/10.1016/j.soilbio.2013.12.004, 2014.
Oksana, C., De Deyn, G. B., and van der Ploeg, M.: Soil microbiota as game-changers in the restoration of degraded lands, Rest. Ecol., 375, 6584, https://doi.org/10.1126/science.abe0725, 2022.
Palansooriya, K. N., Wong, J., Hashimoto, Y., Huang, L., and Rinklebe, J., and Chang, S. X.: Response of microbial communities to biochar-amended soils: a critical review, Biochar., 1, 3–22, https://doi.org/10.1007/s42773-019-00009-2, 2019.
Peng, X., Horn, R., and Hallett, P.: Soil structure and its functions in ecosystems: phase matter & scale matter, Soil Till. Res., 146, 1–3, https://doi.org/10.1016/j.still.2014.10.017, 2015.
Plaza, C., Giannetta, B., Fernández, J., M., López-de-Sá, Esther G., Polo, A., Gascó, G., Méndez, and Zaccone, C.: Response of different soil organic matter pools to biochar and organic fertilizers, Agr. Ecosyst. Environ., 225, 150–159, https://doi.org/10.1016/j.agee.2016.04.014, 2016.
Scow, K. M. and Parikh, S. J.: Biochar additions can enhance soil structure and the physical stabilization of C in aggregates, Geoderma, 303, 110–117, https://doi.org/10.1016/j.geoderma.2017.05.027, 2017.
Sekaran, U., Sandhu, S. S., Qiu, Y., Kumar, S., and Hernandez, J.: Biochar and manure addition influenced soil microbial community structure and enzymatic activities at eroded and depositional landscape positions, Land Degrad. Dev., 31, 894–908, https://doi.org/10.1002/ldr.3508, 2019.
Shi, D., Wang, X., Duan, J., Liu,A., Luo, A., Li, R., and Hou, Z.: Effects of nitrogen reduction combined with biochar application on soil organic carbon active components and mineralization in yellow soil paddy field, Chin. J. Appl. Ecol., 31, 4117–4124, https://doi.org/10.13287/j.1001-9332.202012.027, 2020.
Sokol, N. W. and Bradford, M. A.: Microbial formation of stable soil carbon is more efficient from belowground than aboveground input, Nat. Geosci., 12, 46–53, https://doi.org/10.1038/s41561-018-0258-6, 2019.
Song, D., Chen, L., Zhang, S., Zheng, Q., and Wang, X.: Combined biochar and nitrogen fertilizer change soil enzyme and microbial activities in a 2-year field trial, Europ. J. Soil Biol., 99, 103212, https://doi.org/10.1016/j.ejsobi.2020.103212, 2020.
Thiet, R. K., Frey, S. D., and Six, J.: Do growth yield efficiencies differ between soil microbial communities differing in fungal bacterial ratios? Reality check and methodological issues, Soil Biol. Biochem., 38, 837–844, https://doi.org/10.1016/j.soilbio.2005.07.010, 2006.
Tian, J., Wang, J., Dippold, M., Gao, Y., Blagodatskaya, E., and Kuzyakov, Y.: Biochar affects soil organic matter cycling and microbial functions but does not alter microbial community structure in paddy soil, Sci. Total Environ., 556, 89–97, https://doi.org/10.1016/j.scitotenv.2016.03.010, 2016.
Tisdall, J. M. and Oades, J. M.: Organic matter and water-stable aggregates in soils, J. Soil Sci., 33, 141–163, https://doi.org/10.1111/j.1365-2389.1982.tb01755.x, 1982.
Trivedi, P., Delgado-Baquerizo, M., Jeffries, T. C., Trivedi, C., Anderson, I. C., Mcnee, M., Kenneth, F., Bhupinder, P., David, M., and Brajesh K. S.: Soil aggregation and associated microbial communities modify the impact of agricultural management on carbon content, Environ. Microbiol., 19, 3070–3086, https://doi.org/10.1111/1462-2920.13779, 2017.
Wang, C., Chen, D., Shen, J., Yuan, Q., and Wu, J.: Biochar alters soil microbial communities and potential functions 3–4 years after amendment in a double rice cropping system, Agr. Ecosyst. Environ., 311, 107291, https://doi.org/10.1016/j.agee.2020.107291, 2021.
Wang, C., Jiang, K., Lu, Y. Ou, J., and Huang, W.: Effects of different organic materials application on aggregate composition and stability of latosol, Soil Sci., 50, 1328–1334, https://doi.org/10.19336/j.cnki.trtb.2019.06.10, 2019.
Wang, H. Y., Nie, Y., Clayton, R., Butterly, L., and Wang, Q.: Fertilization alters microbial community composition and functional patterns by changing the chemical nature of soil organic carbon: A field study in a Halosol, Geoderma, 292, 17–24, https://doi.org/10.1016/j.geoderma.2017.01.006, 2017.
Wang, R. F., Zhang, J. W., Dong, S. T., and Liu, P.: The present situation of maize straw resource utilization and its effect in main maize production regions of china, Chin. J. Appl. Ecol., 22, 1504–1510, 2011.
Weyers, S. L. and Spokas, K. A.: Impact of biochar on earthworm populations: a review, Appl. Environ. Soil Sci., 2011, 1–12, https://doi.org/10.1155/2011/541592, 2011.
