the Creative Commons Attribution 4.0 License.
the Creative Commons Attribution 4.0 License.
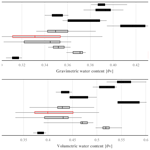
Reusing Fe water treatment residual as a soil amendment to improve physical function and flood resilience
Heather C. Kerr
Karen L. Johnson
David G. Toll
Soil degradation is a global challenge that is intrinsically linked to climate change and food security. Soil degradation has many causes, but all degraded soils suffer from poor soil structure. The increasing global production of water treatment residual (WTR), an organo-mineral waste product from clean water treatment, means that the sustainable reuse of this waste provides a potential timely opportunity, as research has shown that WTR application to soil can improve soil health. Recycling or reuse of WTR to land is commonplace across the world but is subject to limitations based on the chemical properties of the material. Very little work has focused on the physical impacts of WTR application and its potential to rebuild soil structure, particularly improving its ability to hold water and resist the effects of flooding. This paper presents novel research in which the use of Fe WTR and Fe WTR compost [1 : 1] co-amendment has shown to be beneficial for a soil's water retention, permeability, volume change, and strength properties, all critical in soil health. Application rates of WTR were 10 %–30 % by dry mass. Compared with the control sandy loam soil, co-amended samples have 5.7 times the hydraulic conductivity (570 % improvement), 54 % higher shear strength, and 25 % greater saturated water content. Single WTR-amended soil had 26 times the saturated hydraulic conductivity (2600 % improvement), 129 % higher shear strength, and 13.7 % greater saturated water content. Data indicate that Fe WTR can be added as a single amendment to significantly improve soil physical characteristics where shear strength and hydraulic conductivity are the most important factors in application. Although the co-application of Fe WTR with compost provides a lesser improvement in shear strength and hydraulic conductivity compared with single WTR amendment, the co-amendment has the best water retention properties and provides supplementary organic content, which is beneficial for environmental applications where the soil health (i.e. ability to sustain ecosystem functions and support plants) is critical.
- Article
(936 KB) - Full-text XML
- BibTeX
- EndNote
Soil is a living system (Lal, 2016) and requires influxes of both organic and inorganic materials to maintain and improve soil health, which can be defined as the ability of soils to deliver critical ecosystem services such as plant growth, water storage, and carbon storage. A soil's water-holding capacity, permeability, volume change, and shear strength are fundamentally important for the delivery of these ecosystem services, as they control the flux of air and water and turnover of soil organic carbon that feeds the soil microbiome. The enhancement of soil water retention has long been addressed using organic amendments such as manure, biochar, or compost, owing to their beneficial impact on soil structure (Rahman et al., 2017; Zhou et al., 2019, 2020), and correspondingly nations are aiming to increase soil organic carbon by 0.4 % every year as agreed in the COP21 agreement (Rhodes, 2016). Much research has considered the best organic materials to add to soil, and it is generally agreed that “active carbon”, that can feed the soil microbiome allowing it to grow, is important to build soil organic carbon. The water-holding capacity and structural stability of a soil are not just dependent on organic matter, but also on particle size distribution and soil structure, which is largely governed by the inorganic mineral component of the soil. In addition, there is growing evidence that it is inorganic minerals which present the best opportunity to stabilize soil structure (Peng et al., 2007), stabilize carbon, and help us achieve net zero (Tipping and Rowe, 2019).
It is with these rationales that the addition of an organic and inorganic rich waste material to soil provides significant potential to improve soil function. The drive to reuse waste materials has been vastly accelerated, owing to building pressure and initiatives to reuse and recycle waste as well as initiatives to improve soils (Hazbavi and Sadeghi, 2016; Krause et al., 2016). This provides a timely opportunity to promote the use of waste sludges from the clean water treatment industry for soil amendment. The worldwide supply of clean drinking water is an ever-growing demand, with up to 30 % of the world still yet to have access to potable, treated water (Cotruvo, 2017; Turner et al., 2019) and global demand increasing 1 % yr−1 (UNESCO, 2019). Treatment of source water is needed to remove harmful contaminants, pathogens, organisms, and suspended solids and usually involves the addition of iron (Fe) or aluminium (Al) salts to coagulate and flocculate these undesired particles for removal (Keeley et al., 2014). The waste product of this initial process in the treatment of drinking water is commonly referred to as water treatment residual, sludge, or cake (henceforth referred to as WTR). Although properties of the sludge are determined by many local factors, WTR typically contains predominately Al or Fe salts/oxides (60 %) and organic matter (40 %) and is currently classified as a non-hazardous waste, “sludges from water clarification” (European Waste Code 190902, within 11. Common Sludges). From the point of view of rebuilding degraded soils, this waste provides a prime source of inorganic and organic matter since WTR contains both.
The potential chemical/biological changes of the soil (due to WTR addition) that affect functions such as plant growth are covered elsewhere (e.g. Zhao et al., 2018), so this paper focusses on the physical properties. Despite a wealth of studies investigating plant growth and associated chemical/biological effects of WTR amendment, there are very few explicit data on the physical advantages and disadvantages of amendment using Al WTR and none to our knowledge on Fe WTRs, thus meriting its investigation. The potential for WTR's use in environmental remediation or as a soil conditioner to improve soil function has been highlighted because of the high content of organic matter (8 %–40 %) in addition to Al or Fe salts used (Makris et al., 2005). Al/Fe salts and organic matter are able to beneficially modify soil characteristics due to their effect on aggregation and cementation between soil particles, effect on bulk density (due to low density of organic matter), and water retention characteristics. In particular, Fe oxides are known to be good at stabilizing carbon in marine systems (Lalonde et al., 2012), and thus their potential role in carbon stabilization and soil structure is also very interesting (Tipping and Rowe, 2019). As such, we present an investigation on the physical properties (water retention properties, hydraulic conductivity, and shear strength) of a sandy loam soil amended with both Fe WTR and a Fe WTR compost mix. These physical characteristics can be used as a proxy for soil health because of their impact on soil structure and soil function (Neal et al., 2020) and must be assessed to assess the influence of a particular amendment. As discussed in detail by Kerr et al. (2016), by observing these physical characteristics together, one can fully assess the changes to a soil's health due to amendment and understand its capacity to withstand events such as flooding. Singular physical measures commonly used to indicate soil function, such as saturated water content (mass of water mass of solids when all voids filled are with water), are not sufficient to provide real indication of how a soil would respond to a flood event or prolonged periods of wet weather, as structural properties such as shear strength will also have significant influence on the resilience of a soil. Owing to the nature of the amendments used in this research, using gravimetric water content (GWC) alone is a problematic reference parameter as inorganic and organic materials added to soil have high initial water contents (like WTR and compost) and low specific gravities; thus amended soil will naturally have an initially higher maximum gravimetric content (as shown by Moodley et al., 2004). Similarly, volumetric water content (VWC) is calculated using the ratio of volume of water to wet soil volume or by using the gravimetric water content and dry density. As individual measurements, neither convey any information on the increase in volume of the specimen and therefore potential capacity to hold more water, particularly under flooded conditions (Boivin et al., 2009; Sollins and Greg, 2017). The term “flood-holding capacity” is used here to characterize the performance of amended soils, based on the water retention properties (gravimetric and volumetric water content and hydraulic conductivity) in addition to the volume change characteristics of soil under climatic cycles and shear strength properties (Kerr et al., 2016). As such, an amendment that provides soil with the best flood-holding capacity will be one that has a high capacity to hold and transmit water, together with a stable soil structure indicated by volume change and good shear strength.
1.1 Water treatment residual characteristics
Many recent review publications such as Ippolito et al. (2011), Dassanayake et al. (2015), Mokonyama et al. (2017), Odimegwu et al. (2018), and Zhao et al. (2018) have discussed the wide range of global WTR properties, current disposal, and reuse/recycling options for WTR and thus are not detailed here. Turner et al. (2019) provide the most recent and extensive review of potential reuse of WTR; however owing to its prevalence, much of the focus is still on Al WTR. WTR is generally considered to be an organo-mineral, and the water content and mechanical and chemical properties of the sludge are entirely dependent on the procedures employed at each water treatment works (WTWs) and the nature of the local water source. Water treatment works use Fe salts, Al salts, polyelectrolyte (polyDADMAC), or in some cases a combination of all three in coagulation and flocculate stages of treatment, the choice and dosage of which are dependent on the source water. Unprocessed WTR contains a fine solid fraction of between 2 % and 4 % (Dassanayake et al., 2015). This solid fraction comprises predominantly metal salt coagulant, organic constituents, and other particulates from the catchment. Owing to the high volume of waste produced, water treatment works use various methods to dewater the sludge retrieved from settling beds to between 17 % and 35 % solids in order to make its transport and disposal more economically viable, whereby the extent of dewatering is governed by the cost benefit (Li et al., 2016).
Finlay (2015) provides a summary of characteristics of nine WTRs from the NE UK (Table 1), which suggests that the nutritional elements of WTR are similar to soil but generally lower than other typical amendments such as biosolids and composts. Importantly, the levels of N and P vary throughout the seasons, which may be important when considering rate of land application. UK figures are representative of WTR chemical attribute ranges from 62 other regions across the globe (Shen et al., 2019), such as the USA (Elliot and Dempsey, 1991; Makris et al., 2005; Agyin-Birikorang et al., 2007; Ippolito et al., 2009; Nagar et al., 2009), Ireland (Babatunde et al., 2009), Czech Republic (Kyncl, 2008), Egypt (Mahdy et al., 2009), Australia (Oliver et al., 2011), South Africa (Titshall and Hughes, 2005), Belgium (Chiang et al., 2012), and Japan (Keeley et al., 2014).
1.2 Use of WTR to improve physical soil characteristics
There are a considerable number of potential alternative applications for the reuse or recycling of WTR that have been extensively covered in other publications and thus are not discussed here (Ippolito et al., 2011; Zhao et al., 2018; Turner et al., 2019). It is common practice for water companies to spread WTR to land as a disposal method, with examples including Northumbrian Water (England) and Sydney Water (NSW, Australia), which dispose 100 % of their WTR via land spreading (NWL, Ed Higgins, personal communication, 2019; Turner et al., 2019). The existing research on land application of WTRs has focussed on the chemical implications of its application, with most investigating the effect of Al WTR amendments on crop growth owing to the potential toxicity of Al, the presence of other potentially toxic elements (PTEs), and phosphorus immobilization (Moodley et al., 2004). To date there are far fewer reports on the use of Fe WTR in plant trials as Al is prevalent, and its chemical attributes are of greater concern. Notwithstanding, Zhao et al. (2018) provide an extensive review of plant trials using both Fe and Al WTR as a soil amendment and found no standard plant response to WTR addition. For example, Oladeji et al. (2007, 2008) reported alternate plant responses despite otherwise identical parameters at an application rate of 10–25 g kg−1 by oven-dried mass. Similarly Ippolito et al. (2009) investigated the long-term effect (15 years) of Al WTR and biosolid co-amendment at up to 21 Mg ha−1 (no incorporation into the soil profile) and found minimal disruption of soil chemistry, microbial diversity, or plant nutrient levels. Ippolito et al. (2009) suggest that the single application of Al WTR would pose little threat to plant or soil biology and provide long-term solutions for soils with excess. Mukherjee et al. (2014) provide another example of “long-term” application of Al WTR to amend soil (0.5 % wt of WTR per wt of soil) and found little change in soil physiochemical proprieties and greenhouse gas emissions compared with the control soil over 2 years, although they did report an increase in penetration resistance of 87 %.
There is a scarcity in research on physical changes in soil resulting from WTR addition, with physical characteristics only noted as secondary findings to the chemical/biological research. To date only a few studies, highlighted below, have explicitly explored the effect of Al WTR specifically on water retention (relationship between water content and suction), permeability (hydraulic conductivity), or shear strength, and none to the authors' knowledge have investigated Fe WTR. The specific material shear strength characteristics of WTR have been investigated extensively for their beneficial use in construction materials (Hegazy et al., 2012; Rodrigues and Holanda, 2015; Gomes et al., 2019; Liu et al., 2020; Huang and Wang, 2013); however Rengasamy et al. (1980) is the only published study investigating the effect of WTR amendment on shear strength, despite this being a critical parameter for the resilience and stability of soils under saturation. El-Swaify and Emerson (1975) found that Al and Fe hydroxides in WTR act as cementing agents between soil particles, which imparts fundamental changes to important soil physical parameters, by reducing swelling and increasing aggregate stability (Elliot and Dempsey, 1991). Rengasamy et al. (1980) reported a 18 %–85 % increase in water-holding capacity with extremely low rates of Al WTR application [20 Mg ha−1 = 0.002 % wt/wt] whilst also reporting increased aggregation. Elliot and Demsey (1991) identified potential for sludges to significantly alter soil aggregation and permeability and modify water retention properties based on the high proportion of organic matter in the material. More recently, studies have also noted these improvements using Al WTRs, but these physical parameters have not been the focus of the study (Basta, 2000; Dayton and Basta, 2001). Moodley et al. (2004) specifically investigated the water retention and hydraulic conductivity of soil amended with up to 10.6 % wt/wt amendment [1280 Mg ha−1] air-dried WTR and found increased saturated water content at the highest application rate, and a shift in the water retention curves was observed due to addition of fine particles, but the readily available water (−10 to −100 kPa) was unchanged. Three years after the start of the trial, all soil water retention curves (SWRCs) were almost identical to the control, which may indicate that these changes are of short-term benefit. Moodley et al. (2004) also observed a 9–10-fold increase in hydraulic conductivity with WTR addition due to the creation of preferential pathways (large pores and surface channels). WTR's improvement of hydrological conductivity was attributed to high stability and limited swell of WTR aggregates, which reduces pore blockages shown in control soils. The addition of highly porous WTR reduced the bulk density of the soil, an effect strongly correlated with the proportion of amendment. Moodley et al. (2004) summarize that despite increased water retention and conductivity at the highest rate of application, there is no additional benefit for crops as plant available water remains unchanged.