Xiu, L., Zhang, W., Sun, Y., Wu, D., Meng, J., and Chen, W.: Effects of biochar and straw returning on the key cultivation limitations of Albic soil and soybean growth over 2 years, Catena, 173, 481–493, https://doi.org/10.1016/j.catena.2018.10.041, 2019.
Xiu, L., Zhang, W.,Wu, D., Sun, Y., Zhang, H.,Gu, W., Wang, Y., Meng, J., and Chen, W.: Biochar can improve biological nitrogen fixation by altering the root growth strategy of soybean in Albic soil, Sci. Total Environ, 773, 144564, https://doi.org/10.1016/j.scitotenv.2020.144564, 2021.
Xu, G., Song, J., Zhang, Y., and Lv, Y.: Effects of Biochar Application on Soil Organic Carbon Mineralization during Drying and Rewetting Cycles, Bioresources, 14, 9957–9967, 2019.
Xue, B., Huang, L., Huang, Y., Kubar, K., Li, X., and Lu, J.: Straw management influences the stabilization of organic carbon by Fe (oxyhydr) oxides in soil aggregates, Geoderma, 358, 113987, https://doi.org/10.1016/j.geoderma.2019.113987, 2019.
Yin, D., Meng, J., Shi, G., Cao, T., Chen, Z., Zhou, J., Zheng, G., and Chen, W: Biochar as a tool to improve physicochemical properties of Chinese albic soils, J. Biob. Mat. Bioenerg., 12, 102–108, https://doi.org/10.1166/jbmb.2018.1735, 2018.
Ying, D.: Effects of heavy metal pollution on organic carbon mineralization and microbial community structure in paddy soil under corn stalk addition, Nanjing Agricultural University, 7, 1–77, https://doi.org/10.27244/d.cnki.gnjnu.2018.000742, 2018.
Yuan, J. J., Tong, Y. A., Lu, S. H., and Yuan, G. J.: Effects of combined application of biochar and nitrogen fertilizer on soil fertility, yield, and quality of Chinese jujube, Plant Nutr. Fert. Sci., 23, 468–475, https://doi.org/10.11674/zwyf.16285, 2017.
Yuan, Y., Zhang, B., Han, X., Ding, X., and Liang, C.: Higher rates of manure application lead to greater accumulation of both fungal and bacterial residues in macroaggregates of clay soil, Soil Biol. Biochem., https://doi.org/10.1016/j.soilbio.2015.02.015, 2015.
Zhang, H. J., Ding, W., X., Yu, H., Y., and He, X. H.: Carbon uptake by a microbial community during 30-day treatment with 13C-glucose of a sandy loam soil fertilized for 20 years with NPK or compost as determined by a GC–C–IRMS analysis of phospholipid fatty acids, Soil Biol. Biochem., 57, 228e236, https://doi.org/10.1016/j.soilbio.2012.08.024, 2013.
Zhang, Q., Du, Z. L., Lou, Y., and He, X.: A one-year short-term biochar application improved carbon accumulation in large macroaggregate fractions, Catena, 127, 26–31, https://doi.org/10.1016/j.catena.2014.12.009, 2015.
Zhang, S., Cui, J., Wu, H., Zheng, Q., and Zhang, S.: Organic carbon, total nitrogen, and microbial community distributions within aggregates of calcareous soil treated with biochar, Agr. Ecosyst. Environ., 314, 107408, https://doi.org/10.1016/j.agee.2021.107408, 2021.
Zhang, Y., Li, X., Gregorich, E. G., Mclaughlin, N. B., and Liang, A.: Evaluating storage and pool size of soil organic carbon in degraded soils: tillage effects when crop residue is returned, Soil Till. Res., 192, 215–221, https://doi.org/10.1016/j.still.2019.05.013, 2019.
Zhang, Y., Li, X., Gregorich, E., McLaughlin, N.; Zhang, X., Guo, Y., Liang, A., Fan, R., and Sun, B.: No-tillage with continuous maize cropping enhances soil aggregation and organic carbon storage in northeast china, Geoderma, 330, 204–211, https://doi.org/10.1016/j.geoderma.2018.05.037, 2018.
Zhang, Z., Yan, J., Han, X., Zou, W., Chen, X., Lu, X., and Feng, Y.: Labile organic carbon fractions drive soil microbial communities after long-term fertilization, Glob. Ecol. Conserv., 32, e01867, https://doi.org/10.1016/j.gecco.2021.e01867, 2021.
Zheng, W., Zhao, Z., Lv, F., Yin, Y., Zhai, B.: Fungal alpha diversity drives the stochasticity of bacterial and fungal community assembly in soil aggregates in the apple orchard, Soil sampling and aggregate fractionation, https://doi.org/10.21203/rs.3.rs-56672/v1, 2020.Ÿ
Zhou, H., Fang, H., Zhang, Q., Wang, Q., Chen, C., Mooney, S. J., Peng, X. H., and Du, Z. L.: Biochar enhances soil hydraulic function but not soil aggregation in a sandy loam, Eur. J. Soil Sci., 70, 291–300, https://doi.org/10.1111/ejss.12732, 2019.