Most recently, Kerr et al. (2016) used air-dried Fe WTR alone and with a compost co-amendment to change the physical properties of a sandy loam soil. WTR was applied at a rate of between 30 % and 50 % (wt/wt dry mass), which are in the extreme upper limits of realistic field application. The most important finding of this study was that at saturation, the 30 % co-amendment held almost as much water [0.4 g g−1] as the single compost amendment at the same rate of application [0.43 g/g]. Compared with the saturated water content of the control soil [0.32 g/g], this is an important improvement. This provides significant rationale for exploring co-amendment of WTR and compost as an alternative to singular organic amendments typically used to improve water-holding capacity and associated benefits in soil structure. Soils amended with just WTR did not see improvement in saturated water content of the soil compared with the control, although it did improve the rate of water ingress by up to 42 % in the first 24 h compared with the control soil. Fall cone penetrometer (BS 1377–2:1990) testing indicated that the addition of WTR had considerable potential to increase the undrained shear strength of specimens, but owing to large error in results, the effect was inconclusive.
To investigate the physical effects of Fe WTR amendment on a sandy loam, remoulded amended soil specimens were tested for water retention (gravimetric and volumetric water content at saturation), saturated hydraulic conductivity, shear strength, and soil shrinkage behaviour (volume change). Four amendment types were tested: (1) control soil, (2) Fe WTR (as raw and air-dried), (3) compost, and (4) co-amendment using WTR and compost. Each amendment type was tested at application rates of 10 % and 30 % wt/wt by dry mass.
2.1 Materials
Well-characterized topsoil was retrieved from a local farm (Nafferton Farm, NZ065656), and Fe WTR obtained from Mosswood water treatment works (Northumbrian Water Ltd) was added as an amendment to soil as the raw, dewatered material retrieved from the water treatment plant, subsequently named WTRw (∼ 20 % total dissolved solids), or an air-dried version of the same material (0.2 g/g gravimetric water content), named henceforth as WTRd. WTRs exhibit a wide range of physiochemical properties determined by local factors, and therefore extensive characterization of the Mosswood WTR (Kerr, 2019) was carried out prior to soil testing. Commercially available compost was used as received from the supplier (PAS100 compost, 100 % recycled green compost product derived from surplus garden trimmings and local authority recycling contracts). Remoulded soil specimens were compacted dynamically to a density of 1.75 g cm−3 at a water content of 0.175 g/g. As per Kerr et al. (2016), soils containing single amendments of compost and Fe WTR were tested in addition to the co-amendment, to investigate the individual effect of the amendment on the control soil. The application rate of WTR was chosen based on the maximum spreading thresholds of current UK legislation of 3000 t ha−1 yr−1, which equates to ∼ 50 % amendment (% wt/wt by dry mass) with the assumptions made in Sect. 1.4 that incorporation depth is 0.2 m and dry density of the soil is 1.2 g cm−3. Realistic typical deployment of 100 t ha−1 equates to just 0.04 % amendment % wt/wt by dry mass (NWL, Ed Higgins, personal communication, 2019).
2.2 Material properties
Table 2 summarizes the material properties of soil, WTRd, WTRw, and compost, characterized using British Standards BS1377. WTRs are typically characterized as inorganic clay of low to medium plasticity (Huang and Wang, 2013). Particle size distribution testing was not achievable for WTRw due to issues of zone settling for fine particles in the hydrometer, as found in Xia (1994), because of issues with effective flocculant dispersal. Previous research has determined that for most WTRs, 95 % of particles pass 0.075 mm and thus are clay-sized. The small pycnometer method was used to characterize the particle density of WTRd and compost. Following Weindorf and Wittie (2003) and O'Kelly (2016), hexane replaced water in the determination of compost particle density to facilitate submersion of very low-density material. Both compost and WTRd were de-aired within the flask using a vacuum pump for a period of 3 d before particle density. This method was adopted following Basim (1999) in their extensive characterization of WTR and organic soils, as heating the contents for air removal would result in the loss of volatile solids and organic fractions.
2.3 Sample preparation and testing
Preliminary data (not presented) indicated that specimens produced using dynamic compactive effort had considerable differences in density, depending on their amendment proportions and water content, and as such made comparison of water retention characteristics between different amendments very difficult to assess. The dry density (mass of solids (g) divided by the volume of specimen (cm3)) and gravimetric water content (mass of water divided by the mass of dry solids) were used as controlling factors in specimen preparation to provide more comparable data between different amendments. By comparing the performance of amendments based on dry mass per unit area, the density as a controlling factor on water retention is removed, and an assessment can be made on the retention properties of the material. The drawback of this method is that compost must undergo greater compressive efforts to achieve the required dry density owing to compost's low particle density (1.675 g cm−3), thereby reducing the macro porosity through which it achieves good water-holding capabilities, although Peng et al. (2012) found that soil volume change characteristics were independent of soil compaction intensity. This is not as problematic for soil and WTR as their particle densities are more similar (2.65 and 2.11 g cm−3 respectively). The compost within specimens was able to swell and regain structure once wetting was initiated, thus reducing the initial effect of compaction during preparation.
Cylindrical specimens 38×76 mm were compacted using a static press to a dry density of 1.75 g cm−3 at a water content of 0.175 g g−1. WTRd was prepared by air-drying and sieved to 6.3 mm before addition to soil. WTRw was added to soil as a dewatered sludge (20 % solids) and mixed with soil before the amended material was dried to 0.175 g/g. As such, both WTRd and WTRw are air-dried forms of WTR, but amendment using WTRw produces an amendment mixture with greater distribution of fines owing to the preparation procedure. Once extruded, the sides of the specimens were coated with liquid latex and open ends of the specimen were wrapped in fine material to reduce the loss of fines. Amendment proportions were as follows and were mixed based on the dry mass of each component material: 100 % soil (control), 30 % single amendment of WTRw, WTRd, compost, or 30 % co-amendment (1 : 1), 10 % single amendment of WTRw, WTRd 10 % compost, or 10 % co-amendment (1 : 1). Twelve replicates were produced for water retention testing and three replicates for triaxial testing (shear strength and hydraulic conductivity). Specimens were subjected to climatic cycles as per Kerr et al. (2016). Water ingressed through the base of the sample, and once mass negligibly increased, the specimens were flooded for a period of 72 h (Fig. 1). The duration of wetting was 7–14 d depending on the amendment type. After the 72 h period of flooding, specimens were dried for 2 weeks before the second wetting was initiated. As such, the water content of specimens was variable after drying. The mass and specimen volume were measured using a standard mass balance ±0.01 g and digital callipers, through two wetting, flooding, and drying cycles. Specimens were tested in triaxial apparatus 4 weeks after preparation but had not undergone any climatic sequences before testing. The saturated hydraulic conductivity of specimens was tested during consolidated undrained triaxial testing (BS 1377), once the sample had been consolidated. Three specimens of each amendment were subsequently sheared each at the specified cell pressure (25, 50, and 100 kPa).
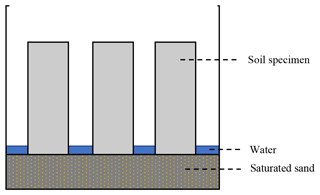
Figure 1Soil specimens with latex coating, situated on saturated silica during for the primary wetting sequence.
As soils that have been compacted or remoulded have been found to reach equilibrium after three to five wetting/drying cycles (Tripathy et al., 2002), analysis of all data was conducted on the second wetting and drying cycle to investigate the initial effects of WTR amendment. Further climatic cycles were not achieved due to deterioration of specimens. Numerical testing for statistical significance was conducted using a Mann–Whitney test assuming non-parametric data.
In general, there were no statistical differences between amendment using WTRd or WTRw despite numerical differences in the averaged values of these amendments. This may reflect heterogeneity between specimens amended with WTRd or WTRw rather than a fundamental difference in material properties as a result of the addition of WTR in a raw or air-dried format. Each physical parameter is discussed below, and conclusions on the cumulative effect on the flood-holding capacity and soil health are subsequently made in Sect. 3.5.
3.1 Water content relationships
All soils with amendments yielded statistically significant (p<0.05) higher gravimetric and volumetric water content at saturation compared with the control soil (with the exception of 10 % WTRd co-amendment). Figure 2 shows that specimens with the highest ratio of amendment had the highest water retention at saturation; the 10 % amended soils had gravimetric water contents (GWC) of between 5.4 % and 17.8 % greater than the control (corresponding volumetric water content (VWC) between 11.3 % and 34.4 %). All 30 % amended soils showed increases of between 11.2 % and 32.4 % GWC (12.8 % and 43.6 % VWC) compared with the control.
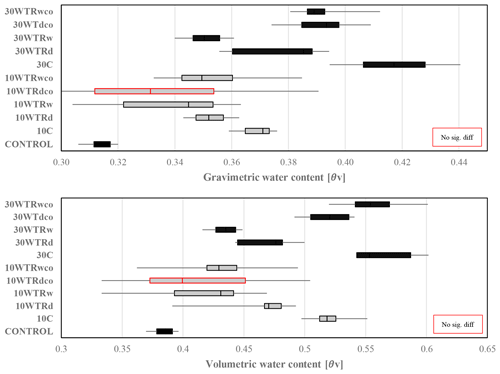
Figure 2Box plot of maximum gravimetric water content (θg) and volumetric water content (θv) of specimens at saturation after second flooding event.
The key findings for water retention are that all single amendments of WTR significantly increased the gravimetric and volumetric water content at saturation compared with the control soil. The differences in GWC and VWC were as follows: 10WTRd (increase of 11.7 % and 21.9 %), 10WTRw (increase of 9.5 % and 11.8 %), 30WTRd (increase of 22.3 % and 23.5 %), 30WTRw (increase of 11.2 % and 12.8 %). Compost-only amendments have higher GWC and VWC at saturation compared with single WTR or co-amended specimens at the same ratio, which is an expected finding owing to compost's well-known water retention properties (Kay, 1998). The use of co-amendment improves both the GWC and VWC compared with the control by 24.7GWC % and 43.6VWC % at 30 % amendment and 10.9GWC % and 11.3VWC % at 10 % amendment. These findings are important for the potential of amendment to increase flood-holding capacity, as it is clear that both co-amendment and single amendments of WTR increase the water held at saturation compared with the control soil, thus increasing the amount of water held in the soil before the initiation of surface run off. Although the addition of a single compost amendment produces the best improvement of water retention at saturation, these results show that the addition of WTR does not hinder the ability of organic amendments to retain water when combined as a co-amendment.
3.2 Soil volume change
Soils with high organic content have more pronounced hysteresis than inorganic soils, which are stiffer in comparison (Peng and Horn, 2007); however Elliot and Dempsey (1991) suggest that WTRs reduce swelling and increase aggregate stability owing to their chemical attributes. These effects are shown in Fig. 3, which shows that amendments with high proportion of organic content swell to a greater degree than the WTR-amended soils.
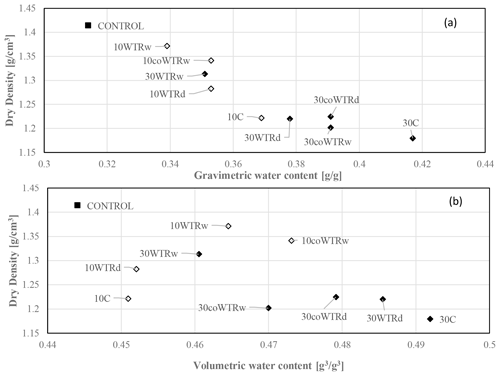
Figure 3(a) Relationship between dry density and saturated gravimetric water content and (b) relationship between dry density and saturated volumetric water content.
In Fig. 3, the relationship between dry density and water content at saturation for amended soils indicates that soils with the greatest organic content undergo the greatest swelling during wetting. Specimens were initially prepared at 1.75 g cm−3, and thus the change in dry density is an indicator of the ability of the material to swell upon wetting. Figure 3 also shows that the water retention at saturation appears to be loosely correlated to the dry density of the specimen at saturation, as a lower density indicates a greater proportion of pore space. The control soil had the highest dry density (1.41 g cm−3), lowest GWC (0.314), and lowest VWC (0.444), and in contrast the 30 % compost amendment had the lowest dry density (1.17 g cm−3) and highest water content (0.417GWC/0.492VWC). The correlation between dry density and maximum gravimetric water content is much stronger than the trend shown for volumetric water content. As discussed in Sect. 1, volumetric water content as a measure does not convey specimen volume change. For example, the 10 % compost specimen has a low volumetric water content compared with other 10 % amendments, but this is because the soil has increased in volume and decreased in dry density, and therefore the ratio of volume of soil to volume of water has reduced.
Further investigation is needed to characterize volume change characteristics; particularly testing over more climatic cycles to reach equilibrium is required to evaluate the true effect of amendments on the swelling and shrinkage properties of the soil. Amended samples undergo a large degree of expansion when wetted, but the shrinkage (data not presented) is greatly reduced compared with the control soil, indicating that the structure-amended specimens are more stable than the control soil. This is important for the application of amendments in areas where large volume change is undesirable but less so for flood resilience. The relationship between the parameters of dry density, water content, and volume change for specimens with the addition of highly compressible material (compost) needs greater exploration, as the preparation conditions, i.e. the use of dry density as a control, clearly have a fundamental impact on the performance of the material in terms of the water retention and volume change.
3.3 Hydraulic conductivity
Figure 4 shows the variation in saturated hydraulic conductivity (ksat) at three different confining stress levels (25, 50, and 100 kPa), using a pressure difference of 15 kPa to generate flow. In general the ksat of specimens is lower at greater confining pressure, as increasing the confining stress causes a reduction in porosity, making water flow more difficult. Single amendments of WTR increase the ksat to the greatest extent (5.7–26.3-fold increase). It is clear that at 25 kPa all amendments improve the ksat compared with soil alone, with the exception of 30 % compost, as shown in Fig. 4. Compost amendments provide little improvement in ksat at 25 kPa, and at higher cell pressure, the presence of organic matter causes a reduction in ksat. This behaviour is typical of any organic containing soils; however in this case any soil type which is 30 % compost is, per volume, mostly compost with disconnected mineral matter, and so the compost dominates the soil. The reduction in permeability of the highly organic amendment under load is similar to the characteristics shown in peat soils (with conductivity around 10−9). We know that compost has a high water retention, and despite organic amended specimens having the highest water content at saturation, we can assume that the permeability is lowest in this case because the pathways through organic matter are much more tortuous than granular soil, despite the large pore space provided by the organic matter. The ability of the co-amendments to improve ksat is dependent on the amendment ratio, where higher amendment proportions show the greatest improvement. The control soil has a ksat of m s−1 at 25 kPa, which is classed as moderate but typical of sand, silt, and clay mixtures. WTR-amended soils have very high ksat in comparison with the control soil at 25 kPa; 30 %WTRd has the highest ksat ( m s−1), which is typical of clean sands, followed by 30 % WTRw ( m s−1), 20 % WTRw ( m s−1), 10 % WTRw ( m s−1), 30 % coWTRw ( m s−1), and 20 % WTRd ( m s−1). The value obtained for 10 % WTRd was m s−1, indicating that this result may be an error. The ksat of WTR-amended specimens is therefore 5.7 to 26.3 times greater than the control soil (570 %–2600 % improvement). WTRd amendment contributes irregularly shaped particles with a broad range of particle size to the control soil, whereas WTRw amendment adds a lower proportion of small irregularly shaped particles and a large proportion of very fine material (<75 µm). Both of these additions improve the soil structure, reduce the bulk density of the amended specimen, and may increase pore space as a result of irregular particle shape. As one WTR type does not consistently result in an improved conductivity over the other, this difference may also be due to the heterogeneity of specimens rather than a fundamental difference in material properties. These results indicate that the addition of WTRw and WTRd has a significant impact on the ksat of the soil, and compost addition dampens this effect. The high ksat of amended soils indicates that a soil would be able to transmit water through the soil profile rapidly and avoid water pooling at the surface during high-intensity events, which is particularly important for reducing erosion at the soil surface.
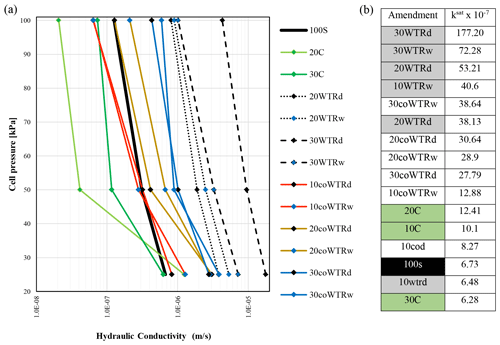
Figure 4(a) Saturated hydraulic conductivity of samples conducted in a triaxial cell at pressures of 25, 50, and 100 kPa, on a log scale. Samples 10C, 10WTRd, and 10WTRw were only tested at 25 kPa and do not feature on the graph; their k values were , , and m s−1 respectively. n=1 for all samples. (b) Ranked conductivity values for all specimens at 25 kPa.
3.4 Shear strength
Amended soil specimens were assessed for their strength in confined undrained triaxial testing to determine any changes in strength due to the addition of WTR or a WTR and compost co-amendment whilst in a saturated state. This was an important measurement to understand how well a soil will cope with shear stresses when flooded. It is clear from Fig. 5a that at low confining pressure (25 kPa) the amendments with WTR alone (30WTR) show the greatest improvement compared with the control soil. Soils amended with 30 % compost (30C) and co-amendments with 15 % compost and 15 % WTR (30coWTR) show an improvement in strength compared with the unamended soil (100S) when tested at 25 kPa. It can be seen from the stress paths in Fig. 5b that the paths for the amended soils shift to the left compared with the un-amended soil (100S), indicating more pore water pressure development. This might be expected for the compost amendments, due to the more compressible nature of the compost. It is interesting to see this is also true for the WTR alone. It seems that the amendment with the wet WTR (30WTRw) shows the greatest improvement (Fig. 5a), but the sharp kink in the stress path in Fig. 5b might suggest that the test using dry WTR (30WTRd) developed a localized shear surface during testing that might have restricted its ability to gain in strength. Therefore, the difference between 30WTRw and 30WTRd could be due to heterogeneity rather than a fundamental difference in behaviour.
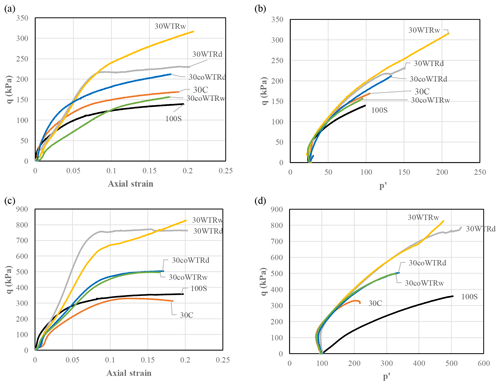
Figure 5Panels (a) and (b) show the stress–strain response and stress paths for undrained triaxial tests carried out for a confining stress of 25 kPa, and panels (c) and (d) show the same information for tests carried out for a confining stress of 100 kPa.
At the higher stress level of 100 kPa (Fig. 5c), the amendment of compost alone (30C) shows no improvement compared with the un-amended soil. However, the co-amendments with 15 % compost and 15 % WTR (30coWTR) do show significant improvements in strength. Again, the greatest improvements are for the WTR alone (30WTR). There is no major difference whether the WTR is wet or dry. Again, the stress paths in Fig. 5d show that all the amended soils develop much greater pore water pressure than the un-amended soil (100S), indicating a more compressible response. This increase in strength with the addition of WTR may be due to physical changes in soil structure owing to the irregular shape of WTR particles or through chemical contributions of the flocculant and coagulant aids that make up WTR. Further investigation is needed to understand the strength mechanics of amended soil, particularly after specimens have been subjected to climatic cycles.
3.5 Soil health and flood-holding capacity
The previous sections have discussed in detail the effect of different amendments to individual physical soil parameters, but a whole-picture assessment must be made to determine to what extent these changes are beneficial to the overall soil health and for particular events such as flooding. To assess the effect on soil health, we must establish what determines a beneficial change. If considering the soil health with respect to events such as flooding, the increase in bulk volume and reduction in density of soil upon wetting is of critical importance as it determines the extent to which soil can accommodate water. In addition, the soil must be able to transmit this water quickly through the profile to reduce phenomena such as runoff and slaking at the soil surface, both of which block downward flow of water into the soil profile. Finally, the soil must retain structural stability while in a highly wetted state. Thus a successful amendment to soil in this respect is one that has a high capacity to hold and transmit water, allows swelling with limited shrinkage during drying phases, and retains strength when saturated. Although compost amendments increase the saturated water content and reduce bulk density of the soil to which they are applied, this amendment does not give the soil the ability to effectively transmit water in WTR, nor does it improve the shear strength and thus structural stability of the soil. In contrast, WTR amendments only have marginal effects on the water retention properties but provide a vastly improved hydraulic conductivity and shear strength in comparison with both the control soil and compost amended soils. In this instance, the co-amendment of WTR and compost provides the best compromise between these physical parameters, where compost addition provides beneficial water retention and low bulk density characteristics, and WTR provides key properties of conductivity and strength.
Although we have assessed the changes in soil health based on its response to flooding, this research shows that highly WTR-amended soil would be well suited to applications where shear strength and permeability are key features, such as retaining structures or non-vegetated areas such as groundwork near structures or as a subsurface to urbanized areas.
The amendment of a sandy loam soil with either Fe WTR or with Fe WTR and compost co-amendment has significant impacts on a soil's flood-holding capacity, which by definition incorporates the parameters of water retention, hydraulic conductivity, volume change, and shear strength. This view incorporating each critical physical parameter of how an amendment affects the properties of a soil is essential if the amendment is going to be used effectively to improve critical services of the soil. The following generalizations can be made on the changes in physical soil parameters: the single addition of WTR results in an increase in water content and reduction in dry density at saturation and increases the saturated hydraulic conductivity and shear strength of the soil compared with the control. The use of compost as a single amendment yielded the best improvements in water retention properties; however the negative effects of this amendment on saturated hydraulic conductivity and shear strength mean that it does not impart the properties required for a flood-resistant soil. However, the addition of compost and WTR as a co-amendment combines the beneficial properties of both materials, resulting in higher water content at saturation compared with both the control soil and WTR alone, whilst also increasing saturated conductivity, increasing the volume change (reduction in dry density) and the shear strength of soil (albeit to a lesser extent than WTR amendment). As such, the co-amendment offers an advantage over the use of single amendments of WTR or compost as each have their own drawbacks of application (concerns on PTEs and P immobilization of WTR and lack of shear strength and hydraulic conductivity of compost). Data presented suggest that recycling WTRs can provide a route with which to address critical Sustainable Development Goals (SDGs) to rebuild and regenerate soils whilst encouraging a circular economy. Finding an optimal amendment balance for amending soil means that with increasing clear water production, water treatment companies are able to fulfil their requirement to recycle waste while benefitting degraded soils. Further research is needed to establish the optimal amendment ratios to provide the most beneficial improvement to physical soil parameters for ecosystem functions, particularly the flood-holding capacity of an amended soil. This includes monitoring the water retention and volume change of specimens over numerous climatic cycles, an assessment of unsaturated hydraulic conductivity, and an assessment of shear strength of amended soils after several climatic cycles. An understanding of the soil water retention curve would aid in further discussion of these changes and allow us to fully understand WTR's effect.
Full data sets are available from FigShare (https://doi.org/10.6084/m9.figshare.16441416.v1, Kerr, 2021).
Research was conceptualized and conducted by HCK under the supervision of KLJ as part of an EPSRC-funded PhD. DGT was a secondary supervisor and aided in investigation of triaxial testing, concept and review of data, and writing for publication.
The contact author has declared that neither they nor their co-authors have any competing interests.
Publisher’s note: Copernicus Publications remains neutral with regard to jurisdictional claims in published maps and institutional affiliations.
Data in this publication were drawn from research funded by the EPSRC as part of a PhD project at the University of Durham. Thanks are expressed to all those that have contributed during Heather Kerr's PhD and in writing this report.
This research has been supported by the EPSRC as part of a PhD project at the University of Durham.
This paper was edited by Jose Alfonso Gomez and reviewed by two anonymous referees.
Agyin-Birikorang, S., O'connor, G. A., Jacobs, L. W., Makris, K. C., and Brinton, S. R.: Long-term phosphorus immobilization by a drinking water treatment residual, J. Environ. Qual., 36, 316–323, 2007.
Babatunde, A. O., Zhao, Y. Q., Burke, A. M., Morris, M. A., and Hanrahan, J. P.: Characterization of aluminium-based water treatment residual for potential phosphorus removal in engineered wetlands, Environ. Pollut., 157, 2830–2836, https://doi.org/10.1016/j.envpol.2009.04.016, 2009.
Basim, S. C.: Physical and geotechnical characterization of water treatment plant residuals, Dissertation, New Jersey Institute of Technology, 977, https://digitalcommons.njit.edu/dissertations/977 (last access: 25 August 2021), 1999.
Basta, N. T.: Examples and case studies of beneficial re-use of municipal by-products, Chap. 17, in: Land application of agricultural, industrial, and municipal by-products, Book Series No. 6, edited by: Power, J. F. and Dick, W. A., Soil Science Society of America, Madison, WI, https://doi.org/10.2136/sssabookser6.c17, 2000.
Boivin, P., Schäffer, B., and Sturny, W.: Quantifying the relationship between soil organic carbon and soil physical properties using shrinkage modelling, Eur. J. Soil Sci., 60, 265–275, https://doi.org/10.1111/j.1365-2389.2008.01107.x, 2009.
Chiang, Y. W., Ghyselbrecht, K., Santos, R. M., Martens, J. A., Swennen, R., Cappuyns, V., and Meesschaert, B.: Adsorption of multi-heavy metals onto water treatment residuals: sorption capacities and applications, Chem. Eng. J., 200, 405–415, https://doi.org/10.1016/j.cej.2012.06.070, 2012.
Cotruvo, J. A.: 2017 WHO Guidelines for drinking-water quality: first addendum to the fourth edition, J. Am. Water Works Ass., 109, 44–51, https://doi.org/10.5942/jawwa.2017.109.0087, 2017.
Dassanayake, K. B., Jayasinghe, G. Y., Surapaneni, A., and Hetherington, C.: A review on alum sludge re-use with special reference to agricultural applications and future challenges, Waste Manage., 38, 321–335, 2015.
Dayton, E. A. and Basta, N. T.: Characterization of drinking water treatment residuals for use as a soil substitute, Water Environ. Res., 73, 52–57, https://doi.org/10.2175/106143001X138688, 2001.
DETS: Soil analysis, https://www.suez.co.uk/en-gb/our-offering/businesses/what-are-you-looking-for/laboratory-and-technical-services/soil-analysis, last access: 13 February 2018.
Elliott, H. A. and Dempsey, B. A.: Agronomic effects of land application of water treatment sludges, J.Am. Water Works Ass., 83, 126–131, https://doi.org/10.1002/j.1551-8833.1991.tb07129.x, 1991.
El-Swaify, S. A. and Emerson, W. W.: Changes in the physical properties of soil clays due to precipitated aluminium and iron hydroxides: I. Swelling and aggregate stability after drying, Soil Sci. Soc. Am. J., 39, 1056–1063, https://doi.org/10.2136/sssaj1975.03615995003900060016x, 1975.
Finlay, N. C.: Using Water Treatment Residual to immobilise lead for in-situ remediation of contaminated soil, Durham theses, Durham University, http://etheses.dur.ac.uk/11887/ (last access: 27 July 2021), 2015.
Gomes, S. D. C., Zhou, J. L., Li, W., and Long, G.: Progress in manufacture and properties of construction materials incorporating water treatment sludge: A review, Resour. Conserv. Recy., 145, 148–159, 2019.
Hazbavi, Z. and Sadeghi, S. H. R.: Potential effects of vinasse as a soil amendment to control runoff and soil loss, SOIL, 2, 71–78, https://doi.org/10.5194/soil-2-71-2016, 2016.
Hegazy, B. E., Hanan, A. F., and Ahmed, M. H.: Brick Manufacturing from Water Treatment Sludge and Rise Husk Ash, Aust. J. Bas. Appl. Sci., 6, 453–461, 2012.
Huang, C. H. and Wang, S. Y.: Application of water treatment sludge in the manufacturing of lightweight aggregate, Constr. Build. Mater., 43, 174–183, https://doi.org/10.1016/j.conbuildmat.2013.02.016, 2013.
Ippolito, J. A., Barbarack, K. A., Stromberger, M. E., Paschke, M. W., and Brobst, R. B.: Water treatment residuals and biosolids long-term co-applications effects to semi-arid grassland soils and vegetation, Soil Sci. Soc. Am. J., 73, 1880–1889, https://doi.org/10.2136/sssaj2008.0352, 2009.
Ippolito, J. A., Barbarick, K. A., and Elliott, H. A.: Drinking water treatment residuals: a review of recent uses, J. Environ. Qual., 40, 1–12, https://doi.org/10.2134/jeq2010.0242, 2011.
Kay, B. D.: Soil structure and organic carbon: A review, in: Soil processes and the carbon cycle, edited by: Lal, R., Kimble, J. M., Follett, R. F., and Stewart, B. A., CRC press, Boca Raton, 169–1971, https://doi.org/10.1201/9780203739273, 1998.
Keeley, J., Jarvis, P., and Judd, S. J.: Coagulant recovery from water treatment residuals: a review of applicable technologies, Crit. Rev. Environ. Sci. Technol., 44, 2675–2719, 2014.
Kerr, H.: Using a water treatment residual and compost co-amendment as a sustainable soil improvement technology to enhance flood holding capacity, PhD thesis, Durham University, http://etheses.dur.ac.uk/12983/ (last access: 25 August 2021), 2019.
Kerr, H.: Summary Data FHC, figshare [data set], https://doi.org/10.6084/m9.figshare.16441416.v1, 2021.
Kerr, H., Johnson, K., Toll, D. G., and Mansfield, F.: Flood holding capacity: a novel concept to evaluate the resilience of amended soils, Proceedings of Geo-Chicago, 14–18 August 2016, Chicago, Illinois, 393–409, https://doi.org/10.1061/9780784480120.041, 2016.
Krause, A., Nehls, T., George, E., and Kaupenjohann, M.: Organic wastes from bioenergy and ecological sanitation as a soil fertility improver: a field experiment in a tropical Andosol, SOIL, 2, 147–162, https://doi.org/10.5194/soil-2-147-2016, 2016.
Kyncl, M.: Opportunities for water treatment sludge re-use, Geosci. Eng., 1, 11–22, 2008.
Lal, R.: Soil health and carbon management, Food and Energy Security, 5, 212–222, https://doi.org/10.1002/fes3.96, 2016.
Lalonde, K., Mucci, A., Ouellet, A., and Gélinas, Y.: Preservation of organic matter in sediments promoted by iron, Nature, 483, 198–200, 2012.
Li, J., Liu, L., Liu, J., Ma, T., Yan, A., and Ni, Y.: Effect of adding alum sludge from water treatment plant on sewage sludge dewatering, J. Environ. Chem. Eng., 4, 746–752, https://doi.org/10.1016/j.jece.2015.07.021, 2016.
Liu, Y., Zhuge, Y., Chow, C. W., Keegan, A., Li, D., Pham, P. N., Huang, J. and Siddique, R.: Utilization of drinking water treatment sludge in concrete paving blocks: Microstructural analysis, durability and leaching properties, J. Environ. Manage., 262, 110352, https://doi.org/10.1016/j.jenvman.2020.110352, 2020.
Mokonyama, S., Schalkwyk, M., and Rajagopaul, R.: Guidelines and Good Practices for Water Treatment Residues Handling, Disposal and Reuse in South Africa, Water Research Commission, Gezina South Africa, 140, Report No. TT738/17, ISBN 978-1-4312-0937-8, http://www.wrc.org.za/wp-content/uploads/mdocs/TT738.pdf (last access: 25 August 2021), 2017.
Mahdy, A. M., Elkhatib, E. A., Fathi, N. O., and Lin, Z. Q.: Effects of co-application of biosolids and water treatment residuals on corn growth and bioavailable phosphorus and aluminium in alkaline soils in Egypt, J. Environ. Qual., 38, 1501–1510, https://doi.org/10.2134/jeq2008.0335, 2009.
Makris, K. C., Harris, W. G., O'Connor, G. A., Obreza, T. A., and Elliott, H. A.: Physicochemical properties related to long-term phosphorus retention by drinking-water treatment residuals, Environ. Sci. Technol., 39, 4280–4289, https://doi.org/10.1021/es0480769, 2005.
Moodley, M., Johnston, M. A., Hughes, J. C., and Titshall, L. W.: Effects of a water treatment residue, lime, gypsum, and polyacrylamide on the water retention and hydraulic conductivity of two contrasting soils under field conditions in KwaZulu-Natal, South Africa, Soil Res., 42, 273–282, https://doi.org/10.1071/SR03045, 2004.
Mukherjee, A., Lal, R., and Zimmerman, A. R.: Effects of biochar and other amendments on the physical properties and greenhouse gas emissions of an artificially degraded soil, Sci. Total Environ., 487, 26–36, 2014.
Nagar, R., Sarkar, D., Makris, K. C., Datta, R., and Sylvia, V. L.: Bioavailability and bioaccessibility of arsenic in a soil amended with drinking-water treatment residuals, Arch. Environ. Con. Tox., 57, 755–766, https://doi.org/10.1007/s00244-009-9318-7, 2009.
Neal, A. L., Bacq-Labreuil, A., Zhang, X., Clark, I. M., Coleman, K., Mooney, S. J., Ritz, K., and Crawford, J. W.: Soil as an extended composite phenotype of the microbial metagenome, Sci. Rep.-UK, 10, 1–16, 2020.
Odimegwu, T. C., Zakaria, I., Abood, M. M., Nketsiah, C. B. K., and Ahmad, M.: Review on different beneficial ways of applying alum sludge in a sustainable disposal manner, Civ. Eng. J., 4, 2230–2241, https://doi.org/10.28991/cej-03091153, 2018.
O'Kelly, B. C.: Biodegradation of biosolids and specific gravity determination, Environ. Geotech., 6, 47–61, https://doi.org/10.1680/jenge.16.00014, 2016.
Oladeji, O. O., O'Connor, G. A., Sartain, J. B., and Nair, V. D.: Controlled application rate of water treatment residual for agronomic and environmental benefits, J. Environ. Qual., 36, 1715–1724, https://doi.org/10.2134/jeq2007.0160, 2007.
Oladeji, O. O., O'Connor, G. A., and Brinton, S. R.: Surface applied water treatment residuals affect bioavailable phosphorus losses in Florida sands, J. Environ. Manage., 88, 1593–1600, https://doi.org/10.1016/j.jenvman.2007.08.001, 2008.
Oliver, I. W., Grant, C. D., and Murray, R. S.: Assessing effects of aerobic and anaerobic conditions on phosphorus sorption and retention capacity of water treatment residuals, J. Environ. Manage., 92, 960–966, https://doi.org/10.1016/j.jenvman.2010.11.016, 2011.
Rhodes, C. J.: The 2015 Paris climate change conference: COP21, Sci. Prog., 99, 97–104, https://doi.org/10.3184/003685016X14528569315192, 2016.
Peng, X., Horn, R., and Smucker, A.: Pore shrinkage dependency of inorganic and organic soils on wetting and drying cycles, Soil Sci. Soc. Am. J., 71, 1095–1104, https://doi.org/10.2136/sssaj2006.0156, 2007.
Peng, X. and Horn, R.: Anisotropic shrinkage and swelling of some organic and inorganic soils, Eur. J. Soil Sci., 58, 98–107, https://doi.org/10.1111/j.1365-2389.2006.00808.x, 2007.
Peng, X., Zhang, Z. B., Wang, L. L., and Gan, L.: Does soil compaction change soil shrinkage behaviour?, Soil Till. Res., 125, 89–95, https://doi.org/10.1016/j.still.2012.04.001, 2012.
Rahman, M. T., Zhu, Q. H., Zhang, Z. B., Zhou, H., and Peng, X.: The roles of organic amendments and microbial community in the improvement of soil structure of a Vertisol, Appl. Soil Ecol., 111, 84–93, https://doi.org/10.1016/j.apsoil.2016.11.018, 2017.
Rengasamy, P., Oades, J. M., and Hancock, T. W.: Improvement of soil structure and plant growth by addition of alum sludge, Commun. Soil Sci. Plan., 11, 533–545, https://doi.org/10.1080/00103628009367061, 1980.
Rodrigues, L. P. and Holanda, J. N. F.: Recycling of water treatment plant waste for production of soil-cement bricks, Proc. Mat. Sci., 8, 197–202, https://doi.org/10.1016/j.mspro.2015.04.064, 2015.
Shen, C., Zhao, Y., Li, W., Yang, Y., Liu, R., and Morgen, D.: Global profile of heavy metals and semimetals adsorption using drinking water treatment residual, Chem. Eng. J., 372, 1019–1027, https://doi.org/10.1016/j.cej.2019.04.219, 2019.
Sollins, P. and Gregg, J. W.: Soil organic matter accumulation in relation to changing soil volume, mass, and structure: Concepts and calculations, Geoderma, 301, 60–71, https://doi.org/10.1016/j.geoderma.2017.04.013, 2017.
Titshall, L. W. and Hughes, J. C.: Characterisation of some South African water treatment residues and implications for land application, Water Research Commission, South Africa, 31, 299–308, https://doi.org/10.4314/wsa.v31i3.5219, 2005.
Tripathy, S., Rao, K. S., and Fredlund, D. G.: Water content-void ratio swell-shrink paths of compacted expansive soils, Can. Geotech. J., 39, 938–959, https://doi.org/10.1139/t02-022, 2002.
Tipping, E. and Rowe, E. C.: Modelling the physical states, element stoichiometries and residence times of topsoil organic matter, Eur. J. Soil Sci., 70, 321–337, https://doi.org/10.1111/ejss.12785, 2019.
Turner, T., Wheeler, R., Stone, A., and Oliver, I.: Potential alternative reuse pathways for water treatment residuals: Remaining barriers and questions – A review, Water Air Soil Poll., 230, 1–30, https://doi.org/10.1007/s11270-019-4272-0, 2019.
UNESCO: The United Nations World Water Development Report 2019: Leaving No One Behind, UNESCO World Water Assessment Programme, Paris, France, Vol. 96, ISBN 978-9-2310-030-97, 2019.
Xia, Z.: Geotechnical characterisation of water treatment plant residuals, Thesis 1230 submitted to the Faculty of Civil and Environmental Engineering, New Jersey Institute of Technology, Newark, NJ, https://digitalcommons.njit.edu/theses/1230 (last access: 18 August 2021), 1994.
Weindorf, D. C. and Wittie, R.: Determining particle density in dairy manure compost, Tex. J. Agriculture and Natural Resources, 16, 60–63, 2003.
Zhao, Y., Liu, R., Awe, O. W., Yang, Y., and Shen, C.: Acceptability of land application of alum-based water treatment residuals – an explicit and comprehensive review, Chem. Eng. J., 353, 717–726, https://doi.org/10.1016/j.cej.2018.07.143, 2018.
Zhou, H., Fang, H., Zhang, Q., Wang, Q., Chen, C., Mooney, S. J., Peng, X., and Du, Z.: Biochar enhances soil hydraulic function but not soil aggregation in a sandy loam, Eur. J. Soil Sci., 70, 291–300, https://doi.org/10.1111/ejss.12732, 2019.
Zhou, H., Chen, C., Wang, D., Arthur, E., Zhang, Z., Guo, Z., Peng, X., and Mooney, S. J.: Effect of long-term organic amendments on the full-range soil water retention characteristics of a Vertisol, Soil Till. Res., 202, 104663, https://doi.org/10.1016/j.still.2020.104663, 2020.